Abstract
Invasive alien predators are a serious threat to biodiversity worldwide. However, there is no generic method for assessing which local species are most at risk following the invasion of a new predator. The harlequin ladybird, Harmonia axyridis (Pallas) (Coleoptera: Coccinellidae), is an alien in Europe and many other parts of the world where it affects other species of ladybirds through competition for food and intra-guild predation (IGP). Here, we describe a method developed to assess which European ladybird species are most at risk following the invasion of H. axyridis. The three components of the risk assessment are: the likelihood that the assessed native species encounters H. axyridis in the field, the hazard of competition for food, and the IGP hazard. Thirty native European ladybird species were assessed through data obtained from field observations, laboratory experiments and literature reviews. The species that are considered most at risk are found on deciduous trees, have immature stages which are highly vulnerable to IGP by H. axyridis, and are primarily aphidophagous. These species should be the focus of specific studies and possibly conservation actions. The risk assessment method proposed here could be applied to other alien predators which are considered a threat to native species through competition and predation.
Similar content being viewed by others
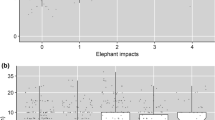
Avoid common mistakes on your manuscript.
Introduction
Invasive alien species are recognised as one of the main causes of animal biodiversity loss (Bellard et al. 2016). Associated animal species declines are mainly due to competitive exclusion, predation, and introduction of new diseases (Long 2003; Kenis et al. 2009). In particular, alien predatory insects are known to displace insects and other animals worldwide through competition for resources and predation (Snyder and Evans 2006; Wagner and van Driesche 2010). In most cases, invasive alien insects have been introduced accidentally, either as contaminants or stowaways (Hulme et al. 2008). Nevertheless, predators and parasitoids have also been released intentionally in biological control programmes for pest control and a small proportion of them have had undesirable ecological effects on non-target species through competition for resources, predation or parasitism on native species (Hajek et al. 2016). Pre-release risk assessment protocols have the potential to limit the risk of non-target effects but these risk assessments are not yet carried out systematically in all countries (van Lenteren et al. 2006; Hajek et al. 2016). Other risk and impact assessment protocols exist for alien species beyond biological control introductions, many of which are appropriate for assessing predators (e.g. D’hondt et al. 2015; Kumschick et al. 2015) and some protocols have been developed for specific groups of predators, such as ants (Harris et al. 2005). However, these protocols aim at assessing the risk or impact of specific invasive alien species, taking into account their general impact in the invaded habitat (Kumschick and Richardson 2013). In contrast, there is no generic method to assess which particular native species are most at risk following the invasion of a predator. Such knowledge is usually lacking despite its obvious potential use for prioritising research and conservation actions in the invaded regions.
An example of an introduced arthropod predator that has had undesirable ecological impacts is the harlequin ladybird Harmonia axyridis (Pallas) (Coleoptera: Coccinellidae). This beetle was introduced into North America in 1916 and throughout the 20th century to control aphids and coccids, and became established in the 1980s (Roy et al. 2016). Since then, although successfully regulating pest aphids in a range of crop systems, it has spread and increased in number rapidly across most of North America, where it has become the dominant aphidophagous ladybird (e.g. Colunga-Garcia and Gage 1998; Harmon et al. 2007). Harmonia axyridis was commercially available in Europe as a pest-control agent from the late 1980s and feral populations were first observed in Germany in 1999 (Brown et al. 2011). The beetle then rapidly invaded most European countries and many other regions on all continents except Antarctica (Roy et al. 2016).
The threat posed by H. axyridis to biodiversity and ecosystem functions in invaded regions may be considerable. Impacts on native species can occur both through direct predation and competition for resources. Harmonia axyridis has a broad diet, putting many non-target species at risk from predation. Whilst preferring aphid and coccid prey, H. axyridis will also accept the immature stages of many insects, including butterflies and other aphid predators (Koch et al. 2003, 2006; Pell et al. 2008). Intra-guild predation (IGP), i.e. the killing and eating of species eating similar, often limiting, resources (Polis et al. 1989), is widespread amongst aphidophagous insects, and H. axyridis is known to attack the immature stages of many ladybird guild members (Ware and Majerus 2008; Ware et al. 2008a; Rondoni et al. 2012a, b; Katsanis et al. 2013). Recent observations have demonstrated that H. axyridis also feeds on European ladybirds in the field (Hautier et al. 2011; Thomas et al. 2013; Rondoni et al. 2014; Brown et al. 2015).
Negative effects of H. axyridis on native Coccinellidae have already been documented in North America. For example, H. axyridis was reported to be displacing Cycloneda sanguinea (L.), in Florida citrus orchards (Michaud 2002). Similarly, within four years of its arrival in Michigan, H. axyridis had taken over the status of Coleomegilla maculata (DeGeer) as the dominant aphid predator, and a decrease in populations of three other species of ladybird was reported: Brachiacantha ursina (F.), Cycloneda munda (Say) and Chilocorus stigma (Say) (Colunga-Garcia and Gage 1998). However, in an analysis of a 26-years dataset in the same region, Bahlai et al. (2015) confirmed the long term decline of two species only, C. maculata and Adalia bipunctata (L.).
In Europe, field studies have been undertaken in several countries to monitor changes in populations of native ladybird species following the arrival of H. axyridis (e.g. Eschen et al. 2007; Adriaens et al. 2008; Brown et al. 2011). A first assessment of the impact of H. axyridis on native species of ladybird in Belgium, Britain and Switzerland showed that some historically widespread and common species are declining (Roy et al. 2012). For A. bipunctata in Belgium, the rate of decline warrants red listing as a vulnerable species (Adriaens et al. 2015). However, ladybird populations are known to fluctuate naturally and, thus, it may take several years before a long-term impact can be ascertained. Also, some species were already in decline before the arrival of H. axyridis, suggesting that the invasion acted as an additional stressor on insect populations on top of other factors (Roy et al. 2012). Furthermore, general faunistic studies are able to monitor changes in the most abundant species but less common species are only rarely captured. It is essential that research is rapidly centred on those species that are most at risk and so we carried out a qualitative risk assessment for native ladybird species in Europe to determine those species that are most and least likely to decline following the invasion of H. axyridis. The risk was assessed for most “true ladybirds” (sensu Majerus 1994, i.e. sub-families Coccinellinae, Epilachninae and Chilocorinae) occurring in Western Europe.
This study also provides us with the opportunity to develop rapid and relevant methods of qualitative risk assessment for established alien predators. In particular, we hope that our approach may be usefully applied to other arthropod predators that are in the process of invasion and for which conclusive impacts on biodiversity are not yet observed or measurable. Also, for already established alien predators the results of such assessments can inform risk management and prioritisation in uninvaded regions.
Materials and methods
A risk assessment involves identifying and quantifying the hazards, and determining the probabilities that these hazards will materialise. The risk is then the product of a likelihood (probability) and a magnitude of hazard (consequence). In this study, the risk posed by H. axyridis on 30 native ladybirds (see list in Table 1) was considered as the product of the likelihood that a given ladybird species encounters H. axyridis in the field, and the consequence of this encounter, through competition for food and direct predation. Therefore, the risk assessment was carried out in three steps: (1) likelihood of encounter with H. axyridis in the field, (2) competition for food, (3) intra-guild predation.
Likelihood of encounter with H. axyridis in the field
The likelihood that a native ladybird species encounters H. axyridis in the field was measured using field data in Switzerland, Belgium and Britain. Datasets comprising field observations were used to assess spatial and temporal co-occurrence between native species and H. axyridis. Since the data in the three countries had not been collected for this particular purpose, sampling and, subsequently, calculation methods differed among countries.
In Switzerland, extensive surveys for ladybirds carried out from 2006 to 2009 in the North-Western part of the country (Cantons Jura, Basel-Landschaft, Basel-Stadt and Solothurn) were used to calculate an ‘asymmetric habitat overlap index’, defined as ‘the proportion of specimens of a given species collected at the same time and the same site as H. axyridis’. The habitat overlap is qualified as “asymmetric” because no ladybird has exactly the same habitat as another and, thus, the proportion of specimens of a species X collected with H. axyridis will most likely be different from the proportion of H. axyridis collected with species X. Surveys (and site locations) were carried out as described in Eschen et al. (2007) and Zindel (2008). Branches of conifers, broadleaved trees and shrubs were beaten over a tray to collect adult ladybirds. Meadows and grasslands were sampled using a sweep net. Insects collected at the same time on the same group of trees (maximum 50 m distance), the same hedgerow (maximum 50 m long) or the same meadow were considered to co-occur. We used data exclusively obtained from sites where H. axyridis was thought to be established, i.e. where either few specimens had been found in the previous year, or high numbers of specimens had been found in the year of observation, and data were collected at the same site for a maximum of two years. Using data from areas where H. axyridis was not yet established could have resulted in false negative co-occurrence while using data from sites where H. axyridis had been abundant for more than two years would have the risk that the displacement of native species had already occurred. For 16 native ladybird species, for which at least 20 specimens were collected, the asymmetric habitat overlap index was measured. A total of 2956 records of adult specimens were included in the analyses.
In Belgium and Britain extensive national surveys were carried out in various habitats providing occurrence data for a larger number of species (adults and, to a lesser extent, larvae) but at lower resolution than in Switzerland (Adriaens et al. 2008; Brown et al. 2011; Roy et al. 2012). In Belgium, an index named “spatio-temporal co-occurrence with H. axyridis” was calculated by Adriaens et al. (2008), based on occurrence data from 2004-2006 extracted from the large dataset of the Belgian ladybird working group (Adriaens et al. 2012). It was defined as the number of collection events (for which the following information was available: species observed, number of individuals, development stage, observation date, observer and location) where a given species had been found with H. axyridis, at the same date in a 1-km2 grid cell, divided by the total number of collection events with this species and any other ladybird species, including H. axyridis (n = 2699 collection events and over 10,000 individuals). This index was calculated for all native ladybirds selected for the assessment. All species had over 20 records in the database.
The British data were analysed similarly to the Belgian data. Ladybird species spatio-temporal co-occurrence data (i.e. where two or more species were recorded at a site on the same date, whether or not one of them was H. axyridis) from 2004–2008 were analysed (n = 3069 collection events comprising 8755 species records). Only species with over 20 records were included. The records were mostly from specific site surveys, rather than general 1-km2 surveys: 66.2 % of the surveys in which H. axyridis was one of the species were recorded at 100 m square resolution or higher. In order to reduce the potential bias caused by a small number of sites being surveyed many times on different dates, a grid reference was excluded from the dataset if the grid reference/date combination had more than 40 records. The British spatio-temporal index was calculated by dividing the number of records where H. axyridis was recorded with a certain species by the total number of records for that species with any other ladybird, including H. axyridis.
The three national co-occurrence indices were then used to assess the likelihood of encounter in three-point scales. Since sampling and calculation methods differed in the three countries, the range of the indices differed. Therefore, for each country, the index range (i.e. the range between the minimum and the maximum indexes) was divided into three sections of equal length and scored as follows: for species with index values in the highest section, the likelihood of encounter was considered very likely (score 3), for those in the mid section, moderately likely (score 2) and for those in the lowest section, unlikely (score 1):
-
3.
Encounter very likely: Swiss data: above 46.4 %, Belgian data: above 57.0 %, British data: above 13.9 %
-
2.
Encounter moderately likely: Swiss data: 23.3–46.4 % in, Belgian data: 38.7–57.0 %, British data: 8.7–13.9 %
-
1.
Encounter unlikely: Swiss data: less than 23.3 %, Belgian data: less than 38.7 %, British data: less than 8.7 %
For each species, a final score of likelihood of encounter was given, corresponding to the average of the country scores, rounded to the nearest unit. Where only two countries provided a score for a particular species and these scores differed by one unit, the highest score was selected.
Competition for food
A three-point scale was adopted to assess the exposure to competition for food, progressing from minimal hazard (1) to major hazard (3). The exact degree of competition for food at the field-scale is extremely difficult to assess. Nevertheless, the most obvious determinant of whether competition for food represents a problem is whether the diet utilised by a given species is also utilised by H. axyridis. Harmonia axyridis is recognised as a primarily aphidophagous species, but is able to feed on other insects (Hodek and Evans 2012). Thus, aphidophagous species will be most exposed to competition for food with H. axyridis. Competition for coccids, adelgids or other insects is a possibility but probably less likely than competition for aphids. Finally, species utilising more unusual diets, such as mildew, pollen and plants will be least exposed to competition for food. Data on dietary requirements of European ladybirds was obtained from the literature (e.g. Majerus 1994; Roy et al. 2011; Hodek and Evans 2012) and used to assign each species to one of the following score categories:
-
3.
Major hazard: feeds principally on aphids
-
2.
Moderate hazard: feeds principally on other insects
-
1.
Minor hazard: feeds principally on non-insect food
Intra-guild predation (IGP)
The consequence of IGP between H. axyridis and native species was assessed through experimental data available for 14 out of the 30 native species. Defensive strategies against IGP at a range of life stages were also considered. Methods and results are described in detail in Ware et al. (2008a), Ware and Majerus (2008) and Katsanis et al. (2013). Similar studies from Félix and Soares (2004), Nóia et al. (2008) and Rondoni et al. (2012a, b) were also included in the assessment for two native species not covered by the three previously cited references, i.e. Coccinella undecimpunctata L. and Platynaspis luteorubra Goeze. To summarise, Petri-dish tests were conducted to collect data on: the susceptibility of different coccinellids at the egg stage to predation by first instar H. axyridis larvae, the susceptibility of coccinellids at the pre-pupal and pupal stage to predation by the last (fourth) instar H. axyridis larval stage, and the outcome of confrontation between H. axyridis and the larvae of native species at first or fourth instar larvae. In each case, single larvae were exposed to either single eggs, single larvae, pupae or pre-pupae for a period of 24 or 48 h, without the presence of any other food source. The full range of tests was carried out with A. bipunctata, A. decempunctata (L.), Anatis ocellata (L.), Calvia quatuordecimguttata (L.), Coccinella septempunctata L., Harmonia quadripunctata Pontoppidan, and Propylea quattuordecimpunctata (L.). Egg predation was not tested with Coccinella quinquepunctata L. and predation on pre-pupae and pupae was not tested on Aphidecta obliterata (L.), Calvia decemguttata (L.), Hippodamia variegata (Goeze) and Oenopia conglobata (L.). Data on predation on fourth instar larvae on P. luteorubra were retrieved from Rondoni et al. (2012a, b) whereas Félix and Soares (2004) and Nóia et al. (2008) provided tests on all developmental stages of C. undecimpunctata.
The work described above in effect addressed physical and chemical defence only. In reality, a range of behavioural tactics may be employed to reduce the risk of predation, many of which may be unnoticeable within the confines of a Petri-dish. Certain behavioural strategies may provide effective immediate escape from the risk of IGP by H. axyridis, such as high mobility of larvae compared to H. axyridis and dropping off the plants when a predator approaches (Sato et al. 2005). Such defence strategies, when known from the literature or from unpublished observations, were taken into account in the assessment of the 14 tested species, as described below.
Based on the above, species were assigned to hazard scores as follows:
-
3.
Major hazard: Native species highly susceptible, i.e. asymmetric IGP in favour of H. axyridis observed in at least two life stages, and no asymmetric IGP in favour of the native species observed in any stage, and short-term behavioural avoidance unlikely (i.e. no obvious strategies documented or observed for this species).
-
2.
Moderate hazard: Native species less susceptible, i.e. no asymmetric IGP in favour of the native species observed in any stage but asymmetric IGP in favour of H. axyridis not observed in more than one life stage. Or, if IGP observed in more than one life stage, short-term behavioural avoidance observed and/or described in the literature.
-
1.
Minor hazard: Asymmetric IGP in favour of the native species observed in at least one life stage, i.e. physical or chemical defences present at all or some life stages.
The 18 species that were not tested were given a moderate hazard score (2).
Final risk assessment
A total risk index score was calculated for each of the 30 native species, by multiplying the score of the likelihood of encounter by the sum of the two hazard scores. Ladybirds reaching the maximum risk index of 18 (3 × (3 + 3)) were considered at very high risk, and those with scores of 12–15, 8–10 and 2–6 were considered at high, medium and low risk, respectively.
Results
Likelihood of encounter with H. axyridis in the field
Scores for likelihood of encounter were generally consistent in the three countries, particularly for those species with high scores (Table 1). The species with the highest co-occurrence indices, i.e. those most likely to encounter H. axyridis in the field, were aphidophagous species living principally on broadleaved trees, i.e. Adalia spp., Calvia spp. and O. conglobata. For example, in Switzerland, 69.8 % of the A. bipunctata adults were collected together with at least one H. axyridis. The lowest indices and scores were obtained by species living in grasses, in particular the pollen and fungus feeding Tytthaspis sedecimpunctata (L.) or the phytophagous Henosepilachna argus (Geoffroy) or Subcoccinella vigintiquatuorpunctata (L.) (Table 1). No specimen of these three species was collected with H. axyridis during our surveys in Switzerland. Aphidophagous species associated with conifers, such as A. obliterata and ubiquitous species such as C. septempunctata and P. quattuordecimpunctata scored low, particularly in Switzerland, where only 3.4, 2.3 and 20.5 % of the adults of these three species were collected with H. axyridis, respectively. In Britain and Belgium, H. axyridis co-occurred to a moderate extent with C. septempunctata, especially where nettles (Urtica spp.) were prominent. Also, overall there was a moderately high co-occurrence in Britain and Belgium with conifer specialists such as H. quadripunctata, A. ocellata and Myrrha octodecimguttata (L.). Another conifer species, Myzia oblongoguttata (L.) even scored high in Britain although H. axyridis is not abundant on pine trees in at least parts of England (Brown et al. 2011).
Competition for food
From the 30 species included in the assessment, 19 are principally aphidophagous and were scored 3 to indicate the high potential for competition for food (Table 2). Chilocorinae (Chilocorus spp. and Exochomus spp.) feed mainly on scale insects. Aphidecta obliterata is mainly a predator of Adelgidae and Coccinella hieroglyphica L. feeds mainly on Chrysomelidae. They received a score of 2. Finally, three phytophagous species (Cynegetis impunctata (L.), H. argus and S. vigintiquatuorpunctata) and three mostly mycophagous species (Halyzia sedecimguttata (L.), Psyllobora vigintiduopunctata (L.) and T. sedecimpunctata) were scored 1.
Intra-guild predation (IGP)
Results of IGP tests are described in detail in previous publications (Félix and Soares 2004; Ware and Majerus 2008; Ware et al. 2008a; Rondoni et al. 2012a, b; Katsanis et al. 2013). From the 14 species in which IGP with H. axyridis was assessed, nine were highly vulnerable to IGP by H. axyridis and behavioural avoidance is not known. Thus these received a score of 3 (Table 2). Two of the tested species were scored 1. Eggs of C. quatuordecimguttata were avoided by H. axyridis larvae due to an external chemical deterrent (Ware et al. 2008b; Katsanis et al. 2013). Larvae of C. quatuordecimguttata were also less vulnerable to IGP than the other species tested and showed mostly symmetric IGP with H. axyridis larvae. Anatis ocellata larvae acted as the only asymmetric intra-guild predator of H. axyridis larvae (Katsanis et al. 2013). These results are attributed to differences in physical defensive structures: larvae of H. axyridis, A. ocellata and, to a lesser extent, C. quatuordecimguttata are protected by a covering of thick dorsal and dorsolateral spines, whereas larvae of other species possess fine hairs only, or are sometimes almost smooth (Ware and Majerus 2008; Roy et al. 2011). The only exception was H. quadripunctata, which has spiny larvae similar to those of H. axyridis but was asymmetrically vulnerable to H. axyridis in the fourth instar and pre-pupal stages (Ware and Majerus 2008). Three species that are also vulnerable to IGP by H. axyridis in Petri-dishes were nevertheless given a score of 2. Propylea quattuordecimpunctata larvae are noticeably long-legged and mobile and are, therefore, more able to escape attack in the field (R. Poland, unpublished data). In addition, larvae of C. septempunctata are commonly reported to drop from a host plant if a potential predator approaches (Sato et al. 2005; Raak-van den Berg et al. 2012). Platynaspis luteorubra was tested only in the fourth instar, by Rondoni et al. (2012a, b). Although the IGP was asymmetric in favour of H. axyridis, they noticed that P. luteorubra was not very susceptible to attack by H. axyridis, which exhibited lower rates of successful attack and predation on P. luteorubra than, e.g., A. bipunctata.
Final risk assessment
Four species, A. bipunctata, A. decempunctata, C. decemguttata and O. conglobata achieved the maximum possible risk score of 18. Myzia oblongoguttata was scored 15 and C. quinquepunctata, C. undecimpunctata and H. quadripunctata were given a score of 12. These four species are therefore also considered as being at relatively high risk. Eleven species achieved a score of 8–10, suggesting a moderate risk, and 11 species reached a low risk score of 2–6 (Table 3).
Discussion
The risk assessment method presented here allows us to rank European coccinellids according to their order of risk from negative interactions with H. axyridis, thus informing the direction and focus of conservation and control initiatives. The four species identified as being most at risk, A. bipunctata, A. decempunctata, C. decemguttata and O. conglobata, are usually found on deciduous trees, where H. axyridis is also found most commonly. They are primarily aphidophagous and have immature stages which are highly vulnerable to IGP by H. axyridis. Calvia quatuordecimguttata is another aphidophagous species living on broadleaved trees, and, thus, is likely to encounter and compete with H. axyridis in the field. However, in contrast to other European species, its eggs are protected by a chemical deterrent and its larvae are protected by strong spines, lowering the risk of displacement by H. axyridis (Ware et al. 2008b; Katsanis et al. 2013, 2016).
Phytophagous and mycophagous ladybird species are clearly at lower risk because they do not compete for the same resource and will be less likely to encounter H. axyridis than homopteran-feeding ladybirds. Aphidophagous species found mainly or exclusively on conifers and in grasslands seem to be at medium risk. Harmonia axyridis shows a strong preference for broadleaved habitats compared to conifers and grasslands, as shown by our asymmetric habitat overlap index calculated in Switzerland. However, observations from Belgium show that it can also be a dominant species on conifers (Adriaens et al. 2008). It is also very abundant within herbaceous crops (e.g. Colunga-Garcia and Gage 1998; Jansen and Hautier 2008) but surveys in Switzerland and Britain showed that, more than ten years after its arrival, it is still an uncommon species in grasslands, except when specific plants, such as nettles, are present (Roy et al. 2011; Kenis, unpublished data).
Among the three risk components considered in the method, the assessment of the likelihood of encounter is a key element, albeit difficult to assess, particularly for uncommon species. We were fortunate that data from ladybird surveys were available from three countries over a relevant time period. However, these surveys were not carried out specifically for the purpose of risk assessment and, therefore, the data are not ideal for assessing habitat overlap. In particular, data from Belgium and, to a lesser extent, Britain, are available at relatively low spatial resolution, which may still contain different habitats, such as broadleaved trees and conifers or grasslands. Because of this scale issue, the likelihood of encounter of a species with H. axyridis could be over-estimated. However, the results are relatively consistent with a previous study showing high niche similarity between H. axyridis and these species at lower spatial scale, i.e. based on co-occurrence on the same plants (Adriaens et al. 2008). The common generalist species C. septempunctata appears to be stable in distribution and abundance across Europe, despite the arrival of H. axyridis (Roy et al. 2012). The defensive behavioural strategy of its larvae may partly explain this. However, this species is mainly associated with herbaceous vegetation and low-scale spatio-temporal co-occurrence indices may overestimate the likelihood of encounter with H. axyridis. Swiss records were made at a higher spatial resolution, i.e. a specific meadow, a broadleaved hedge or a group of trees of a single conifer species. However, the Swiss dataset is smaller than the datasets from the two other countries and so less species could be properly assessed for their co-occurrence with H. axyridis. For example, C. quinquepunctata and C. undecimpunctata, found mainly on low vegetation, were not present in the Swiss dataset and it is possible that, for these two species, the likelihood of encounter based on the Belgian and British dataset (2) and, consequently, the final risk scores (15) were overestimated. Furthermore, all records in Switzerland and most of them in Britain and Belgium were of adult ladybirds, while co-occurrence of larvae would be especially relevant since immature life stages are more susceptible to IGP and competition for food than adults, which are more mobile (Pell et al. 2008). However, for a given ladybird species, the adults and larvae use the same feeding resources and tend to frequently co-occur (e.g. Brown et al. 2011). It could be argued that the likelihood of encounter could be derived from general habitat preference data gathered from the literature. However, such data are too vague for most native coccinellid species, and often inconsistent between information sources and geographic areas.
Effects of direct interactions and defensive mechanisms are straightforward to assess but the rearing of high numbers of ladybird species is time-consuming and some species are difficult to rear due to their specific diets. Therefore, these effects could only be assessed by the authors for 12 species and retrieved from other publications for two additional species. For the remaining 16 species, putative predation scores could be derived from defence mechanisms of taxonomically or morphologically related species. However, without specific IGP experiments, it would have been impossible to observe, e.g. that C. quatuordecimguttata eggs are chemically protected against predation while eggs of the closely-related C. decemguttata are not (Katsanis et al. 2016). Large larval spines, such as those of H. axyridis, A. ocellata and C. quatuordecimguttata seem to provide effective mechanical defence against IGP (Katsanis et al. 2013). However, other spiny larvae such as those of H. quadripunctata are vulnerable to H. axyridis predation (Ware and Majerus 2008). Furthermore, unpublished observations (A. Katsanis) showed that H. axyridis larvae are able to avoid spiny larvae of Exochomus quadripustulatus (L.) by biting them at their softer ventral surface. Other experiments, using H. axyridis larvae in which spines had been removed by hand, showed that spines of H. axyridis allow reducing the bites from C. septempunctata but do not increase survival, suggesting that spines are not the major component of its defence (Hautier 2012). For these reasons, all non-tested species were given a medium predation score of 2 until proper experiments are made. It must be noted that, even if the non-tested species had been scored high (3) in IGP tests, only one of them, M. oblongoguttata, would present a final risk that is as high as that of the top four species because all the others showed a lower likelihood of encounter. It could also be argued that observation of IGP in a Petri-dish does not necessarily translate to occurrence in the field. Analyses of exogenous alkaloids or DNA in field-collected H. axyridis provide useful tools but will generally identify the most common species (Hautier et al. 2011; Thomas et al. 2013; Rondoni et al. 2014; Brown et al. 2015). Furthermore, the abundance of the extra-guild prey (e.g. aphids) may affect IGP (Nóia et al. 2008). Thus, when possible, extraguild prey should be included in IGP tests.
Dietary overlap is the best determinant we found for evaluating the level of hazard due to competition for food, although we realise that this parameter is linked to the likelihood of encounter, which depends on resource similarity, since eggs are laid close to resources (Majerus 1994). One way to improve the assessment would be to consider the ladybird diet more precisely, i.e. to prey species or genus level, but this requires a precise knowledge of ladybird diets and preferences, which, for the moment, is largely lacking (Hodek and Evans 2012). Competitive interaction studies on competition for food between H. axyridis and European ladybirds are rare. Soares and Serpa (2007) showed that the presence of H. axyridis adults significantly affected the reproductive capacity of C. undecimpunctata even when the resource was not in short supply.
It remains to be seen whether long-term studies on ladybird species abundance that are presently being carried out in the three countries will validate our risk assessments. The Holarctic A. bipunctata, one of the species identified as most at risk in this study, has declined severely in the last 20 years in North America, possibly due to the invasion of H. axyridis and other exotic ladybirds (Harmon et al. 2007; Bahlai et al. 2015). Strong evidence of a decline of A. bipunctata and, to a lesser extent, other native species has been demonstrated in Britain, Belgium and Switzerland (Brown et al. 2011; Roy et al. 2012; Comont et al. 2014; Adriaens et al. 2015). In Belgium and Britain, H. axyridis has been shown to commonly feed on Adalia spp. in the field (Hautier et al. 2011; Thomas et al. 2013; Rondoni et al. 2014; Brown et al. 2015). Interestingly, A. bipunctata, the species that shows by far the strongest decline in Europe, is also the one that showed the highest likelihood of encounter with H. axyridis in our study, in particular in Switzerland where records were made at the highest spatial resolution. Had we based this assessment solely on the literature, we would probably have considered the congeneric A. decempunctata as having a higher likelihood of encountering H. axyridis than A. bipunctata because of its strong association with arboreal, broadleaved habitats. However, general long-term surveys, while suitable for assessing trends in abundant species, are less appropriate for detecting effects on rare species, which were sparsely recorded prior to the arrival of the invasive alien species. Therefore, it is essential that specific studies are conducted focusing on species found historically at lower abundance and which were also ascribed high scores in our risk assessment (such as C. decemguttata, O. conglobata and C. quinquepunctata). The relative scarcity of these species coupled with their restricted ecological niches could enhance the risk of local extinction.
Many generic assessment methods exist to assess the broad risk of invasive species, including predators, introduced accidentally or as biological control agents (e.g. van Lenteren et al. 2006; D’hondt et al. 2015; Kumschick et al. 2015). Other, more specific risk assessments focus on the hazard of predation on a single prey (e.g. Koch et al. 2006). However, the risk assessment method presented here is unique in the sense that it allows us to rank a whole set of native species, according to their order of risk from negative interactions with the invasive species. It is hoped that the risk assessment method proposed here could be applied to other situations where the arrival of an alien predator potentially threatens native species through competition and intra-guild or extra-guild predation. Classifying competitor or prey species according to their exposure and vulnerability, and selecting the most vulnerable species for research, protection and conservation programmes could be a useful approach. Potential candidates include, among others, several invasive alien predatory wasps and hornets that threaten biodiversity worldwide. For example, the Asian hornet, Vespa velutina, a recent invader in Western Europe, may displace various native insects such as bees, flies and vespid wasps through direct predation and competition (Beggs et al. 2011). The same approach could also be considered for assessing the risk of invasive ants since these are also generalist predators that are known to threaten native species, in particular ants, through various mechanisms (Holway et al. 2002). Furthermore, this approach is not limited to insects. Other predatory terrestrial and aquatic invertebrates would be suitable for similar assessments. For example, the killer shrimp, Dikerogammarus villosus, has undergone an explosive invasion in Europe in the last two decades and has recently been found in Britain (MacNeil et al. 2010), where a similar risk assessment could be performed to evaluate which native gammarids or other aquatic invertebrates are most at risk. A similar protocol could be developed for invasive alien predaceous fish, amphibians, reptiles and even mammals, with some taxon-specific adaptations. The approach is primarily applicable to assess the risk posed to the displacement of native biodiversity by an invasive alien predator that is at an early stage of invasion, for which a firm impact cannot yet be ascertained but habitat and food overlaps can already be calculated. The approach should be used with more caution for risk assessments prior to invasion, but could for example complement pre-release risk assessment protocols for biological control agents (van Lenteren et al. 2006; Hajek et al. 2016), in which case a likelihood of encounter score could be estimated based on parameters such as general habitat requirements or feeding niches defined from data collected in the present distribution range of the species.
References
Adriaens T, Martin San, y Gomez G, Maes D (2008) Invasion history, habitat preferences and phenology of the invasive ladybird Harmonia axyridis in Belgium. BioControl 53:69–88
Adriaens T, San Martin y Gomez G, Maes D, Brosens D, Desmet P (2012) Belgian Coccinellidae—Ladybird beetles in Belgium. v1.0. Research Institute for Nature and Forest (INBO). Dataset/Occurrence. http://doi.org/10.15468/0refva. Accessed 15 Mar 2016
Adriaens T, Martin S, y Gomez G, Bogaert J, Crevecoeur L, Beuckx J-P, Maes D (2015) Testing the applicability of regional IUCN Red List criteria on ladybirds (Coleoptera, Coccinellidae) in Flanders (north Belgium): opportunities for Conservation. Insect Conserv Divers 8:404–417
Bahlai CA, Colunga-Garcia M, Gage SH, Landis DA (2015) The role of exotic ladybeetles in the decline of native ladybeetle populations: evidence from long-term monitoring. Biol Invasions 17:1005–1024
Beggs JR, Brockerhoff EG, Corley JC, Kenis M, Masciocchi M, Muller F, Rome Q, Villemant C (2011) Ecological effects and management of invasive alien Vespidae. BioControl 56:505–526
Bellard C, Cassey P, Blackburn TM (2016) Alien species as a driver of recent extinctions. Biol Lett 12:20150623
Brown PMJ, Frost R, Dobersk J, Sparks T, Harrington R, Roy HE (2011) Decline in native ladybirds in response to the arrival of Harmonia axyridis: early evidence from England. Ecol Entomol 36:231–240
Brown PMJ, Ingels B, Wheatley A, Rhule EL, de Clercq P, van Leeuwen T, Thomas A (2015) Intraguild predation by Harmonia axyridis (Coleoptera: Coccinellidae) on native insects in Europe: molecular detection from field samples. Entomol Sci 18:130–133
Colunga-Garcia M, Gage SH (1998) Arrival, establishment, and habitat use of the multicolored Asian lady beetle (Coleoptera: Coccinellidae) in a Michigan landscape. Environ Entomol 27:1574–1580
Comont RF, Roy HE, Harrington R, Shortall CR, Purse BV (2014) Ecological correlates of local extinction and colonisation in the British ladybird beetles (Coleoptera: Coccinellidae). Biol Invasions 16:1805–1817
D’hondt B, Vanderhoeven S, Roelandt S, Mayer F, Versteirt V, Adriaens T, Ducheyne E, San Martin G, Grégoire JC, Stiers I, Quoilin S, Cigar J, Heughebaert A, Branquart E (2015) Harmonia+ and Pandora+: risk screening tools for potentially invasive plants, animals and their pathogens. Biol Invasions 17:1869–1883
Eschen R, Babendreier D, Nauer S, Bigler F, Kenis M (2007) Surveys for ladybirds (Coleoptera: Coccinellidae) in Switzerland and confirmation of the presence of the invasive alien ladybird species Harmonia axyridis (Pallas). Mitt Schweiz Entomol Ges 80:7–14
Félix S, Soares AO (2004) Intraguild predation between the aphidophagous ladybird beetles Harmonia axyridis and Coccinella undecimpunctata (Coleoptera: Coccinellidae): the role of body weight. Eur J Entomol 101:237–242
Hajek AE, Hurley BP, Kenis M, Garnas JR, Bush SJ, Wingfield MJ, van Lenteren JC, Cock MJ (2016) Exotic biological control agents: a solution or contribution to arthropod invasions? Biol Invasions 18:953–969
Harmon JP, Stephens E, Losey J (2007) The decline of native coccinellids (Coleoptera: Coccinellidae) in the United States and Canada. J Insect Conserv 11:85–94
Harris R, Abbott K, Barton K, Berry J, Don W, Gunawardana D, Lester P, Rees J, Stanley M, Sutherland A, Toft R (2005) Invasive ant pest risk assessment project for Biosecurity New Zealand. Series of unpublished Landcare Research contract reports to Biosecurity New Zealand. BAH/35/2004-1
Hautier L (2012) Intraguild predation in Harmonia axyridis and its effects on native coccinellids. PhD thesis, Université Libre de Bruxelles, Belgium
Hautier L, Martin San, y Gomez G, Callier P, de Biseau J, Gregoire J (2011) Alkaloids provide evidence of intraguild predation on native coccinellids by Harmonia axyridis in the field. Biol Invasions 13:1805–1814
Hodek I, Evans EW (2012) Food relationships. In: Hodek I, van Emden HF, Honek A (eds) Ecology and behaviour of the ladybird beetles (Coccinellidae). Wiley-Blackwell, Chichester, pp 141–274
Hodek I, van Emden HF, Honek A (2012) Ecology and behaviour of the ladybird beetles (Coccinellidae). Wiley-Blackwell, Chichester
Holway DA, Lach L, Suarez A, Tsutsui N, Case TJ (2002) The causes and consequences of ant invasions. Annu Rev Ecol Syst 33:181–233
Hulme P, Bacher S, Kenis M, Klotz S, Kuhn I, Minchin D, Nentwig W, Olenin S, Panov V, Pergl J, Pysek P, Roques A, Sol D, Solarz W, Vila M (2008) Grasping at the routes of biological invasions: a framework for integrating pathways into policy. J Appl Ecol 45:403–414
Jansen J-P, Hautier L (2008) Ladybird population dynamics in potato: comparison of native species with an invasive species, Harmonia axyridis. BioControl 53:223–234
Katsanis A, Babendreier D, Nentwig W, Kenis M (2013) Intraguild predation between the invasive ladybird Harmonia axyridis and non-target European coccinellid species. BioControl 58:73–83
Katsanis A, Magro A, Ramon-Portugal F, Kenis M, Babendreier D (2016) Chemical defences of native European coccinellid eggs against intraguild predation by the invasive Asian coccinellid, Harmonia axyridis (Pallas). BioControl, this issue
Kenis M, Auger-Rozenberg M-A, Roques A, Timms L, Péré C, Cock MJW, Settele J, Augustin S, Lopez-Vaamonde C (2009) Ecological effects of invasive alien insects. Biol Invasions 11:21–45
Koch RL, Hutchison WD, Venette RC, Heimpel GE (2003) Susceptibility of immature monarch butterfly, Danaus plexippus (Lepidoptera: Nymphalidae: Danainae), to predation by Harmonia axyridis (Coleoptera: Coccinellidae). Biol Control 28:265–270
Koch RL, Venette RC, Hutchison WD (2006) Predicted impact of an exotic generalist predator on monarch butterfly (Lepidoptera: Nymphalidae) populations: a quantitative risk assessment. Biol Invasions 8:1179–1193
Kumschick S, Richardson DM (2013) Species-based risk assessments for biological invasions: Advances and challenges. Divers Distrib 19:1095–1105
Kumschick S, Bacher S, Marková Z, Pergl J, Pyšek P, Vaes-Petignat S, van der Veer G, Vilà M, Nentwig W (2015) Comparing impacts of alien plants and animals using a standard scoring system. J Appl Ecol 52:552–561
Long JL (2003) Introduced mammals of the world. Their history distribution and influence. CABI, Wallingford
MacNeil C, Plavoet D, Dick JTA, Fielding N, Constable A, Hall N, Aldridge D, Renals T, Diamond M (2010) The Ponto-Caspian ‘killer shrimp’, Dikerogammarus villosus (Sowinsky, 1894), invades the British Isles. Aquat Invasions 5:441–445
Majerus MEN (1994) Ladybirds (No. 81, New Naturalist Series). HarperCollins, London
Michaud JP (2002) Invasion of the Florida citrus ecosystem by Harmonia axyridis (Coleoptera : Coccinellidae) and asymmetric competition with a native species, Cycloneda sanguinea. Environ Entomol 31:827–835
Nóia M, Borges I, Soares AO (2008) Intraguild predation between the aphidophagous ladybird beetles Harmonia axyridis and Coccinella undecimpunctata (Coleoptera: Coccinellidae): the role of intra and extraguild prey densities. Biol Control 46:140–146
Pell JK, Baverstock J, Roy HE, Ware RL, Majerus MEN (2008) Intraguild predation involving Harmonia axyridis: a review of current knowledge and future perspectives. BioControl 53:147–168
Polis GA, Myers CA, Holt RD (1989) The ecology and evolution of intraguild predation: potential competitors that eat each other. Annu Rev Ecol Syst 20:297–330
Raak-van den Berg CL, De Lange HJ, van Lenteren JC (2012) Intraguild predation behaviour of ladybirds in semi-field experiments explains invasion success of Harmonia axyridis. PLoS ONE 7(7):e40681
Rondoni G, Onofri A, Ricci C (2012a) Laboratory studies on intraguild predation and cannibalism among coccinellid larvae (Coleoptera: Coccinellidae). Eur J Entomol 109:353–362
Rondoni G, Onofri A, Ricci C (2012b) Differential susceptibility in a specialised aphidophagous ladybird, Platynaspis luteorubra (Coleoptera: Coccinellidae), facing intraguild predation by exotic and native generalist predators. Biocontrol Sci Technol 22:1334–1350
Rondoni G, Athey KJ, Harwood JD, Conti E, Ricci C, Obrycki JJ (2014) Development and application of molecular gut-content analysis to detect aphid and coccinellid predation by Harmonia axyridis (Coleoptera: Coccinellidae) in Italy. Insect Sci 22:719–730
Roy HE, Brown PMJ, Frost R, Poland RL (2011) Ladybirds (Coccinellidae) of Britain and Ireland. NERC Centre for Ecology & Hydrology, Wallingford
Roy HE, Adriaens T, Isaac NJB, Kenis M, Onkelinx T, San Martin G, Brown PMJ, Hautier L, Poland R, Roy DB, Comont R, Eschen R, Frost R, Zindel R, van Vlaenderen J, Nedvěd O, Ravn HP, Grégoire J-C, de Biseau J-C, Maes D (2012) Invasive alien predator causes rapid declines of native European ladybirds. Divers Distrib 18:717–725
Roy HE, Brown PMJ, Adriaens T, Berkvens N, Borges I, Clusella-Trullas S, De Clercq P, Comont RF, Eschen R, Estoup A, Evans EW, Facon B, Gardiner MM, Gil A, Grez AA, Guillemaud T, Haelewaters D, Herz A, Honek A, Howe AG, Hui C, Hutchison WD, Kenis M, Koch RL, Kulfan J, Lawson Handley L, Lombaert E, Loomans A, Losey J, Lukashuk AO, Maes D, Magro A, Murray KM, San Martin G, Martinkova Z, Minnaar IA, Nedved O, Orlova-Bienkowskaja MJ, Rabitsch W, Ravn HP, Rondoni G, Rorke SL, Ryndevich SK, Saethre M-G, Sloggett JJ, Soares AO, Stals R, Tinsley MC, Vandereycken A, van Wielink P, Viglášová S, Zach P, Zakharov IA, Zaviezo T, Zhao Z (2016) The harlequin ladybird. Harmonia axyridis: global perspectives on invasion history and ecology. Biol Invasions 18:997
Sato S, Yasuda H, Evans EW (2005) Dropping behaviour of larvae of aphidophagous ladybirds and its effects on incidence of intraguild predation: interactions between the intraguild prey, Adalia bipunctata (L.) and Coccinella septempunctata (L.), and the intraguild predator, Harmonia axyridis Pallas. Ecol Entomol 30:220–224
Snyder WE, Evans EW (2006) Ecological effects of invasive arthropod generalist predators. Annu Rev Ecol Evol Syst 37:95–122
Soares AO, Serpa A (2007) Interference competition between ladybird beetle adults (Coleoptera: Coccinellidae): effects on the growth and reproductive capacity. Pop Ecol 49:37–43
Thomas A, Trotman J, Wheatley A, Aebi A, Zindel R, Brown PMJ (2013) Predation of native coccinellids by the invasive alien Harmonia axyridis (Coleoptera: Coccinellidae): detection in Britain by PCR based gut analysis. Insect Conserv Divers 6:20–27
van Lenteren JC, Bale JS, Bigler F, Hokkanen HMT, Loomans AJM (2006) Assessing risks of releasing exotic biological control agents of arthropod pests. Annu Rev Entomol 51:609–634
Wagner DL, van Driesche RG (2010) Threats posed to rare or endangered insects by invasions of nonnative species. Annu Rev Entomol 55:547–568
Ware RL, Majerus MEN (2008) Intraguild predation of immature stages of British and Japanese coccinellids by the invasive ladybird Harmonia axyridis. BioControl 53:169–188
Ware RL, Evans N, O’Farrell K, Majerus MEN, Malpas L, Michie LJ (2008a) Intraguild predation of British and Japanese coccinellid eggs by the invasive ladybird Harmonia axyridis. Neobiota 7:263–275
Ware RL, Ramon-Portugal F, Magro A, Ducamp C, Hemptinne J-L, Majerus MEN (2008b) Chemical protection of Calvia quatuordecimguttata eggs against intraguild predation by the invasive ladybird Harmonia axyridis. BioControl 53:189–200
Zindel R (2008) The potential impact of an exotic ladybird, Harmonia axyridis (Pallas), on native species in Switzerland. Master Thesis, University of Fribourg, Switzerland
Acknowledgments
The authors acknowledge the help and support of I. Wright and L.-J. Michie, formerly of the Department of Genetics, University of Cambridge, UK. The work in Switzerland was supported by the EU project ALARM (GOCE-CT-2003-506675) and GRANTs from the Swiss Federal Office for the Environment (F232-0377) and the Swiss National Science Foundation (3100A0-117831). HER is supported by the Natural Environment Research Council, Joint Nature Conservation Committee and the Department for the Environment, Food and Rural Affairs. We are very grateful to all the recorders contributing records to the national surveys in Britain and Belgium.
Author information
Authors and Affiliations
Corresponding author
Additional information
Handling Editor: Lori-Jayne Lawson Handley
Rights and permissions
About this article
Cite this article
Kenis, M., Adriaens, T., Brown, P.M.J. et al. Assessing the ecological risk posed by a recently established invasive alien predator: Harmonia axyridis as a case study. BioControl 62, 341–354 (2017). https://doi.org/10.1007/s10526-016-9764-x
Received:
Accepted:
Published:
Issue Date:
DOI: https://doi.org/10.1007/s10526-016-9764-x