Abstract
Trichomoniasis is the most common non-viral sexually transmitted disease worldwide and can lead to serious consequences in reproductive health, cancer, and HIV acquisition. The current approved treatment present adverse effects and drug resistance data on this neglected parasitic infection is underestimated. Chalcones are a family of molecules that present biological applications, such as activity against many pathogenic organisms including protozoan pathogens. Chalcone (1) and three amino-analogues (2–4) were synthesized by Claisen–Schmidt condensation reaction and had their activity evaluated against the parasitic protozoan Trichomonas vaginalis. This bioassay indicated the presence and position of the amino group on ring A was crucial for anti-T. vaginalis activity. Among these, 3′-aminochalcone (3) presented the most potent effect and showed high cytotoxicity against human vaginal cells. On the other hand, 3 was not able to exhibit toxicity against Galleria mellonella larvae, as well as the hemolytic effect on human erythrocytes. Trophozoites of T. vaginalis were treated with 3, and did not present significant reactive oxygen species (ROS) accumulation, but induced a significantly higher ROS accumulation in human neutrophils after co-incubation. T. vaginalis pyruvate:ferredoxin oxidoreductase (PFOR) and β-tubulin gene expression was not affected by 3.
Similar content being viewed by others
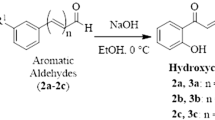
Avoid common mistakes on your manuscript.
Introduction
The flagellate protozoan Trichomonas vaginalis (Donné 1836) is the etiological agent of trichomoniasis, a sexually transmitted infection (STI) that represents a heavy burden on public health. Trichomoniasis is the most common non-viral STD worldwide, and in 2008, about 280 million new cases were reported (WHO 2012). Infection can lead to serious consequences such as cervical cancer (Viikki et al. 2000) and predisposition to prostate cancer (Sutcliffe et al. 2012). Reproductive outcomes may also occur, such as pelvic inflammatory disease (Cherpes et al. 2006) and infertility (Grodstein et al. 1993), and also premature birth and low birth weight (Cotch et al. 1997). T. vaginalis also acts as a facilitating agent for HIV and HPV transmission and acquisition (Kissinger and Adamski 2013, Noël et al. 2010).
Currently, 5-nitromidazoles (metronidazole and tinidazole) are the only therapeutic agents for trichomoniasis approved by the Food and Drug Administration (FDA, USA). However, besides limitations such as adverse effects, these drugs present an increasing number of infections that do not respond to treatment due to drug resistance (Kirkcaldy et al. 2012). Also, the absence of a surveillance system for this infection indicates that both numbers on infected patients and data on resistance are probably underestimated and, therefore, trichomoniasis is considered a neglected parasitic infection (Secor et al. 2014). New alternatives have been researched, and many substances have presented good activity against T. vaginalis in vitro, but no treatment options have progressed to clinical trials (Vieira et al. 2017).
Natural and synthetic chalcone analogues are privileged structures to medicinal chemistry and present activities against many pathogenic organisms, including protozoan pathogens, with promising results and several proteins/enzymes as possible targets (Mahapatra et al. 2015). Besides antiparasitic, chalcones present anti-inflammatory, antioxidant, anticancer, antinociceptive, and antiplatelet activities and are considered hepatoprotective agents (Karimi-Sales et al. 2018). The proposed mechanisms of action include the inhibition of mitochondrial dehydrogenases and fumarate reductase causing an interference with the structure and function of the mitochondria of Leishmania species (Zhai et al. 1995, 1999; Chen et al. 2001). The anticancer activity of chalcones might be caused by the induction of apoptosis, DNA, and mitochondrial damage, inhibition of kinases, angiogenesis, and tubulin polymerization (Das and Manna 2016). Recently, two studies have demonstrated the anti-T. vaginalis activity of metronidazole-chalcone conjugates (Anthwal et al. 2014), and chalconyl blended triazole allied organosilatranes (Singh et al. 2016).
Chalcone-derivatives potential activity against T. vaginalis is a field yet to be explored. In this sense, this study presents the anti-T. vaginalis potential of synthesized chalcone derivatives and some biological effects of 3′-aminochalcone.
Material and methods
Chalcone-derivatives synthesis
Chalcone (1) and its amino-analogues (2–4) were synthesized by Claisen–Schmidt aldol condensation reactions between acetophenone derivatives and benzaldehydes (Scheme 1), using the protocol described by Zeraik and coauthors, with minor modifications (Zeraik et al. 2012). The structure of compounds was confirmed by 1H NMR and 13C NMR spectra analysis as presented in supplementary material.
In vitro culture of T. vaginalis
The T. vaginalis isolate 30236 (from the American Type Culture Collection (ATCC), USA) was used in this study. Parasites were cultured in vitro in a trypticase-yeast extract-maltose (TYM) medium pH 6.0 supplemented with 10% (v/v) heat-inactivated adult bovine serum, and incubated at 37 °C (Diamond 1957). Trophozoites in the logarithmic phase of growth and exhibiting more than 95% viability and normal morphology were harvested, centrifuged, and resuspended in new TYM medium for the experimental assays.
Screening of anti-T. vaginalis activity of chalcone derivatives
The activity of chalcone derivatives against T. vaginalis was performed in 96-well microtiter plates. Dimethyl sulfoxide (DMSO) was used as a solubilization vehicle for the compounds at 0.6% final concentration. Trichomonads suspension at 2.0 × 105 trophozoites/milliliter density was incubated with the compounds at 100 μM for 24 h in a humidified 5% CO2 incubator at 37 °C. The viability of parasites was assessed by counting in Neubauer chamber using trypan blue (0.2%) as an exclusion dye. Three controls were carried out: negative controls with trophozoites in a supplemented culture medium only, vehicle control with DMSO at 0.6%, and metronidazole control at 100 μM. Results were expressed as the percentage of viable trophozoites compared to untreated parasites. Compounds that presented the best activities were tested to determine the half maximal inhibitory concentration (IC50).
Determination of IC50 values
For the determination of the IC50 values of the most active compound, the experiments were performed in similar conditions in the screening experiments, with compound concentrations ranging from 100 to 0.7812 μM. The IC50 values were assessed using GraphPad Prism6 software through a nonlinear regression model.
Growth kinetics
The aminochalcone with the best IC50 value was incubated with T. vaginalis ATCC30236 for the evaluation of its effect on growth kinetics. Parasite suspensions at 2.0 × 105 trophozoites/milliliter final density were incubated with the compound in the IC50; negative and vehicle control (DMSO 0.6%) were also evaluated. The parasites were counted in a Neubauer chamber using trypan Blue (0.2%) as an exclusion dye after 2, 4, 6, 12, 24, 48, 72, 96, and 120 h of incubation. Results were expressed as the number of viable trophozoites per milliliter.
Cytotoxicity against vaginal epithelial cells (HMVII)
The compound that presented the best IC50 value was tested for cytotoxicity against HMVII vaginal epithelial cell lineage. The culture was maintained in RPMI-1640 medium supplemented with 20% fetal bovine serum. The day before the experiment cells were seeded in a 96-well microtiter and kept overnight at 37 °C in humidified 5% CO2 incubator to reach pre-confluence. After this period, the medium was replaced by the molecule in concentrations ranging from 100 to 0.7812 μM in the supplemented medium. Triton X100 0.02% was added as a positive control and 0.6% DMSO as a vehicle control. The plates were incubated for 48 h in a humidified 5% CO2 incubator at 37 °C. After this period, the cell viability was evaluated by the MTT assay (Mosmann 1983). Briefly, the medium was removed, a 0.5 mg/mL 3-(4,5-dimethylthiazol-2-yl)-2,5-diphenyltetrazolium bromide (MTT) solution was added and incubated for 1 h and removed, and the insoluble purple formazan was dissolved in DMSO and the absorbance was read at 570 nm. The concentration required to kill 50% of the cells (CC50) was determined using GraphPad Prism6 software, through a nonlinear regression model.
In vivo toxicity against Galleria mellonella model
The aminochalcone with the best IC50 value was also tested for toxicity in vivo using the Galleria mellonella (Linnaeus, 1758) larvae model. Larvae weighing 280 ± 20 mg were separated into three groups of ten for each condition. Three concentrations were tested: 1 mg/kg, 10 mg/kg, and 50 mg/kg of larvae, and saline solution was used as the vehicle. Larvae were injected through the last left pro-leg with 10 μL of the solutions using a Hamilton syringe (Sigma Aldrich®, USA) (Kavanagh and Fallon 2010). Three control conditions were performed: negative control (not injected larvae), vehicle control, and positive control (larvae injected with DMSO only). Larvae were kept in Petri dishes in an incubator at 37 °C and viability was observed in 24, 48, 72, 96, and 120 h. Determination of larvae viability was made by the evaluation of reorientation after being placed backwards, mobility, and response to stimuli.
Hemolysis assay
Hemolytic effect of the aminochalcone was evaluated against erythrocytes obtained from a heparinized blood of healthy human donors (documents, procedures, and project approved by the Universidade Federal do Rio Grande do Sul Research Ethical Committee under authorization CAAE 47423415.5.0000.5347). Erythrocytes were washed three times in PBS, ressuspended to 1% density on saline pH 7.0, and incubated with the compound in its IC50 and at 100 μM. Experiment controls were: negative, vehicle (0.6% DMSO), and positive (Triton X-100 0.1%). After 1 and 24 h of incubation at 37 °C, supernatant absorbance was measured at 540 nm (Gauthier et al. 2009). Results were expressed as a percentage of hemolysis compared to the positive control.
Determination of reactive oxygen species (ROS) production in T. vaginalis
For the determination of induction of ROS production in trophozoites by the compound, T. vaginalis suspensions at 5 × 106 density were incubated with 10 μM of 2′,7′-dichlorofluorescein diacetate (2′,7′-DCF-DA), an oxidation-sensitive fluorescent probe, for 1 h at 37 °C. After this time, the molecule at IC50 and at 100 μM was added and incubated for an additional 1 h at 37 °C. Parasites treated with hydrogen peroxide (5.0 mM) were used as a positive control. After incubation, fluorescence was measured by flow cytometry (BD FACSVerse™, Becton Dickinson) and 10,000 cells were gated and analyzed using BD FACSuite™ software (Becton Dickinson). Results were expressed in fluorescence units.
Determination of ROS production in neutrophils
Human neutrophils were isolated as previously described (Böyum 1968) with some modifications. Briefly, venous blood of healthy volunteers (documents, procedures, and project approved by the Universidade Federal do Rio Grande do Sul Research Ethical Committee under authorization 19346) was collected on heparin anticoagulant solution, centrifuged (250×g, 10 min, 24 °C), and platelet-rich plasma discarded. Leukocytes were obtained following erythrocyte sedimentation in 2.0% Dextran T-500 and centrifuged (525×g, 20 min, 24 °C) through a layering on Histopaque 1077. The neutrophil-enriched pellet was subjected to a 15-s hypotonic lysis to remove the remaining erythrocytes and centrifuged (1000×g, 5 min, 24 °C). The purified neutrophils were resuspended in RPMI 1640 supplemented with 10% fetal bovine serum and 10 mM HEPES for the experiments.
Parasites (5.0 × 105 trophozoites/milliliter) were treated with the aminochalcone at 100 μM and IC50. After 2 hours, trophozoites were washed three times in PBS, counted in a hemocytometer and resuspended in RPMI medium. Neutrophils (1.0 × 105) were cocultured with treated trophozoites (1.0 × 104) on 96-well microplates at 37 °C in 5.0% CO2-humidified incubator for 30 min. After incubation, the medium was removed and cells were resuspended in PBS with 10 μM 2′,7′-DCF-DA and incubated at 37 °C for 15 min. Hydrogen peroxide (5.0 mM) was added 5 min before reading as positive control for ROS production. The neutrophils fluorescence was measured by flow cytometry (FACSVerse, Becton Dickinson). Ten thousand cells were gated and analyzed using the BD FACSuite™ software (Becton Dickinson). Results were expressed in fluorescence units.
RT-PCR for pyruvate:ferredoxin oxidoreductase (PFOR) and β-tubulin
Trophozoites (4 × 106 trophozoites/milliliter) were treated for 12 and 24 h with the aminochalcone at IC50, then the total RNA was extracted using TriZol™ following the manufacturer’s instructions and was stored at − 80 °C until use. Quantity and purity of the RNA were determined using spectrophotometric method, and only the high-quality samples, in which the A260/A280 was 1.8 and A260/A230 was 2.0, were used for subsequent qRT-PCR analyses. Samples were pretreated with DNAse, to ensure that there was no contamination with genomic DNA in the qRT-PCR analysis.
Real-time PCR reactions were performed in the Rotor-Gene QTM (Qiagen, Netherlands) equipment using GoTaqTM 1-Step RT-qPCR kit (Promega, USA) in a final volume of 12 μL. Each PCR reaction contained 5 μL of 2X Go Taq qPCR Master Mix, 200 nM of DNATopII, and PFOR primers, and 100 nM of β-tubulin primer, 0.2 μL Go Script RT Mix, and 50 ng of RNA. The qRT-PCR cycling conditions were as follows: reverse transcription for 15 min at 37 and 95 °C for 10 min, followed by 40 cycles of denaturation at 95 °C for 10 s and annealing at 64 °C for 30 s, with fluorescence data collection recording at this step, and extension at 72 °C for 30 s. The melting curve analyses were performed by raising the temperature at the end of each run in by 1 °C per 5 s from 64 to 95 °C. Parallel reactions without RNA template were used as a negative control. Primers used were: DNATopII forward (F): 5’ATCGGTGTCGGTTGGTCAAG3’ and reverse (R): 5’TGGCTGTTTGACACCGTCTTT3’; β-tubulin F: 5’TCCGTGGCCGTATGTCATCT3’, R: 5’GCTGTTGTGTTGCCGATGAA3’; PFOR F: 5’CTCGTTTGGGGTGCTACATT3’, R: 5’TCCTGATCCCAAACCTTGAG3’ (dos Santos et al. 2015).
The values of the cycle threshold (Ct) for each reaction were calculated by the Rotor-Gene Q series software 2.1.0 and these values were used to determine the average Ct for each sample. The relative change in gene expression was analyzed using the 2-ΔΔCt method (Livak and Schmittgen 2001).
Statistical analysis
All experiments were performed with three independent samples, in triplicates. Results were expressed as means ± SD. Statistical analysis was conducted using the Student’s t test for comparison between two groups. Statistical significance was considered at p < 0.05.
Results
Chalcone derivatives synthesis
In this study, chalcone derivatives were synthesized by Claisen–Schmidt base-catalyzed aldol condensation reactions. These reactions were feasible and versatile, allowing the synthesis of library of compounds, with high chemodiversity (Zhuang et al. 2017). Chalcones 1, 2, 3, and 4 were obtained in yields of 78%, 82%, 55%, and 60%, respectively. Structures of 1–4 were confirmed by 1H NMR and 13C NMR spectra analysis. All chalcones are known compounds and their NMR data were confirmed by comparison with literature data. For chalcones 1 and 4 were compared with Santos and co-authors (2017) (Santos et al. 2017), while chalcones 2 and 3 were compared with Iqbal and collaborators (2014) (Iqbal et al. 2014). The most typical signals in the 1H NMR spectra were a pair of doublets with coupling constants values of 15.0 Hz. In the 13C NMR spectrum, the most downfield signals with chemical shifts ranging from 186.5 to 191.0 ppm were attributed to the carbonyl of the α,β-unsaturated ketone moiety. For all compounds, NMR data (chemical shifts, integrations, multiplicities, and coupling constants) corresponded with the proposed structures.
Chalcone derivatives anti-T. vaginalis activity
The compounds had their activity screened against T. vaginalis ATCC 30236 (JH 31A #4, from the American Type Culture Collection, USA) tested at 100 μM. (Fig. 1). Chalcone (compound 1) was inactive showing a percentage of viability value equals to 122 ± 21. Amino-analogues of 1 (2–4) exhibited antiprotozoal activities. Aminochalcone 3 displayed IC50 of 29 μM. Chalcones 2 and 4 with an amino group at positions 2′ and 4′, respectively, exhibited inferior potency against T. vaginalis, showing the percentage of viability values of 50 ± 10 and 10 ± 3, respectively.
Compound 3 affects T. vaginalis growth kinetics
For the evaluation of the effect of compound 3 on growth kinetics, trophozoites incubated with the molecule at IC50 were counted over time, up to 120 h. The results demonstrate trophozoites viability is affected by the compound over time (Fig. 2).
Kinetic growth curve of trophozoites treated with compound 3 in 29 μM in comparison to untreated parasites (control) and parasites with 0.6% DMSO (vehicle). The initial inoculum was 2.0 × 105 trophozoites/mL. Results are presented as mean ± standard deviation of three independent experiments in triplicate
Compound 3 is toxic to vaginal epithelial cells in vitro
Compound 3, which presented the highest potency against T. vaginalis, had its cytotoxicity against HMVII cells assessed. A nonlinear regression model was used in order to determine the CC50 value, which is 21 μM. The selectivity index is the ratio between the values of CC50 and IC50 and indicates whether the compound toxicity is selective against the parasite, and SI for compound 3 is 0.72.
Galleria mellonella larvae injected with compound 3 maintained viability
Galleria mellonella larvae injected with 3 at 1 mg/kg, 10 mg/kg, and 50 mg/kg showed no significant increase in mortality up to 120 h of observation at all conditions tested (p > 0.05) (Table 1).
Compound 3 does not induce hemolysis in human erythrocytes
Human erythrocytes were incubated with compound 3 for 1 and 24 h and the hemolysis rate at 100 μM and IC50 did not present any significant difference form the negative control group (p > 0.05) (Table 2).
Compound 3 does not induce ROS accumulation in T. vaginalis trophozoites
Trophozoites treated with 3 were evaluated for ROS accumulation by flow cytometry. Results indicate that the compound did not induce ROS accumulation in the trophozoites in any significant manner (Fig. 3).
T. vaginalis induced ROS production in neutrophils is increased by compound 3
ROS production by neutrophils incubated with T. vaginalis trophozoites pretreated with compound 3 was increased in a significant manner (Fig. 4). On the other hand, neutrophils incubated only with 3 presented significant reduction in ROS production at 100 μM concentration.
ROS accumulation in neutrophils incubated with compound 3, and neutrophils cocultured with treated and non-treated T. vaginalis trophozoites. N, non treated neutrophils; N + 3100 μM, N + 3 29 μM, neutrophils incubated with compound 3 at different concentrations; N + TV, neutrophils coincubated with non-treated T. vaginalis trophozoites; N + TV + 3100 μM, N + TV + 3 29 μM, neutrophils incubates with T. vaginalis trophozoites treated with the compound in different concentrations. a Significant reduction, b significant increase (p < 0.05). Hydrogen peroxide (5.0 mM)–treated neutrophils presented 3154.2 ± 445.3 fluorescence units
PFOR and β-tubulin gene expression are not affected by compound 3
Trophozoites treated for 12 and 24 h did not appear to express PFOR gene nor β-tubulin differently different form control group in a significant manner (Table 3).
Discussion
Trichomoniasis is the most common non-viral STI worldwide and it can lead to serious health consequences. Treatment is still restricted to two drugs, metronidazole and tinidazole. Taking into account the increasing incidence of resistance to these drugs, treatment has become a challenge, therefore, the increasing need to search for new therapeutic alternatives (Vieira et al. 2017). In recent years, chalcone-based compounds have shown a wide range of biological activities, including activity against pathogenic agents, especially protozoa, and T. vaginalis (Anthwal et al. 2014, Singh et al. 2016).
The 3′-aminochalcone (compound 3) exhibited potent activity against T. vaginalis (IC50 = 29 μM) demonstrating the importance of the 3′ position of ring A. While aminochalcones with amino group in positions 2′ and 4′ exhibited a less potent activity against T. vaginalis showing the percentage of viability values equal to 50 ± 10 and 10 ± 3, respectively. Altogether, these data suggest that the presence and position on ring A of the amino group improved antiprotozoal activity. Amino group substitutions in the chalcone framework have been shown to play a central role in the bioactivity. Thus, aminochalcone 3 was selected for subsequent bioassays. In growth kinetics evaluation, parasites counting at 24 h confirmed that compound 3 at this concentration reduce parasites to 50% of the control.
Since the compound showed high cytotoxicity, resulting in a selectivity index of 0.72 which suggests low selectivity of the molecule for the parasites in comparison to human cells, Galleria mellonella model was used to access in vivo toxicity. Results indicate that 3 is not toxic in living complex organisms. The use of in vitro models for toxicity is advanced since live animals represent an ethical challenge; however, no in vitro model can mimic the complexity of a live biological organism. In this sense, this insect model possesses many attractive features as experimental model when compared with mammals, including the fact that G. mellonella larvae are cheaper and easier to maintain, they can be maintained at 37 °C and they are not submitted to ethical restriction. Its great acceptance by the scientific community is evidenced by the increasing number of scientific publications in international journals using this invertebrate for infectious research. Larvae possess an innate immune system that is structurally and functionally similar to that of mammals (Browne et al. 2013), and gastrointestinal and hepatic systems of insect and mammals present similarities and, in this context, the insect fat body is an organ that functions in metabolism and is similar to the liver of mammals (Büyükguzel et al. 2013). Also, there are enzymes, which are involved in drug detoxification, located in the fat body and there are data indicating cytochrome P450 enzymes behave like their counterparts in mammals (Feyereisen, 1999). Ignasiak and Maxwell (2017) reported that the toxicity determined in G. mellonella model correlates well with toxicity in rats and mice.
Chalcone 3 is a potential cationic amphiphilic agent, due to its ring A being substituted by the basic and hydrophilic group, as well as ring B and α,β-unsaturated ketone bridge, two hydrophobic moieties. These structural features can be responsible for the promiscuous disruptor effects on biomembranes. The capacity of the disrupting cell membrane may be considered a primary mechanism of cell death, since membrane integrity is essential for the maintenance of cellular structural integrity, therefore, required for cell survival. The hemolysis assay was performed to investigate if the active compounds have this effect on the trophozoites. The fact that compound 3 did not have a hemolytic effect suggests that it does not affect cell membrane integrity. Taking into account that the trophozoites death was probably not caused by membrane disruption, other mechanisms responsible for the chalcone activity were investigated. The hemolysis assay also provides an indication that the molecule presents compatibility with human erythrocytes, in contrast with the cytotoxic effect observed against the vaginal epithelial cells.
Aminochalcone 3 bearing aniline moiety as ring A, which is a recognized inductor moiety of reactive oxygen species (ROS) overproduction leads to oxidative stress and consequent cell damage (Wang et al. 2016). Compound 3 did not induce ROS accumulation in the trophozoites or the scavenging system of the parasite was efficient, thus indicating that this is not the mechanism of action of this molecule on T. vaginalis. The mode of action of metronidazole and tinidazole is based on production of toxic nitro-radical anions through electron donation by the parasite’s redox pathway (Kulda 1999), and in the search for new alternatives for trichomoniasis, it is a good approach to find compounds with different therapeutic targets, aiming to reduce the possible occurrence of cross-resistance. Our results indicated 3 did not act on trophozoites by mode of action of 5-nitroimidazole drugs, thus the effect of 3 towards unexplored molecular targets can reduce the occurrence of cross-resistance with broadly used drugs.
Neutrophils are the main immune cell recruited on the site of infection by T. vaginalis. As part of the immune response to trichomoniasis, ROS accumulation in these cells is induced by T. vaginalis (Song et al. 2008). For this reason, we compared ROS production in neutrophils induced by trophozoites treated and non-treated with 3. Since aminochalcone 3 activates ROS production in neutrophils stimulated by treated trophozoites, it seems to enhance this important feature of the immune response. Neutrophils incubated only with 3 presented a significant reduction in ROS production at the highest concentration, which may be due to the antioxidant and anti-inflammatory potential already described for chalcone-based compounds (Popoola et al. 2015).
The bioreduction of metronidazole in its active form by T. vaginalis has been related to hydrogenosomal enzyme activity including pyruvate:ferredoxin oxidoreductase (PFOR) and alterations of expression of PFOR are present in metronidazole-resistant isolates (Land et al. 2001). As part of the investigation of the mechanism of death induced by compound 3, we evaluated whether the compound affects the gene expression of this enzyme. As demonstrated by the results, trophozoites treated for 12 and 24 h did not appear to express this gene differently form control group in a significant manner (Table 3).
Chalcones are widely evaluated for their antitumoral potential and it has been demonstrated that many of these molecules target tubulin polymerization (Mirzaei and Emami 2016). Besides the importance of microtubules in the maintenance of cell shape and organization, motility, and cell division, β-tubulin may be specifically involved in T. vaginalis cytoadherence, which is part of the pathogenesis of the parasite (Alderete and Garza 1985). As β-tubulin is an important factor for parasite survival and pathogenesis, the influence of the aminochalcone on β-tubulin gene expression of T. vaginalis was investigated. Our results demonstrate no influence of the molecules on this gene expression of parasites treated with compound 3 for 12 and 24 h (Table 3), therefore this also may not be the molecular target of this compound.
Overall, this study showed the potent activity of 3′-aminochalcone against T. vaginalis, responsible for a neglected parasitic infection with high worldwide incidence and serious health outcomes. The increasing number of drug resistance among patients highlights the urgent necessity of new options to treat trichomoniasis, and chalcones and 3′-aminochalcone, presented high potential to challenge this public health problem.
References
Alderete JF, Garza GE (1985) Specific nature of Trichomonas vaginalis parasitism of host cell surfaces. Infect Immun 50:701–708
Anthwal A, Rajesh UC, Rawat MS, Kushwaha B, Maikhuri JP, Sharma VL, Gupta G, Rawat DS (2014) Novel metronidazole-chalcone conjugates with potential to counter drug resistance in Trichomonas vaginalis. Eur J Med Chem 79:89–94
Böyum A (1968) Isolation of mononuclear cells and granulocytes from human blood. Isolation of monuclear cells by one centrifugation, and of granulocytes by combining centrifugation and sedimentation at 1 g. Scand J Clin Lab Invest Suppl 97:77–89
Browne N, Heelan M, Kavanagh K (2013) An analysis of the structural and functional similarities of insect hemocytes and mammalian phagocytes. Virulence 4(7):597–603
Büyükgüzel E, Büyükgüzel K, Snela M, Erdem M, Radtke K, Ziemnicki K, Adamski Z (2013) Effect of boric acid on antioxidant enzyme activity, lipid peroxidation, and ultrastructure of midgut and fat body of Galleria mellonella. Cell Biol Toxicol 29(2):117–129
Chen M, Zhai L, Christensen SB, Theander TB, Kharazmi A (2001) Inhibition of fumarate reductase in Leishmania major and L. donovani by chalcones. Antimicrob Agents Chemother 45:2023–2029
Cherpes TL, Wiesenfeld HC, Melan MA, Kant JA, Cosentino LA, Meyn LA, Hillier SL (2006) The associations between pelvic inflammatory disease, Trichomonas vaginalis infection, and positive herpes simplex virus type 2 serology. Sex Transm Dis 33:747–752
Cotch MF, Pastorek JG 2nd, Nugent RP, Hillier SL, Gibbs RS, Martin DH, Eschenbach DA, Edelman R, Carey JC, Regan JA, Krohn MA, Klebanoff MA, Rao AV, Rhoads GG (1997) Trichomonas vaginalis associated with low birth weight and preterm delivery. The Vaginal Infections and Prematurity Study Group. Sex Transm Dis 24(6):353–360
Das M, Manna K (2016) Chalcone scaffold in anticancer armamentarium: a molecular insight. J Toxicol 2016:7651047
Diamond LS (1957) The establishment of various trichomonads of animals and man in axenic cultures. J Parasitol 43:488–490
dos Santos O, de Vargas Rigo G, Frasson AP, Macedo AJ, Tasca T (2015) Optimal reference genes for gene expression normalization in Trichomonas vaginalis. PLoS One 10:e0138331
Feyereisen R (1999) Insect P450 enzymes. Annu Rev Entomol 44:507–533
Gauthier C, Legault J, Girard-Lalancette K, Mshvildadze V, Pichette A (2009) Haemolytic activity, cytotoxicity and membrane cell permeabilization of semi-synthetic and natural lupane- and oleanane-type saponins. Bioorg Med Chem 17:2002–2008
Grodstein F, Goldman MB, Cramer DW (1993) Relation of tubal infertility to history of sexually transmitted diseases. Am J Epidemiol 137:577–584
Ignasiak K, Maxwell A (2017) Galleria mellonella (greater wax moth) larvae as a model for antibiotic susceptibility testing and acute toxicity trials. BMC Res Notes 10(1):428
Iqbal H, Prabhakar V, Sangith A, Chandrika B, Balasubramanian R (2014) Synthesis, anti-inflammatory and antioxidant activity of ring-A-monosubstituted chalcone derivatives. Med Chem Res 23:4383–4394
Karimi-Sales E, Mohaddes G, Alipour MR (2018) Chalcones as putative hepatoprotective agents: preclinical evidence and molecular mechanisms. Pharmacol Res 129:177–187
Kavanagh K, Fallon JP (2010) Galleria mellonella larvae as models for studying fungal virulence. Fungal Biol Rev 24:79–83
Kirkcaldy RD, Augostini P, Asbel LE, Bernstein KT, Kerani RP, Mettenbrink CJ, Pathela P, Schwebke JR, Secor WE, Workowski KA, Davis D, Braxton J, Weinstock HS (2012) Trichomonas vaginalis antimicrobial drug resistance in 6 US cities, STD surveillance network, 2009-2010. Emerg Infect Dis 18:939–943
Kissinger P, Adamski A (2013) Trichomoniasis and HIV interactions: a review. Sex Transm Infect 89:426–433
Kulda J (1999) Trichomonads, hydrogenosomes and drug resistance. Int J Parasitol 29:199–212
Land KM, Clemens DL, Johnson PJ (2001) Loss of multiple hydrogenosomal proteins associated with organelle metabolism and high-level drug resistance in trichomonads. Exp Parasitol 97:102–110
Livak KJ, Schmittgen TD (2001) Analysis of relative gene expression data using real-time quantitative PCR and the 2(−Delta Delta C(T)) method. Methods 25:402–408
Mahapatra DK, Bharti SK, Asati V (2015) Chalcone scaffolds as anti-infective agents: structural and molecular target perspectives. Eur J Med Chem 101:496–524
Mirzaei H, Emami S (2016) Recent advances of cytotoxic chalconoids targeting tubulin polymerization: synthesis and biological activity. Eur J Med Chem 121:610–639
Mosmann T (1983) Rapid colorimetric assay for cellular growth and survival: application to proliferation and cytotoxicity assays. J Immunol Methods 65:55–63
Noël JC, Fayt I, Romero-Munoz MR, Simon P, Engohan-Aloghe C (2010) High prevalence of high-risk human papillomavirus infection among women with Trichomonas vaginalis infection on monolayer cytology. Arch Gynecol Obstet 282:503–505
Popoola OK, Marnewick JL, Rautenbach F, Ameer F, Iwuoha EI, Hussein AA (2015) Inhibition of oxidative stress and skin aging-related enzymes by prenylated chalcones and other flavonoids from Helichrysum teretifolium. Molecules 20:7143–7155
Santos MB, Pinhanelli VC, Garcia MAP, Silva G, Baek SJ, França SC, Fachin AL, Martins M (2017) Antiproliferative and pro-apoptotic activities of 2′- and 4′-aminochalcones against tumor canine cells. Eur J Med Chem 138:884–889
Secor WE, Meites E, Starr MC, Workowski KA (2014) Neglected parasitic infections in the United States: trichomoniasis. Am J Trop Med Hyg 90:800–804
Singh G, Arora A, Mangat SS, Rani S, Kaur H, Goyal K, Sehgal R, Maurya IK, Tewari R, Choquesillo-Lazarte D, Sahoo S, Kaur N (2016) Design, synthesis and biological evaluation of chalconyl blended triazole allied organosilatranes as giardicidal and trichomonacidal agents. Eur J Med Chem 108:287–300
Song HO, Shin MH, Ahn MH, Min DY, Kim YS, Ryu JS (2008) Trichomonas vaginalis: reactive oxygen species mediates caspase-3 dependent apoptosis of human neutrophils. Exp Parasitol 118:59–65
Sutcliffe S, Neace C, Magnuson NS, Reeves R, Alderete JF (2012) Trichomonosis, a common curable STI, and prostate carcinogenesis--a proposed molecular mechanism. PLoS Pathog 8:e1002801
Vieira PB, Tasca T, Secor WE (2017) Challenges and persistent questions in treatment of trichomoniasis. Curr Top Med Chem 17:1249–1265
Viikki M, Pukkala E, Nieminen P, Hakama M (2000) Gynaecological infections as risk determinants of subsequent cervical neoplasia. Acta Oncol 39:71–75
World Health Organisation (2012) Global incidence and prevalence of selected curable sexually transmitted infections – 2008. WHO, Geneva
Wang Y, Gao H, Na XL, Dong SY, Dong HW, Yu J, Jia L, Wu YH (2016) Aniline induces oxidative stress and apoptosis of primary cultured hepatocytes. Int J Environ Res Public Health 13:1188
Zhai L, Blom J, Chen M, Christensen SB, Kharazmi A (1995) The antileishmanial agent licochalcone a interferes with the function of parasite mitochondria. Antimicrob Agents Chemother 39:2742–2748
Zhai L, Chen M, Blom J, Theander TG, Christensen SB, Kharazmi A (1999) The antileishmanial activity of novel oxygenated chalcones and their mechanism of action. J Antimicrob Chemother 43:793–803
Zhuang C, Zhang W, Sheng C, Zhang W, Xing C, Miao Z (2017) Chalcone: a privileged structure in medicinal chemistry. Chem Rev 117:7762–7810
Zeraik ML, Ximenes VF, Regasini LO, Dutra LA, Silva DH, Fonseca LM, Coelho D, Machado SA, Bolzani VS (2012) 4'-Aminochalcones as novel inhibitors of the chlorinating activity of myeloperoxidase. Curr Med Chem 19:5405–5413
Funding
Conselho Nacional de Desenvolvimento Científico e Tecnológico (CNPq/Brazil), Marine Biotechnology Program (A.J.M., RedeMarAtivo, grant 408578/2013–0) and Universal Program (D.S.T., grant 443150/2014–1) supported this study. A.J.M., L.O.R, and T.T. thank CNPq for researcher fellowships (grants 303353/2016–3, 306251/2016–7, 312292/2017–1, respectively).
Author information
Authors and Affiliations
Corresponding author
Ethics declarations
Conflict of interest
The authors declare that they have no conflict of interest.
Additional information
Handling Editor: Julia Walochnik
Publisher’s Note
Springer Nature remains neutral with regard to jurisdictional claims in published maps and institutional affiliations.
Electronic supplementary material
ESM 1
(PDF 1187 kb)
Rights and permissions
About this article
Cite this article
Trein, M.R., Rodrigues e Oliveira, L., Rigo, G.V. et al. Anti-Trichomonas vaginalis activity of chalcone and amino-analogues. Parasitol Res 118, 607–615 (2019). https://doi.org/10.1007/s00436-018-6164-4
Received:
Accepted:
Published:
Issue Date:
DOI: https://doi.org/10.1007/s00436-018-6164-4