Abstract
One side effect of cisplatin, a cytotoxic platinum anticancer drug, is peripheral neuropathy; however, its central nervous system effects remain unclear. We monitored respiratory nerve activity from the C4 ventral root in brainstem and spinal cord preparations from neonatal rats (P0–3) to investigate its central effects. Bath application of 10–100 μM cisplatin for 15–20 min dose-dependently decreased the respiratory rate and increased the amplitude of C4 inspiratory activity. These effects were not reversed after washout. In separate perfusion experiments, cisplatin application to the medulla decreased the respiratory rate, and application to the spinal cord increased the C4 burst amplitude without changing the burst rate. Application of other platinum drugs, carboplatin or oxaliplatin, induced no change of respiratory activity. A membrane potential analysis of respiratory-related neurons in the rostral medulla showed that firing frequencies of action potentials in the burst phase tended to decrease during cisplatin application. In contrast, in inspiratory spinal motor neurons, cisplatin application increased the peak firing frequency of action potentials during the inspiratory burst phase. The increased burst amplitude and decreased respiratory frequency were partially antagonized by riluzole and picrotoxin, respectively. Taken together, cisplatin inhibited respiratory rhythm via medullary inhibitory system activation and enhanced inspiratory motor nerve activity by changing the firing property of motor neurons.
Similar content being viewed by others
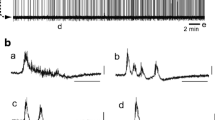
Avoid common mistakes on your manuscript.
Introduction
Although immune checkpoint inhibitors are becoming the mainstream drug therapy for cancer [16], cytotoxic anticancer drugs are still widely used [35]. Cisplatin is a platinum-based anticancer drug that inhibits DNA replication and that has been used in chemotherapy for many cancers since its development [12]. Clinically, cisplatin is used to treat a wide variety of carcinomas, including lung, head and neck, ovarian, and breast cancers [10]. Cisplatin has various side effects, including myelosuppression, gastrointestinal disorders, renal disorders, and neurological disorders [36]. Neuropathy includes peripheral neuropathy and hearing impairment [41]. Peripheral neuropathy is particularly troublesome because once it develops, it is irreversible, causing numbness, pain, hypoesthesia, and mechanical sensitivity in the extremities, leading to a decline in quality of life [17]. The mechanism by which cisplatin causes peripheral neuropathy is unclear, but accumulation of cisplatin in the dorsal root ganglia (DRG), including the primary afferent sensory nerves, is thought to cause peripheral neuropathy. In contrast, cisplatin-induced central nervous system (CNS) disorders include seizures [44] and cognitive decline after chemotherapy, so-called chemobrain [11], but it is unclear whether they are directly caused by cisplatin, and the frequency and mechanism of their occurrence are not clear. In clinical practice, as acute side effects involving the CNS, hiccups, nausea, and vomiting are frequently observed during chemotherapy, especially with cisplatin [24]. It is postulated that the putative central pattern generator of hiccups in the brainstem interacts with the respiratory pattern generator [45]. The neuronal circuit producing vomiting is also located in the medulla and interacts with the respiratory pattern generator [21]. Thus, the clinical question arises as to whether cisplatin may have some acute effects not only on peripheral neuropathy but also on the CNS, especially the lower brainstem including the respiratory center.
The brainstem–spinal cord preparation isolated from newborn rats preserves the neuron networks essential for respiratory rhythm generation and can produce respiratory activity that can be monitored from spinal ventral roots for several hours under in vitro conditions [5, 20, 46]. This preparation has great advantages for pharmacological study of a brainstem function such as the respiratory center because it can be maintained in an anesthetic-free condition with no actual sensory peripheral input, and drugs can be applied by superfusion in known concentrations. In the present study, we presumed that we could detect any significant changes in spinal motor neuron activity of this in vitro preparation if cisplatin affected neuronal activity in the brainstem and spinal cord, and we verified this possibility. We found that cisplatin induced an increase in the amplitude of inspiratory (Insp) motor neuron bursts in the spinal cord and a decrease in respiratory rhythm in the medulla.
Methods
Preparations and solutions
The experimental protocols were approved by the Animal Research Committee of Showa University (approval nos. 09049, 02,022, 03,066) in accordance with Law No. 105 for the care and use of laboratory animals of the Japanese Government. All efforts were made to minimize the number of animals used and their suffering.
Brainstem and spinal cords were isolated from Wistar rats of either sex at postnatal days 0–3 under deep anesthesia with isoflurane. The specimens (108 preparations in total) were cut slightly rostral to the level of the anterior inferior cerebellar artery and caudally at the 6–8th cervical cord and were continuously perfused at a rate of 2.5–3 ml/min with artificial cerebrospinal fluid (ACSF) [46] composed of (in mM) 124 NaCl, 5 KCl, 1.2 KH2PO4, 2.4 CaCl2, 1.3 MgCl2, 26 NaHCO3, and 30 glucose, equilibrated with 95% O2 and 5% CO2, pH 7.4, at 25–26 °C.
Separate perfusion
In some experiments, we performed separate perfusion to investigate the effects of drugs on the medulla and spinal cord, respectively [3, 20, 46]. For this, two thin plastic partitions were placed at the levels of the medulla–spinal cord junction and C2 spinal cord, and perfusates were separately introduced from the spinal cord and medulla sides. Refluxed solutions were ejected from the gap between the two partitions using surface tension to prevent mixing of solutions from the spinal cord and medulla sides.
Drugs and protocols
Cisplatin, carboplatin, and oxaliplatin were used as the platinum-based drugs and were purchased from Tokyo Chemical Industry (TCI), Tokyo, Japan. These drugs were stocked as 100 mM solutions in DMSO and stored at 4 °C and used at final concentrations of up to 100 μM for experiments after being dissolved in ACSF, as described above. We confirmed that this concentration of DMSO had no effect on the neural activity of brainstem-spinal cord preparation. Riluzole was purchased from Sigma-Aldrich (Tokyo, Japan) and was stocked as a 100 mM solution in dimethyl sulfoxide. Picrotoxin was purchased from Wako Pure Medical Co. (Tokyo, Japan) and was stocked as a 10 mM solution including 42% ethanol.
Experiments were divided into four main sections.
-
1.
Effects of cisplatin on C4 inspiratory activity: As there were no similar previous studies on the concentration of cisplatin, and its dose dependence was unknown, we first conducted experiments with four concentration levels of cisplatin: 1, 10, 50, and 100 μM. The perfusion time for all drugs was 15–20 min. Activity after the washout of drugs was followed for a minimum of 30 min. Separate perfusion experiments were performed for 100 μM cisplatin. The other platinum drugs were examined at concentration of 100 μM. This section also included membrane potential recordings of respiratory neurons in the rostral ventrolateral medulla and of inspiratory motor neurons in the spinal cord (see below).
-
2.
Effects of riluzole on cisplatin-induced changes in respiratory activity: We used riluzole because this drug has the property of decreasing the firing frequency of action potentials [7, 8, 26]. Previous studies suggested that riluzole takes more than 10 min to exert a pharmacological effect possibly due to slow diffusion into cells of the medulla and/or spinal cord. Therefore, riluzole was administered for 15 min prior to the application of cisplatin, and then 100 μM cisplatin was applied in the presence of the riluzole. Riluzole was applied at concentrations of 10, 50, and 100 μM based on a previous report in which these concentrations of riluzole alone induced a dose-dependent decrease of the C4 burst rate (i.e., increase in the burst interval) without noticeable change in the burst amplitude [26].
-
3.
Effects of GABAA receptor blocker picrotoxin [31] on cisplatin-induced changes in respiratory activity: A previous study indicated that 10 μM picrotoxin alone induced minor change in the respiratory frequency and also induced a low frequency of seizure-like activity [32]. We administered 100 μM cisplatin for approximately 15 min. After 15 min of washout, 10 μM picrotoxin was applied for 15–20 min. The burst parameters were compared before and after picrotoxin application. In these experiments, 10 μM riluzole was co-applied with cisplatin to prevent the induction of the “huge wave” (see the “Results” section) because measurements were interrupted for more than 10 min if this wave occurred even once.
-
4.
Detailed description of seizure-like activity and the huge wave induced by cisplatin or cisplatin + picrotoxin.
Electrophysiology
Respiratory activity was monitored by the 4th cervical ventral root (C4) activity in most experiments, and by the C5 or C6 activity in some cases. The nerve activity was amplified via a 0.5-Hz high-pass filter (MEG-5200, Nihon Kohden, Tokyo, Japan). We also recorded the membrane potential of pre-inspiratory (Pre-I) or Insp neurons of the rostral ventrolateral medulla at the level of 50–250 μm caudal to the caudal end of the facial nucleus, i.e., in the range from the caudal parafacial respiratory group (pFRG) to the rostral part of the preBötzinger complex (preBötC) [6, 20]. The intracellular recordings were performed using a blind whole-cell patch-clamp method [20, 34], with a high input impedance DC amplifier (CEZ-3100; Nihon Kohden). The electrodes were made by pulling thin-wall borosilicate glass (GC100TF-10, Harvard Apparatus LTD, Kent, UK) while heating. The inner tip diameter was 1.2–2.0 μm and the resistance was 4–8 MΩ. The electrodes were filled with a pipette solution (in mM) of 130 K-gluconate,10 EGTA, 10 HEPES, 2 Na2-ATP, 1 CaCl2, and 1 MgCl2, KOH at pH 7.2–7.3. The electrode tip was filled with 0.5% Lucifer Yellow (lithium salt; Sigma-Aldrich) dissolved in the same solution for the histological analysis of recorded cells. The electrode was inserted into a small area of the ventral medulla oblongata, where the pia membrane was removed by a glass needle. Weak positive pressure was applied to keep the tip of the patch electrode clean (20–40 cmH2O). When the electrode reached the respiratory neuron, it was functionally confirmed by monitoring the extracellular action potentials, and negative pressure was applied for gigaohm (> 1 GΩ) seal formation. The whole-cell configuration is then completed by a single hyperpolarization (amplitude, 0.6 nA; duration, 30 ms) associated with the application of slight negative pressure. A bridge balance circuit was used to compensate for the access resistance, which ranged from 20 to 60 MΩ. In some experiments, Insp motor neurons were recorded in the ventral horn of the C5 or C6 spinal cord with a similar procedure to that described above. In these experiments, the spinal cord of the preparation was transversally cut at the C5 or C6 level, and the ventral root was suctioned for nerve recording and antidromic stimulation. Insp motor neurons were recorded by an approach from the cut surface of the spinal cord. We analyzed the resting membrane potential, input resistance, and peak firing frequency during the burst phase. Hyperpolarizing current pulses (10–100 pA; duration, 0.5 s) were injected around the middle of the interburst period to measure the input resistance. Firing properties of neurons were also examined by applying depolarizing current pulses (10–250 pA) during the interburst period.
After the experiment, specimens were fixed in 4% paraformaldehyde in 0.1 M phosphate-buffered solution (PBS) at 4 °C and stored for histological analysis, and transverse 50-μm slices were cut with a vibrating-blade tissue slicer (PR07, DOSAKA EM CO. Ltd., Osaka, Japan). Labeled neurons were photographed using a fluorescence microscope (BX60; Olympus Optical, Tokyo, Japan). The locations of the cell bodies of recorded neurons were confirmed after staining with NeuroTrace (435/455 blue or 530/615 red fluorescence; Molecular Probes/Invitrogen).
Data analysis
The initial data analysis was performed using the Lab Chart 7 Pro software program (AD Instruments). The burst rate was calculated as an average of 3–5 min. The amplitude and duration time were calculated from the average of 6–10 consecutive cycles. The peak firing frequency of action potentials in respiratory neurons was calculated using Lab Chart 7 Pro. The significance of the values was analyzed by a paired t-test or one-way ANOVA after passing the normality test, followed by a Tukey–Kramer multiple comparisons test at a confidence level of P < 0.05 using the GraphPad Prism 6 software program (GraphPad Software Inc., La Jolla, CA, USA).
Results
Effects of cisplatin on C4 activity
To our knowledge, no previous studies in the relevant literature have described the proper concentrations of cisplatin for in vitro experiments. Therefore, we first examined the effects of a wide range of concentrations (1, 10, 50, 100 μM; 20-min application) of cisplatin on C4 Insp activity. No changes in amplitude, burst duration, or burst rate were observed at 1 μM cisplatin (Fig. 1a). As shown in the examples of Fig. 1b and c and Fig. 2, cisplatin at more than 10 μM increased the amplitude of C4 Insp activity. Table 1 summarizes the dose-dependent changes by cisplatin on C4 Insp activity. Averaged values from five preparations of each concentration showed that a significant increase of burst amplitude was induced at 50 and 100 μM, and the burst rate was significantly reduced at 100 μM (Figs. 1, 2; Table 1). These results suggested that cisplatin caused dose-dependent effects. Typically, the application of 100 μM cisplatin induced increases of the C4 amplitude and burst duration to 312 and 166.7% of control, respectively, and a decrease of burst rate to 43.7% (Fig. 2). These effects were sustained for more than 30 min even after washout. In many cases, the application of 100 μM cisplatin also induced spinal seizure-like activity (see below).
Effects of 100 μM cisplatin on C4 inspiratory activity. a A C4 trace during and after washout of cisplatin. Cisplatin (100 μM) induced an amplitude increase and burst rate decrease of C4 activity. Note that the amplitude increase and burst rate decrease did not recover after the washout of cisplatin. b Faster sweep representations of C4 burst activity; b1 and b2 correspond to b1 and b2 in (a). Note the increase in amplitude and burst duration of C4 inspiratory activity after the application of cisplatin in b2. c Summary of the effects of 100 μM cisplatin on C4 burst activity (n = 5). Control, before the application of cisplatin; 20 min, immediately before washout following 20-min cisplatin application; washout, at 30-min washout of cisplatin. *P < 0.05, **P < 0.01, ***P < 0.001 by one-way ANOVA followed by Tukey–Kramer multiple comparisons test
Effects of separate perfusion of cisplatin
In the next step, separate perfusion was performed to clarify the effects of the application of cisplatin to the medulla or spinal cord (n = 5 in each experiment). When 100 μM cisplatin was applied to the spinal cord (Fig. 3a), the respiratory frequency and duration did not change to a statistically significant extent (101.7 ± 9.9% and 105.4 ± 12.9% of control in cisplatin, respectively), but the amplitude was significantly increased to 351.5 ± 106.9% of control. The increase of amplitude did not recover after washout (Fig. 3a,c). When cisplatin was applied from the medullary side (Fig. 3b), the respiratory frequency decreased to 36.3 ± 7.7% and the duration increased to 162.0 ± 38.8%, whereas the amplitude increased slightly to 112.3 ± 19.4%, but this was not a significant change (Fig. 3b,d). These effects did not recover after washout.
Effects of separate perfusion of 100 μM cisplatin on C4 inspiratory activity. a Example of the application of cisplatin to the spinal cord. Note that the C4 amplitude increased but the burst rate did not decrease. b Example of the application of cisplatin to the medulla. Note that the C4 burst rate decreased with slight increase of burst amplitude. c, d Summary of the effects of the application of 100 μM cisplatin to the spinal cord (c) or the medulla (d) on C4 inspiratory activity (n = 5, respectively). Control, before the application of cisplatin; 20 min, immediately before washout following 20-min cisplatin application; washout, at 30-min washout of cisplatin. *P < 0.05, ***P < 0.001 by a one-way ANOVA followed by Tukey–Kramer multiple comparisons test
Effects of other platinum drugs on C4 activity
We examined the effects of other platinum drugs, carboplatin and oxaliplatin, on C4 activity. We applied 100 μM carboplatin and oxaliplatin (five preparations for each drug). However, the application of these drugs did not induce any change in the burst rate, duration, or amplitude (Fig. 4; Table 2).
Effects of cisplatin on respiratory-related neurons
We performed whole-cell recordings from six Insp neurons and four Pre-I neurons in the rostral ventrolateral medulla distributing from the caudal pFRG to the rostral part of the preBötC. In the example of an Insp neuron shown in Fig. 5, the peak firing frequencies of action potentials in the burst phase were calculated as the average over 10 consecutive bursts, and they decreased to 23.7 Hz (in cisplatin) from 27.6 Hz (in control) (Fig. 5a,b). However, averaged values from six neurons in six preparations did not reach statistical significance (Table 3). Table 3 also shows that the peak firing frequency during the burst phase in Pre-I neurons (n = 4) was significantly reduced. The membrane potentials and input resistances did not change to a statistically significant extent during the application of 100 μM cisplatin (Table 3).
Effects of 100 μM cisplatin on an inspiratory neuron in the ventrolateral medulla. a, b Burst activity during inspiratory phase in control (a) and 16-min cisplatin application (b). aʹ, bʹ Membrane potential responses to depolarizing current pulse in control (aʹ) and at 4 min after washout of cisplatin (bʹ). Note that the firing frequency of the action potential tended to decrease after the application of cisplatin. c Traces of C4 activity (C4), membrane potential trajectory of this inspiratory neuron (Vm), stimulus current (I), and firing frequency of action potentials (Spike rate). Note that the peak firing frequency tended to decrease after the application of cisplatin. d Location of the recorded cell. d1 A low-magnification view and d2 a higher-magnification view of the highlighted square in a. Arrow denotes the Lucifer Yellow–stained cell. The level of the slice was 200 μm caudal to the caudal end of the facial nucleus, corresponding to area between the caudal pFRG and preBötC
Next, we recorded the membrane potential of the Insp motor neurons at C5 or C6. We found that the firing pattern in the burst phase changed to show repeated clusters of higher frequency firings during the application of 100 μM cisplatin (Fig. 6b, b'1). In the example of an Insp motor neuron shown in Fig. 6, the peak firing frequencies of action potentials in the burst phase were calculated as the average over 10 consecutive bursts, and they increased to 33.4 Hz (in cisplatin) from 74.6 Hz (in control). Clustering of action potential firings was also observed in the action potential firings in response to a depolarizing pulse (Fig. 6b'2). The peak firing frequencies of action potentials in the burst phase averaged from six neurons in six preparations significantly increased after the application of cisplatin (Table 3). The time change of the increase in the peak frequency of action potentials (spike rate in Fig. 6c) during the application of 100 μM cisplatin corresponded to the time course of the increase in the amplitude of Insp motor nerve activity. To clarify the relationship between the firing frequency of action potentials in the Insp motor neuron and motor nerve activity, we conducted cycle trigger averaging (Fig. 6d). The peak of the firing frequency appeared to correspond to the peak of C5 activity in the early phase of the Insp burst. Figure 6e shows the relationship between the peak firing frequency in each Insp burst and the amplitude of Insp motor nerve activity, indicating a good correlation between these parameters. These results suggest that the increase in the amplitude of Insp motor nerve activity is caused by the increase in the peak firing frequency of the action potentials of motor neurons. Thus, we hypothesized that drugs reducing the firing frequency of action potentials could antagonize the increase in amplitude induced by cisplatin. Riluzole is known to decrease the firing frequency of action potentials [7, 8, 26]. Therefore, in the next step, we investigated whether riluzole could inhibit the increase of Insp burst amplitude induced by cisplatin.
Effects of 100 μM cisplatin on an inspiratory motor neuron of C5. a, b Burst activity during the inspiratory phase in control (a) and at 7 min after washout following the application of cisplatin (b). aʹ-1, bʹ-1 Faster sweep representation of early inspiratory phase of (a) and (b). aʹ-2, bʹ-2 Membrane potential responses to depolarizing current pulse in control (aʹ-2) and at 16-min cisplatin application (bʹ-2). Note that the firing frequency of the action potential increased after the application of cisplatin. c Traces of C5 activity (C5), membrane potential trajectory of this inspiratory motor neuron (Vm), stimulus current (I), and firing frequency of action potentials (Spike rate). Note that the peak firing frequency increased in association with the application of cisplatin. d Cycle trigger averaging of the firing frequency of action potentials (Spike rate). The firing frequency was averaged with the peak of the C5 activity as the trigger pulse (10 cycles). Left, control; right, after 100 μM cisplatin. Note that the peak of the firing frequency appeared to correspond to the peak of C5 activity. e Plot of the C5 amplitude to the peak firing frequency of the recorded motor neuron. Note the good correlation between these parameters (r = 0.834, P < 0.0001). f Location of the recorded neuron (Lucifer Yellow staining). f1 A low-magnification view and f2 a higher-magnification view of the highlighted square in (a). This cell was in the ventral horn, and the axon could be traced into the C5 nerve root (arrow)
Effects of riluzole on cisplatin-induced C4 amplitude increase
Riluzole was applied at concentrations of 10, 50, and 100 μM by 15-min pretreatment and co-application with 100 μM cisplatin (six preparations in each condition). In the presence of more than 10 μM riluzole, there was a tendency to suppress the decrease in respiratory frequency by cisplatin, although the change was not statistically significant in comparison with the value in the control (Fig. 7a). The increase in the amplitude by cisplatin was significantly suppressed in the presence of more than 50 μM riluzole (Fig. 7b). In addition, it was notable that the administration of riluzole suppressed the seizure-like activity induced by cisplatin (see below).
Effects of riluzole on the changes of C4 burst activity induced by 100 μM cisplatin. Riluzole (10, 50, or 100 μM) was co-applied with cisplatin. Values are calculated as a percentage of those after 20-min cisplatin application in comparison to those immediately before the start of cisplatin application. a The C4 burst interval tended to decrease in the presence of riluzole. b The C4 burst amplitude decreased in the presence of 50 and 100 μM riluzole. The sample number was 6 in each condition. *P < 0.05
Effects of picrotoxin on cisplatin-induced C4 burst rate decrease
The decrease of the respiratory frequency by cisplatin might be caused by excitation of the inhibitory system such as GABAA receptor in the medulla. Because picrotoxin is one of the drugs that blocked inhibitory synaptic effects, we investigated its effects on the cisplatin-induced suppression of respiratory rhythm (n = 5). We applied 100 μM cisplatin for 15–16 min and performed washout for 15 min. After confirmation of a burst rate decrease by cisplatin, 10 μM picrotoxin was applied (Fig. 8) in the presence of 10 μM riluzole (see “Methods” section). The Insp burst interval was shortened to 14.1 ± 2.8 s (in picrotoxin) from 31.6 ± 3.3 s (before picrotoxin) (P < 0.001, n = 5), whereas it was 9.9 ± 1.3 s in control. The C4 amplitude decreased to 68% of that after the application of 100 μM cisplatin (i.e., before picrotoxin) (P < 0.05).
Effects of 10 μM picrotoxin on the changes of C4 burst activity induced by 100 μM cisplatin. a Example of a C4 trace. Cisplatin was applied for 16 min. After 15-min washout of cisplatin, 10 μM picrotoxin was applied for 19 min. This experiment was performed in the presence of 10 μM riluzole to prevent induction of the huge wave (see Figs. 9 and 10). b Faster sweep representation; b1–b4 correspond to b1–b4 in (a). Note that the C4 burst rate increased after the application of picrotoxin
Seizure activity and huge waves
Application of 100 µM cisplatin increased the amplitude and also induced seizure-like activity in many cases (70% of preparations examined) (e.g., Fig. 9b, arrowheads; Fig. 10c2, arrowhead). In addition, in approximately half of the cases to which 100 μM cisplatin was applied, strong depolarization of neurons followed by a temporary cessation of activity (> 10 min) was induced (Fig. 9, arrows; Fig. 10b, arrow). We named this phenomenon the “huge wave.” Respiratory activity was restored again after 10–20 min. This phenomenon was observed only at a concentration of 100 µM cisplatin and not at the lower concentrations. Furthermore, it was not observed with the other platinum drugs. The huge wave appeared to be triggered in conjunction with seizure-like activity (e.g., Fig. 9b, Fig. 10c3). It was observed during the application of 100 μM cisplatin or at its washout but more frequently occurred when cisplatin washout was followed by the application of picrotoxin (Fig. 10). We recorded membrane potentials from 11 respiratory neurons in the rostral medulla when the huge wave appeared. At this time, the membrane of the respiratory neurons depolarized to − 13.9 ± 6.1 mV. At the peak of the huge wave, the input resistance measured in three cells decreased to 28% of the control. The membrane potential gradually repolarized and action potentials were generated again, following which respiratory activity reappeared (Fig. 10b). The generation of the huge wave was never observed in preparations that were treated by riluzole at concentrations of > 10 μM (n = 23), suggesting that riluzole has a strong depressive effect on huge wave generation.
Huge waves induced by cisplatin. a Example of the huge wave (arrow) after the application of cisplatin. C4 activity was silenced after the huge wave and reappeared after 24 min. b Faster sweep representation around the huge wave (black line in a). Note that the seizure-like bursts (arrowheads) appeared before the huge wave
Membrane potential change of a pre-inspiratory neuron in the rostral ventrolateral medulla. Traces of C4 activity (C4), membrane potential trajectory of this neuron (Vm), and stimulus current (I). a, b Consecutive recordings. Application of 10 μM picrotoxin after cisplatin induced the huge wave (b, arrow). c Faster sweep representations of C4 and membrane potential; c1–c4 correspond to c1–c4 in (a) and (b). Cisplatin induced seizure-like bursts of C4 (c2, arrowhead). When the huge wave appeared, the neuron was depolarized and silenced (c3). The burst activity reappeared after 12 min, and the C4 burst activity reappeared after 16 min (c4). d Location of the recorded cell (Lucifer Yellow staining). d1 A low-magnification view and d2 a higher-magnification view of the highlighted square in (d1). The level of the slice was 100 μm caudal to the caudal end of the facial nucleus corresponding to the caudal pFRG
Discussion
We found that cisplatin (10–100 μM) increased the amplitude of Insp C4 burst activity and slowed the respiratory rhythm in the medulla-spinal cord preparation. Moreover, we showed that the separate perfusion of cisplatin to the medulla suppressed the respiratory rhythm without a change of the C4 amplitude, whereas perfusion to the spinal cord increased the amplitude without a change of the respiratory rhythm. To the best of our knowledge, this is the first report indicating that cisplatin suppresses the rhythm of the respiratory center and increases the amplitude of Insp motor neuron activity in the spinal cord.
In addition, we examined the effects of carboplatin and oxaliplatin as other platinum drugs. Along with cisplatin, oxaliplatin, which is often used—especially in the field of gastrointestinal cancer—has been reported to cause peripheral neuropathy [9]. The peripheral neuropathy caused by oxaliplatin is characterized by cold allodynia, which is a cold-induced electric shock pain [43]. This implies that other platinum drugs may have effects similar to those seen with the application of cisplatin. However, neither oxaliplatin nor carboplatin had any effect on respiratory neural activity. These findings indicated that at the very least, the phenomenon observed in the present study was unique to cisplatin. These three drugs contain platinum with different ligands [47]. Therefore, the specific molecular structure of cisplatin may be involved in the unique effects, although the detailed mechanisms are unknown.
Amplitude increase of spinal motor neuron activity by cisplatin
Cisplatin increased the peak firing frequency of Insp spinal motoneurons during bursts. In contrast, this phenomenon was not observed in medullary Insp neurons. There was a good correlation between the peak firing frequency of the motor neurons and Insp motor nerve activity. On the basis of these results, we anticipated that substances that suppressed the firing frequency of action potentials would antagonize the effects of cisplatin on the Insp motor nerve amplitude. Riluzole [26, 30], as one of these substances, partially inhibited the cisplatin-induced amplitude increase in Insp motor nerve activity. It is known that riluzole inhibits fast sodium channels and persistent sodium channels [23, 48] and that it also blocks calcium channels, thereby activating potassium ion channels and G-protein-dependent signaling pathways [7]. Thus, we suggest that the increase in the amplitude of Insp motor nerve activity was caused by a specific action of cisplatin on Insp spinal motor neurons via a change in the characteristics of action potential firings. It is also possible that changes in potassium channel properties are indirectly involved in this change. However, no such amplitude increase has been reported with the administration of various potassium channel blockers [33]. This suggests that cisplatin most likely acts on sodium channels that are specifically expressed in motor neurons (but not medullary interneurons); however, further studies are needed. One previous study reported that oxaliplatin altered voltage-gated Na+ channel kinetics on rat sensory neurons [2] and showed that application of oxaliplatin (250 μM) elicited repetitive firings in sural nerve preparations. This observation might correlate with our result indicating an increase of peak firing frequency induced by cisplatin, although oxaliplatin (100 μM) had no effect on neuronal activity in the brainstem-spinal cord preparation.
Inhibition of respiratory rhythm by cisplatin
The inhibitory effect of cisplatin on the respiratory rhythm was caused by an effect on the medullary rhythm generator, and picrotoxin partially reversed the inhibition. Therefore, the inhibitory effect of cisplatin on respiratory rhythm may be at least partially due to the activation of GABAergic or glycinergic inhibitory neurons in the medulla oblongata [5, 22, 32].
Seizure-like activity and huge wave
Apart from the above effects, cisplatin induced spinal seizure-like activity in many cases. In addition, a huge wave appeared in approximately 50% of the specimens that were treated with 100 μM cisplatin, followed by a loss of respiratory rhythm, and respiratory activity was restored again after 10–20 min. This phenomenon occurred following spinal seizure-like activity. The time course of depolarization and repolarization of the medullary neurons and that of nerve recordings (e.g., C4), were almost parallel, suggesting that this phenomenon occurs over a wide area from the spinal cord to the medulla oblongata. The large decrease observed in the input resistance (28% of control) at the peak (approximately − 14 mV) of the huge wave in recorded neurons might be due to non-selective opening of cation channels. The huge wave was not observed at all in the presence of low concentrations of riluzole (e.g., 10 μM). This effect was not directly related to the antagonistic effect of riluzole on the cisplatin-induced increase in burst amplitude that required riluzole of more than 50 μM. A previous report showed that 10 μM riluzole depressed seizure-like activity induced by pharmacological treatments [25]. As riluzole inhibits sodium channels, glutamate release, and NMDA receptors [23], it is possible that these combined effects blocked seizure-like activity followed by the huge wave. This result is also consistent with the neuroprotective effect of riluzole [49]. Due to its neuroprotective properties, riluzole was approved by the U.S. Food and Drug Administration in 1995 for the treatment and management of amyotrophic lateral sclerosis and is in clinical use [39].
Molecular structure of cisplatin and effects caused
It is not clear at this time why the phenomenon observed in the present study occurs only with cisplatin and not with other platinum drugs. As mentioned above, the effects of cisplatin are thought to be due to its specific molecular structure [29], which is different from other platinum drugs. Cisplatin (cis-diamminedichloroplatinum(II)) is classified as a platinum complex. The central metal of the complex is platinum (Pt), and the ligands are ammine and chloride ions. The ligands form the complex in the cis form. Because cisplatin is a neutral metal complex with zero charge [27], it can easily pass through the cell membrane and enter the cell. As the chloride ion concentration in the cells is lower than that in the plasma, the chloride ions coordinated to the platinum are replaced by water molecules [28]. As hydrogen ions are ionized from water molecules, several platinum complexes reach an equilibrium state, and the hydrolysis products bind to the guanine and adenine N-7 positions of DNA to form a cross-link. The DNA adducts inhibit the replication of cancer cells and exert anticancer effects [14].
The intracellular molecule derived from the unique structure of cisplatin may have acted on sodium channels, causing changes in the firing pattern of motoneurons and an increase in the amplitude of Insp motor output. However, the detailed molecular mechanism needs to be studied further. Cisplatin releases chloride ions after it has penetrated into cells [40], and these chloride ions may have some effects on neural activity. If the equilibrium potential of chloride ions were reversed, the inhibitory postsynaptic potential would become depolarizing and cause excitation. However, the results of our preliminary experiments with a gramicidin perforated patch clamp from medullary neurons did not show this possibility and the effects of GABA did not turn excitatory (unpublished observation). Thus, effects of intracellular free chloride ions are thought to be negligible. However, we do not exclude the possibility that the increase of intracellular chloride ions in interneurons of the spinal cord are involved in the generation of spinal seizure-like activity by cisplatin, and further studies are needed to investigate this point.
Effects of cisplatin on the CNS
Cisplatin-induced neurological damage is mostly peripheral and is less recognized as CNS damage. The reason for this may be the existence of the blood–brain barrier (BBB) [13]. In general, lipophilic molecules with smaller molecular weights (< 15 g/mol) can pass freely through the BBB [41], whereas molecules with larger hydrophilicity [37], such as cisplatin (300 g/mol), are considered less permeable. This suggests that cisplatin has little effect on the CNS. However, cisplatin-induced cognitive impairment has been reported in animal models [18]. In humans, posterior reversible leukoencephalopathy syndrome [19] and cognitive memory impairment (chemobrain) [42] can also occur after chemotherapy with cisplatin. From these reports, it can be inferred that cisplatin has an effect on the CNS. In recent animal experiments, chronic oxaliplatin treatment has been reported to cause changes in cardiorespiratory function, such as an increase in mean arterial pressure and respiratory frequency and a decrease in heart rate and amplitude of phrenic nerve activity, whereas acute oxaliplatin injection had no effect [38]. The same study reported that platinum accumulated in the brain after chronic oxaliplatin treatment. Thus, platinum could actually reach the CNS and have some effects.
The reason anticancer drugs, including platinum drugs, reach the CNS despite the presence of the BBB is thought to be the increased permeability of the BBB. The possible causes include but are not limited to [41]: conditions in which cells are destroyed, such as brain metastasis or brain tumors, combined use of multiple anticancer drugs, repeated exposure, high drug concentrations, administration into the cerebrospinal fluid, and the presence of inflammatory cytokines. In the present experiment, the specimen was the isolated brainstem and spinal cord, so there was no BBB and the drugs could directly reach the CNS.
Limitations
As mentioned above, there are clinical reports of changes in cognitive function, but no actual suppression of respiratory rhythm or enhancement of respiratory output during the administration of cisplatin was confirmed. Because we used brainstem and spinal cord specimens from rats up to 3 days of age, it is necessary to consider the effects of differences in drug concentration per body weight and volume and differences in sensitivity according to the number of days of age.
Assuming a typical Japanese body size of 170 cm in height and 60 kg in weight, the body surface area is approximately 1.69 m2. Assuming that the circulating blood volume is 1/13 of the body weight, the specific gravity of blood is 1.055, and plasma is 55–60% of blood, the circulating plasma volume is estimated to be approximately 2.5 l. In standard lung cancer chemotherapy, cisplatin is often used at a dose of 80 mg/m2 [15], and if this is applied to a typical body size, the concentration of cisplatin in the blood vessels is theoretically calculated to be 180 μM. In clinical practice, the concentration is expected to be lower than this because hydration with extracellular fluid is performed at the same time as the administration of cisplatin. In light of these factors, the concentration of cisplatin in our experiments was speculated to be lower than or almost equal to the concentration used in clinical practice.
It was reported that cisplatin occasionally induced encephalopathy and seizure [44]. Cisplatin frequently induced spinal seizure-like activity in the in vitro preparation used in the present study. However, it is unclear whether this seizure-like activity is caused by the same mechanisms in different preparations. Furthermore, we did not detect the induction of neuronal activity implying hiccups that were observed rather frequently in cisplatin-treated patients [24].
Cisplatin is used in humans to treat pediatric cancers (osteosarcoma, neuroblastoma, germ cell tumor, hepatoblastoma, and others) [1, 50]; however, it is rarely used in neonates. Thus, the effects of cisplatin on the CNS in neonates are unclear, and therefore, the effect of cisplatin sensitivity in immature individuals is unknown. It should also be noted that brain development (especially in the medullary respiratory network) of P0–3 in rats corresponds to that at approximately 25–30 weeks of gestation in humans [4].
The clinical administration of cisplatin in the human body is generally via intravascular injection. As the drugs in the present experiment were applied directly to the medulla and spinal cord in which there was no BBB, it is quite possible that the effect of cisplatin was greater than that in actual clinical practice.
Conclusion
In P0–3 rats, we showed, for the first time, that cisplatin induced respiratory rhythm suppression in the medulla oblongata and increased the amplitude of Insp motoneuron activity in the spinal cord. The detailed mechanism and clinical significance of this effect are still unclear, and further studies are needed.
References
Abou Ali B, Salman M, Ghanem KM, Boulos F, Haidar R, Saghieh S, Akel S, Muwakkit SA, El-Solh H, Saab R, Tamim H, Abboud MR (2019) Clinical prognostic factors and outcome in pediatric osteosarcoma: effect of delay in local control and degree of necrosis in a multidisciplinary setting in Lebanon. J Glob Oncol 5:1–8. https://doi.org/10.1200/JGO.17.00241
Adelsberger H, Quasthoff S, Grosskreutz J, Lepier A, Eckel F, Lersch C (2000) The chemotherapeutic oxaliplatin alters voltage-gated Na(+) channel kinetics on rat sensory neurons. Eur J Pharmacol 406:25–32. https://doi.org/10.1016/s0014-2999(00)00667-1
Arata A, Onimaru H, Homma I (1998) The adrenergic modulation of firings of respiratory rhythm-generating neurons in medulla-spinal cord preparation from newborn rat. Exp Brain Res 119:399–408. https://doi.org/10.1007/s002210050355
Ballanyi K (2004) Neuromodulation of the perinatal respiratory network. Curr Neuropharmacol 2:221–243. https://doi.org/10.2174/1570159043476828
Ballanyi K, Onimaru H, Homma I (1999) Respiratory network function in the isolated brainstem-spinal cord of newborn rats. Prog Neurobiol 59:583–634
Ballanyi K, Ruangkittisakul A, Onimaru H (2009) Opioids prolong and anoxia shortens delay between onset of preinspiratory (pFRG) and inspiratory (preBotC) network bursting in newborn rat brainstems. Pflugers Arch 458:571–587
Bellingham MC (2011) A review of the neural mechanisms of action and clinical efficiency of riluzole in treating amyotrophic lateral sclerosis: what have we learned in the last decade? CNS Neurosci Ther 17:4–31. https://doi.org/10.1111/j.1755-5949.2009.00116.x
Bellingham MC (2013) Pre- and postsynaptic mechanisms underlying inhibition of hypoglossal motor neuron excitability by riluzole. J Neurophysiol 110:1047–1061. https://doi.org/10.1152/jn.00587.2012
Branca JJV, Carrino D, Gulisano M, Ghelardini C, Di Cesare ML, Pacini A (2021) Oxaliplatin-induced neuropathy: genetic and epigenetic profile to better understand how to ameliorate this side effect. Front Mol Biosci 8:643824. https://doi.org/10.3389/fmolb.2021.643824
Brown A, Kumar S, Tchounwou PB (2019) Cisplatin-based chemotherapy of human cancers. J Cancer Sci Ther 11:97
Chiu GS, Maj MA, Rizvi S, Dantzer R, Vichaya EG, Laumet G, Kavelaars A, Heijnen CJ (2017) Pifithrin-mu prevents cisplatin-induced chemobrain by preserving neuronal mitochondrial function. Cancer Res 77:742–752. https://doi.org/10.1158/0008-5472.CAN-16-1817
Dasari S, Tchounwou PB (2014) Cisplatin in cancer therapy: molecular mechanisms of action. Eur J Pharmacol 740:364–378. https://doi.org/10.1016/j.ejphar.2014.07.025
Deeken JF, Loscher W (2007) The blood-brain barrier and cancer: transporters, treatment, and Trojan horses. Clin Cancer Res 13:1663–1674. https://doi.org/10.1158/1078-0432.CCR-06-2854
Fong CW (2016) Platinum anti-cancer drugs: free radical mechanism of Pt-DNA adduct formation and anti-neoplastic effect. Free Radic Biol Med 95:216–229. https://doi.org/10.1016/j.freeradbiomed.2016.03.006
Griesinger F, Korol EE, Kayaniyil S, Varol N, Ebner T, Goring SM (2019) Efficacy and safety of first-line carboplatin-versus cisplatin-based chemotherapy for non-small cell lung cancer: a meta-analysis. Lung Cancer 135:196–204. https://doi.org/10.1016/j.lungcan.2019.07.010
Hargadon KM, Johnson CE, Williams CJ (2018) Immune checkpoint blockade therapy for cancer: an overview of FDA-approved immune checkpoint inhibitors. Int Immunopharmacol 62:29–39. https://doi.org/10.1016/j.intimp.2018.06.001
Hung HW, Liu CY, Chen HF, Chang CC, Chen SC (2021) Impact of chemotherapy-induced peripheral neuropathy on quality of life in patients with advanced lung cancer receiving platinum-based chemotherapy. Int J Environ Res Public Health 18:5677. https://doi.org/10.3390/ijerph18115677
John J, Kinra M, Mudgal J, Viswanatha GL, Nandakumar K (2021) Animal models of chemotherapy-induced cognitive decline in preclinical drug development. Psychopharmacology 238:3025–3053. https://doi.org/10.1007/s00213-021-05977-7
Kabre RS, Kamble KM (2016) Gemcitabine and cisplatin induced posterior reversible encephalopathy syndrome: a case report with review of literature. J Res Pharm Pract 5:297–300. https://doi.org/10.4103/2279-042X.192464
Katsuki S, Ikeda K, Onimaru H, Dohi K, Izumizaki M (2021) Effects of acetylcholine on hypoglossal and C4 nerve activity in brainstem-spinal cord preparations from newborn rat. Respir Physiol Neurobiol 293:103737. https://doi.org/10.1016/j.resp.2021.103737
Kirkwood PA, Ford TW (2004) Do respiratory neurons control female receptive behavior: a suggested role for a medullary central pattern generator? Prog Brain Res 143:105–114. https://doi.org/10.1016/S0079-6123(03)43010-0
Kuwana S, Tsunekawa N, Yanagawa Y, Okada Y, Kuribayashi J, Obata K (2006) Electrophysiological and morphological characteristics of GABAergic respiratory neurons in the mouse pre-Botzinger complex. Eur J Neurosci 23:667–674. https://doi.org/10.1111/j.1460-9568.2006.04591.x
Lamanauskas N, Nistri A (2008) Riluzole blocks persistent Na+ and Ca2+ currents and modulates release of glutamate via presynaptic NMDA receptors on neonatal rat hypoglossal motoneurons in vitro. Eur J Neurosci 27:2501–2514. https://doi.org/10.1111/j.1460-9568.2008.06211.x
Liaw CC, Wang CH, Chang HK, Wang HM, Huang JS, Lin YC, Chen JS (2005) Cisplatin-related hiccups: male predominance, induction by dexamethasone, and protection against nausea and vomiting. J Pain Symptom Manage 30:359–366. https://doi.org/10.1016/j.jpainsymman.2005.08.008
Lin ST, Ohbayashi M, Yamamoto T, Onimaru H, Kogo M (2017) Effects of riluzole on spinal seizure-like activity in the brainstem-spinal cord preparation of newborn rat. Neurosci Res 125:46–53. https://doi.org/10.1016/j.neures.2017.07.002
Lin ST, Onimaru H (2015) Effects of riluzole on respiratory rhythm generation in the brainstem-spinal cord preparation from newborn rat. Neurosci Res 94:28–36. https://doi.org/10.1016/j.neures.2014.12.001
Maheswari KU, Ramachandran T, Rajaji D (2000) Interaction of cisplatin with planar model membranes - dose dependent change in electrical characteristics. Biochim Biophys Acta 1463:230–240. https://doi.org/10.1016/s0005-2736(99)00189-3
Makovec T (2019) Cisplatin and beyond: molecular mechanisms of action and drug resistance development in cancer chemotherapy. Radiol Oncol 53:148–158. https://doi.org/10.2478/raon-2019-0018
Martinho N, Santos TCB, Florindo HF, Silva LC (2018) Cisplatin-membrane interactions and their influence on platinum complexes activity and toxicity. Front Physiol 9:1898. https://doi.org/10.3389/fphys.2018.01898
Miles GB, Dai Y, Brownstone RM (2005) Mechanisms underlying the early phase of spike frequency adaptation in mouse spinal motoneurones. J Physiol 566:519–532. https://doi.org/10.1113/jphysiol.2005.086033
Olsen RW (2006) Picrotoxin-like channel blockers of GABAA receptors. Proc Natl Acad Sci U S A 103:6081–6082. https://doi.org/10.1073/pnas.0601121103
Onimaru H, Arata A, Homma I (1990) Inhibitory synaptic inputs to the respiratory rhythm generator in the medulla isolated from newborn rats. Pflugers Arch 417:425–432
Onimaru H, Ballanyi K, Homma I (2003) Contribution of Ca2+-dependent conductances to membrane potential fluctuations of medullary respiratory neurons of newborn rats in vitro. J Physiol 552:727–741. https://doi.org/10.1113/jphysiol.2003.049312
Onimaru H, Homma I (1992) Whole cell recordings from respiratory neurons in the medulla of brainstem-spinal cord preparations isolated from newborn rats. Pflugers Arch 420:399–406
Opzoomer JW, Sosnowska D, Anstee JE, Spicer JF, Arnold JN (2019) Cytotoxic chemotherapy as an immune stimulus: a molecular perspective on turning up the immunological heat on cancer. Front Immunol 10:1654. https://doi.org/10.3389/fimmu.2019.01654
Oun R, Moussa YE, Wheate NJ (2018) The side effects of platinum-based chemotherapy drugs: a review for chemists. Dalton Trans 47:6645–6653. https://doi.org/10.1039/c8dt00838h
Pardridge WM (2005) The blood-brain barrier: bottleneck in brain drug development. NeuroRx 2:3–14. https://doi.org/10.1602/neurorx.2.1.3
Rahman AA, Stojanovska V, Pilowsky P, Nurgali K (2021) Platinum accumulation in the brain and alteration in the central regulation of cardiovascular and respiratory functions in oxaliplatin-treated rats. Pflugers Arch 473:107–120. https://doi.org/10.1007/s00424-020-02480-4
Rothstein JD (2017) Edaravone: a new drug approved for ALS. Cell 171:725. https://doi.org/10.1016/j.cell.2017.10.011
Salerno M, Yahia D, Dzamitika S, de Vries E, Pereira-Maia E, Garnier-Suillerot A (2009) Impact of intracellular chloride concentration on cisplatin accumulation in sensitive and resistant GLC4 cells. J Biol Inorg Chem 14:123–132. https://doi.org/10.1007/s00775-008-0430-3
Santos N, Ferreira RS, Santos ACD (2020) Overview of cisplatin-induced neurotoxicity and ototoxicity, and the protective agents. Food Chem Toxicol 136:111079. https://doi.org/10.1016/j.fct.2019.111079
Simo M, Rifa-Ros X, Rodriguez-Fornells A, Bruna J (2013) Chemobrain: a systematic review of structural and functional neuroimaging studies. Neurosci Biobehav Rev 37:1311–1321. https://doi.org/10.1016/j.neubiorev.2013.04.015
Staff NP, Cavaletti G, Islam B, Lustberg M, Psimaras D, Tamburin S (2019) Platinum-induced peripheral neurotoxicity: from pathogenesis to treatment. J Peripher Nerv Syst 24(Suppl 2):S26–S39. https://doi.org/10.1111/jns.12335
Steeghs N, de Jongh FE, Sillevis Smitt PA, van den Bent MJ (2003) Cisplatin-induced encephalopathy and seizures. Anticancer Drugs 14:443–446. https://doi.org/10.1097/00001813-200307000-00009
Straus C, Vasilakos K, Wilson RJ, Oshima T, Zelter M, Derenne JP, Similowski T, Whitelaw WA (2003) A phylogenetic hypothesis for the origin of hiccough. BioEssays 25:182–188. https://doi.org/10.1002/bies.10224
Suzue T (1984) Respiratory rhythm generation in the in vitro brain stem-spinal cord preparation of the neonatal rat. J Physiol 354:173–183
Todd RC, Lippard SJ (2009) Inhibition of transcription by platinum antitumor compounds. Metallomics 1:280–291. https://doi.org/10.1039/b907567d
Urbani A, Belluzzi O (2000) Riluzole inhibits the persistent sodium current in mammalian CNS neurons. Eur J Neurosci 12:3567–3574. https://doi.org/10.1046/j.1460-9568.2000.00242.x
Ustun Bezgin S, Uygur KK, Gokdogan C, Elmas C, Goktas G (2019) The effects of riluzole on cisplatin-induced ototoxicity. Int Arch Otorhinolaryngol 23:e267–e275. https://doi.org/10.1055/s-0038-1676654
Yancey A, Harris MS, Egbelakin A, Gilbert J, Pisoni DB, Renbarger J (2012) Risk factors for cisplatin-associated ototoxicity in pediatric oncology patients. Pediatr Blood Cancer 59:144–148. https://doi.org/10.1002/pbc.24138
Funding
This work was partially supported by JSPS KAKENHI (grant no. 19K06946).
Author information
Authors and Affiliations
Contributions
S.O. and H.O. designed and performed the experiments, analyzed data, and wrote the manuscript. M.I. helped to draft the manuscript. All authors read and approved the final manuscript.
Corresponding author
Ethics declarations
Ethics approval and consent to participate
The experimental protocols were approved by the Animal Research Committee of Showa University (approval nos. 09049, 02022, 03066) in accordance with Law No. 105 for the care and use of laboratory animals of the Japanese Government.
Human and animal ethics
This study was performed with animal experiments. All efforts were made to minimize the number of animals used and their suffering.
Consent for publication
All authors consent to publication.
Conflict of interest
The authors declare no competing interests.
Additional information
Publisher's note
Springer Nature remains neutral with regard to jurisdictional claims in published maps and institutional affiliations.
Rights and permissions
Springer Nature or its licensor (e.g. a society or other partner) holds exclusive rights to this article under a publishing agreement with the author(s) or other rightsholder(s); author self-archiving of the accepted manuscript version of this article is solely governed by the terms of such publishing agreement and applicable law.
About this article
Cite this article
Ota, S., Onimaru, H. & Izumizaki, M. Effect of cisplatin on respiratory activity in neonatal rats. Pflugers Arch - Eur J Physiol 475, 233–248 (2023). https://doi.org/10.1007/s00424-022-02762-z
Received:
Revised:
Accepted:
Published:
Issue Date:
DOI: https://doi.org/10.1007/s00424-022-02762-z