Abstract
The present study evaluated whether manganese (Mn) combined with silicon (Si) added to the foliar spray solution favors the physiological aspects, productivity, and protein content of grains in Mn-deficient soybean crops. The experiment was carried out to test four concentrations of Mn (0.00, 0.29, 0.58, and 0.87 g L−1) as chelate with added Si (0.48 g L−1) as potassium silicate and without Si (control), following a 4 × 2 factorial scheme, arranged as randomized block design with five replications. The treatments were applied via foliar in the V6, V8, R1, and R2 phenological stages of the soybean crop. Soybean plants in the control group demonstrated deficiency symptoms such as physiological damage. The beneficials effects of Si on soybean crops it was due by reduction of oxidative stress, due to the increase in the content of the compound antioxidant phenol (9%), causing a decrease in the rate of cell leakage. This mechanism increased the relative chlorophyll index (6%) and the Fv/Fm, thus increasing leaf area, shoot dry mass, productivity (7%), and protein content (7%) in optimal concentration of Mn. The foliar spraying of optimal concentrations of Mn associated with Si is a promising way to improve the efficiency of soybean crops and to meet the nutritional demand for Mn.
Similar content being viewed by others
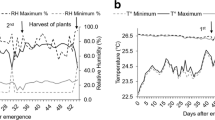
Explore related subjects
Discover the latest articles, news and stories from top researchers in related subjects.Avoid common mistakes on your manuscript.
1 Introduction
Manganese (Mn) deficiency in soybean crops is frequent and intensified in natural alkaline soils or weathered acidic soils, being induced by the anthropogenic action caused by high doses of limestone (Mousavi et al. 2011). The high pH of the soil either decreases the Mn availability or increases complexation caused by the increasing microbial activity and greater adsorption due to the increasing ionic exchange capacity of minerals in the clay fraction of the soil (Kahle et al., 2004). Additionally, in the cultivation of transgenic soy (Roundup Ready®), the application of the herbicide induces a deficiency of the micronutrient in the plant (Nava et al. 2012).
In this scenario of Mn deficiency, the biological functions of this nutrient in the plant are compromised, causing low enzymatic activity and biosynthesis of primary metabolites (Alejandro et al. 2020) and decreasing the content of phenolic compounds, as previously reported for Tanacetum parthenium (Farzadfar et al., 2017). This deficiency aggravates biological damage due to the destabilization and disintegration of photosystem II complexes, which induces a disorderly growth of reactive oxygen species (Kim et al. 2017), consequently causing oxidative stress (Schmidt et al. 2015). Photosynthesis decreases (Schmidt et al. 2016) due to the decrease of leaf chlorophyll content (Papadakis et al., 2007), and the quantum efficiency of photosystem II (Lichtenthaler et al., 2005) also decreases, inducing symptoms such as internerval chlorosis in new leaves (Marschner 2011), consequently decreasing crop yield as well. Additionally, Mn deficiency impairs the nutritional value of the grains, decreasing their protein content (Mann et al. 2002) and compromising quality.
Foliar spraying has been widely used to meet the demand for micronutrients in soybean plants grown in deficient soils, benefiting growth and yield. However, there is a lack of research on the foliar application of micronutrients (Niu et al. 2020), especially regarding the optimal concentration of Mn in the spray solution. To supply Mn to the plant, the most used sources would be manganese sulfate or chelate, having similar agronomic efficiency (Coutinho Neto et al., 2020).
A strategy to reduce the harmful effects of nutritional deficiency would be the use of silicon (Si) applied via a nutrient solution, as previously observed in forages (Buchelt et al., 2020), quinoa (Sales et al. 2021), and sugarcane (Oliveira et al. 2021). In a study of Poaceae, Si was applied through foliar application and reduced the Mn deficiency (Oliveira et al. 2020). It is believed that Si can improve the response of the plant submitted to the foliar application of Mn. This is likely to happen, as one study indicated that isolated foliar applications of Si increased the quantum efficiency of photosystem II and the leaf chlorophyll content of non-Mn deficient plants (Hussain et al. 2021a, b). In addition, Si acts indirectly by increasing the antioxidant defense capacity of the soybean crop (Hussain et al. 2021a, b) and decreasing the production of reactive oxygen species (ROS) (Miao et al. 2010) through the increase in the content of phenolic compounds, as observed for barley (Vega et al. 2019) and arugula crops (Silva et al. 2021).
In this scenario, it is relevant to explore the possible interaction between Mn and Si supplied in the same solution for foliar spraying. A pioneer study with Poaceae using a mixture of Mn in the form of chelate (Mn-EDTA) and potassium silicate stabilized in solution for foliar spraying indicated synergy, improving the response of these plants to Mn supply (Oliveira et al. 2020). This study was carried out on Si-accumulating species (foliar content of Si > 1%), with no reports for non-accumulating species (foliar content of Si < 0.5%) (Mitani and Ma, 2005), including the soybean crop.
Given the importance of Mn foliar spraying in soybean crops and the possible synergy with Si observed in Poaceae but still unknown in plants that do not accumulate the beneficial element, further research is needed to answer the following two hypotheses: i) whether supplying Si via foliar spray associated with manganese supplied as chelate ensures adequate absorption of both elements by soybean plants, indicating possible chemical compatibility, which is a pertinent question due to the risk of the polymerization of Si in solution in the presence of cations (Lindsay 1979), thus hindering their foliar absorption; ii) whether foliar Mn at different concentrations and with or without Si benefits grain quality and crop yield by reducing the stress due to reduced leaf-electrolyte-leakage, thus increasing the chlorophyll content and the quantum efficiency of photosystem II due to the production of antioxidant total phenols.
If both hypotheses are accepted, the addition of Si in the solution should emerge as a new strategy to enhance the effects of foliar fertilization with Mn in soybean cultivation, having wide application, as there are many tropical and subtropical regions with Mn- and Si-deficient soils. To answer these questions, this research aimed at evaluating whether Mn combined with Si in the solution applied via foliar spraying favors the physiological aspects, yield, and protein content of the Mn-deficient soybean grain.
1.1 Material and methods
2 Growth condition and treatments studied
The experiment was carried out in a greenhouse at the São Paulo State University “Júlio de Mesquita Filho,” in Jaboticabal, Brazil. The treatments consisted of four manganese concentrations (0.0, 0.29, 0.58 and 0.87 g L−1) as a chelate (Mn-EDTA: 13%), without Si (control) or with Si concentration of 0.48 g L−1, as potassium silicate (137 g L−1 Si, 164.4 g L−1 K2O), with additives for silicon stability. Mn concentrations were chosen based on literature values (350 g ha−1 of Mn) (Staut, 2007). The Si concentration was determined based on preliminary tests of Mn with Si without visible Si polymerization. The experimental design used was randomized blocks in a 4 × 2 factorial scheme, with five replications. For treatments without addition of Si, foliar spraying with K in the form of KCl was carried out to adjust the concentration of this element since the source of Si contains K. The experimental unit was a 7.0 dm3 polypropylene pot (upper diameter: 16 cm; lower diameter: 11 cm, height: 33 cm) filled with 6 dm3 of sand, previously washed with HCl solution (0.3%, v:v) and deionized water. The soybean Glycine max L. Merrill, cultivar NS 7709, characterized by an early cycle and indeterminate growth habit, was sown in the planting pots, and ten days after emergence (DAE), thinning was performed, keeping three plants per pot.
Deionized water was applied. Ten days after emergence (DAE), nutrient solution by Hoagland and Arnon (1950) was used, modified to Fe concentration (180 µmol L−1) for the Fe-EDDHA source and without addition of Mn to induce Mn deficiency in plants. The pH value of the nutrient solution was adjusted to 5.5 ± 0.5 using HCl (1.0 mol L−1) or NaOH (1.0 mol L−1). The ionic strength of the nutrient solution supplied to the plant was initially set at 15%, but increased to 75% at 20 DAE and maintained until the end of the experiment.
The treatments were applied via foliar spraying to all leaves present on the plants in four defined phenological stages: V6 (five fully developed leaves—plants to 32 DAE); V8 (seven fully developed sheets—plants to 42 DAE); R1 (beginning of flowering, having an open flower at any node of the main stem—plants to 51 DAE) and R2 (beginning of pod formation—plants to 68 DAE), corresponding to the volumes of sprayed solution equal to 5, 12, 18 and 30 mL in the plant grown in the pot, respectively. To ensure only foliar application, the surface of the pot was covered with a layer of cotton and absorbent paper to avoid drift in the substrate and absorption of chemical elements by the roots. The pH value of the solution used for foliar spray was kept at 7.0 for all treatments using HCl (1.0 mol L−1) or NaOH (1.0 mol L−1).
Relative humidity and air temperature were recorded during the experimental period (Fig. 1). During foliar spraying, the air relative humidity was 86, 98, 82 and 85%, in the first, second, third and fourth foliar application, respectively, for providing optimal foliar absorption of Mn and Si.
Temperature (°C) maximum (T max) and minimum (T min) and relative humidity (%) maximum (H max) and minimum (H min) values during the development of the experiment in a greenhouse. The arrows indicate the climatic conditions in the 1st, 2nd, 3rd and 4th foliar application of treatments on soybean plants, collection of plants and grains
3 Analyzed Variables
3.1 Si and Mn accumulation
To determine Si and Mn accumulation, the collected plant material was washed in a detergent solution (0.1%, v:v), hydrochloric acid (0.3%, v:v), and deionized water (Prado 2021).
After drying, the samples were ground in a Willey-type mill, using a 20 mesh sieve, for further chemical analysis. The silicon content was determined by wet digestion, using hydrogen peroxide (H2O2) and sodium hydroxide (NaOH), raising the temperature in an air circulation oven at 120 °C for 4 h to induce the reaction according to the methodology of Kraska and Breitenbeck (2010). The extract was read using a spectrophotometer at 410 nm, by the colorimetry method with hydrochloric acid, oxalic acid and ammonium molybdate (Korndörfer et al. 2004).
The total manganese content was determined following the method described by Bataglia et al. (1983). The Si and Mn accumulations were calculated as the product of dry mass times the element content and expressed as milligrams per plant.
3.2 Relative chlorophyll index
The relative chlorophyll index (IRC) was obtained at 96 DAE, evaluating fully developed younger leaves that received the last foliar spray that were marked when spraying occurred. The estimate of IRC was performed with the aid of the indirect chlorophyll measuring device Opti-sciences® CCM—200 with an average of five readings per plant, in which the indirect values of chlorophyll A and B were obtained, and by the sum, the values of relative index of total chlorophyll.
3.3 Quantum efficiency of photosystem II
The quantum efficiency of photosystem II (Fv / Fm) was obtained, at 99 DAE, from the measurement of chlorophyll fluorescence using a fluorometer (Opti-sciences—Os30P). For this, the sampled region was left in the dark for at least 30 min before the excitation of the one-second red light pulse (Saturation light source: array of red LEDs 660 nm). The following readings F0 (minimum fluorescence for chlorophyll excitation) and Fm (maximal fluorescence for chlorophyll excitation) were obtained. From these parameters, Fv (fluorescence variation was calculated from F0 and Fm), Fv / Fm (variable fluorescence and maximum fluorescence ratio) and Fv / F0 (variable fluorescence and minimum fluorescence ratio) were also obtained. The readings were taken between 6:30 am and 7:30 am on the fourth fully developed leaf of the plant counted from the apex and that received the last foliar application of the treatments.
3.4 Total phenol content
The total phenol content was extracted from 0.1 g of fresh leaves collected at 100 DAE that were transferred to 15 mL falcon tubes covered with aluminum foil, diluted in concentrated methanol in a water bath at 25 °C for 3 h, following the methodology of Singleton and Rossi (1965).
For the colorimetric reaction, 1 mL of the filtered extract was transferred to another 15 mL falcon tube covered with aluminum foil and the volume was made up with 10 mL of water and 0.5 mL of Folin-Ciocauteau 2 N, left to stand for 3 min. After this time, 1.5 ml of 20% sodium carbonate was added and again allowed to react for 2 h. Subsequently, the sample absorbance readings were performed with the aid of a spectrophotometer (765 nm). Control samples were prepared following all mentioned procedures, with the exception of fresh material.
3.5 Relative Cell Leakage Index
Five leaf discs were removed from the fourth fully developed leaf counted from the apex that received the last foliar application at 100 DAE. Subsequently, the leaf discs were submerged in 20 mL of deionized water in a beaker at room temperature for 2 h, as indicated by Dionisio-Sese and Science (1998).
The solution electrical conductivity (EC1) was read using a benchtop conductivity meter (digital meter TDS—3). Soon after measurement, the samples were autoclaved at 121ºC for 20 min, and after cooling, a new reading was performed to obtain the final electrical conductivity (EC2). Cell leakage index was determined as EC1/EC2 × 100.
3.6 Leaf area and dry mass production
The plant shoots (leaves and stems) were harvested and the evaluations were performed 103 DAE. The leaf area was quantified by the LICOR 3100 leaf area integrator device. Then, all plant material (leaves and stems) was washed in a detergent solution (0.1%, v:v), hydrochloric acid (0.3%, v:v), and deionized water (Prado 2021). Subsequently, the plant material was placed in paper bags, properly identified, and dried in an oven with forced ventilation, at 65ºC ± 2, until they presented a constant mass. Then, the plant material was weighed on a precision analytical balance and the dry mass of the aboveground part of the plant was determined.
All physiological and growth analyzes were performed before the senescence period.
3.7 Grain quantity, yield, and protein content
At physiological maturity (133 DAE), soybean pods were collected, manually threshed, and the number of grains per plant counted. Also, grain yield (g per plant) was obtained from mass, with moisture corrected to 13%.
Subsequently, the grains were washed in a detergent solution (0.1%, v:v), hydrochloric acid (0.3%, v:v) and deionized water (Prado 2021) to determine the protein content. The samples were dried, ground, in a Willey type mill, using a 20 mesh sieve, and stored for further chemical analysis. Nitrogen (N) was determined from the sample digestion in a sulfuric acid solution, distillation with a Kjeldahl distiller, and titration with a sulfuric acid solution (Bataglia et al. 1983). The protein content in the grain was calculated by the product of the nitrogen content of the sample and the correction factor (FC = 6.25), as proposed by Quast (1966).
3.8 Statistical analysis
After testing normality (Kolmogorov–Smirnov test) and homogeneity of variance (Shapiro–Wilk test), all data were subjected to analysis of variance by the F test at 1% and 5% probability. Means of fertilization with Mn via the leaves, with and without Si, were compared by Tukey test at 5% probability.
The effects of Mn concentrations were evaluated from the polynomial regression study, and the models were chosen with a significant F test at 5% probability and with the highest values of the coefficients of determination. For statistical analysis, SAS software version 9.1 (Cary, NC, USA) was used. Values were obtained using the SigmaPlot version 14.0 program.
4 Results
Leaf spraying with increased Mn concentration in the solution, in the absence or presence of Si, increased the accumulation of Si in the leaves of soybean plants. The addition of Si to the solution, in relation to its absence, promoted an increase in the accumulation of Si in the leaves of soybean plants at all concentrations of Mn used (Fig. 2a).
The accumulation of Mn in plants depends on the concentrations of Mn and Si in the solution sprayed on the leaves (Fig. 2b). Leaf spraying with increased concentration of Mn in the solution, with or without Si, increased the accumulated Mn in soybean leaves. The Si added to the foliar spray solution promoted a greater accumulation of Mn in soybean leaves at all concentrations used (Fig. 2b).
Foliar spraying with increased Mn concentration in the solution increased the relative index of total soybean chlorophyll, reaching the maximum value for Mn concentrations equal to 0.53 and 0.56 g L−1 with and without Si, respectively (Fig. 3a). Furthermore, the Si added to the solution increased the relative chlorophyll index of soybean when sprayed at Mn concentrations of 0, 0.29, 0.58 and 0.87 g L−1 (Fig. 3a). In addition, Mn applied via the leaves increased the quantum yield of photosystem II (Fv / Fm) of the soybean plant, reaching maximum values with the use of Mn concentrations equal to 0.59 and 0.64 g L−1 with and without Si, respectively (Fig. 3b). The Si added to the solution promoted an increase in the quantum yield of photosystem II (Fv / Fm), especially in foliar sprays at Mn concentrations equal to 0, 0.29, 0.58 and 0.87 g L−1 (Fig. 3b). The Mn applied via the leaves increased the total phenol content of the soybean plant, reaching the maximum for Mn concentrations equal to 0.49 and 0.74 g L−1 with and without Si, respectively (Fig. 3c). The Si added to the solution increased the total phenol content only for foliar sprays at Mn concentrations equal to 0, 0.29 and 0.58 g L−1 (Fig. 3c).
Relative index of total chlorophyll (a), quantum efficiency of photosystem II (PSII) (b), total phenolic content (c) and cellular electrolyte leakage (d) of soybean plants in relation to increased concentrations of manganese (Mn) in the presence (+ Si) and in the absence of silicon (- Si). ** p < 0.01; * p < 0.05 and ns p > 0.05 by the F test. The bars represent the standard error of the mean; n = 5
Furthermore, increasing Mn concentrations in the sprayed solution on plants decreased electrolyte leakage from concentrations of 0.08 and 0.04 g L−1 with and without Si, respectively (Fig. 3d). The effect of Si added to the foliar spray solution reduced cellular electrolyte leakage only for concentrations of 0 and 0.29 g L−1 Mn (Fig. 3d).
Leaf spraying with increased Mn concentration without adding Si to the solution increased the leaf area of soybean plants with linear adjustment, but in the presence of Si in the solution this increase reached a maximum value at the Mn concentration equal to 0.88 g L−1 (Fig. 4a). The addition of Si to the solution resulted in an increase in leaf area at all Mn concentrations studied (0.29 to 0.87 g L−1) (Fig. 4a). The Mn added to the solution increased the shoot dry mass of soybean independently of the Si added to the solution, reaching the maximum value in the foliar spray with Mn concentrations equal to 0.71 and 0.89 g L−1 with and without Si, respectively (Fig. 4b). Furthermore, the addition of Si in the solution increased the shoot dry mass of the soybean plant in the foliar sprays with Mn concentrations equal to 0, 0.29 and 0.58 g L−1 (Fig. 4b).
Leaf-applied Mn increased soybean grain yield independently of added Si, reaching the maximum for Mn concentrations of 0.63 and 0.65 g L−1, with and without Si, respectively (Fig. 5a). The Si added to the solution increased soybean grain yield with foliar sprays at Mn concentrations equal to 0.58 and 0.87 g L−1 (Fig. 5a).
Foliar spraying on the soybean plant with increasing concentrations of Mn without adding Si to the solution promoted a linear increase in the protein content of the grain, but with the addition of Si to the solution this increase reached a maximum value at the concentration equal to 0.65 g L−1 of Mn (Fig. 5b). There was an increase in the protein content of the grain with the addition of Si in the solution, mainly for foliar spraying with Mn at concentrations equal to 0 to 0.58 g L−1 (Fig. 5b).
5 Discussion
Biological damage caused by Mn deficiency was clearly observed in soybean plants in the control treatment, which did not receive Mn, and also in the treatment that received foliar spraying with Si. Manganese is important for activating the enzyme RNA polymerase, which is involved in the synthesis of lipids and proteins and in cell multiplication (Prado 2021), affecting the formation of pigmented membranes (Gong et al. 2010) and decreasing the relative chlorophyll content (Fig. 3a). This nutritional disorder also induced energy loss during electron transfer, as evidenced by the low quantum efficiency of photosystem II (Fig. 3b) due to chloroplast damage. These micronutrient functions are linked to the integrity of the membranes of this organelle due to Mn composing specific proteins of PSII and the enzymatic complex of photo-oxidation of water (Schmidt et al. 2016).
In this scenario, the lack of Mn caused stress to the plant, given the low content of antioxidants, such as phenolic compounds (Fig. 3c), as the biosynthesis of this secondary metabolite is compromised, which may have contributed to increase the rate of electrolyte leakage in the cells (Fig. 3d). This fact is caused by the inhibition of the Hill reaction, which can lead to the formation of reactive oxygen species that should be eliminated by the action of the enzyme superoxide dismutase, which can have low activity in Mn deficiency (Reddi et al., 2009) compromising the detoxification of cells. These failing mechanisms hamper the subsequent reactions, such as photophosphorylation and CO2 fixation (Kirkby and Römheld, 2004), thus impairing plant growth by decreasing the leaf area index (Fig. 4a), shoot dry mass (Fig. 4b) and, consequently, the grain yield of the soybean crop (Fig. 5). These results in the control plants indicate that Mn-deficient soybean plants are sensitive to oxidative stress that induces physiological damage (Farzadfar et al., 2017).
The biological damage caused by Mn deficiency without added Si induced symptoms in the soybean leaves such as sprouts with a light green color and more accentuated chlorosis between the midrib, a fact already highlighted by other authors (Epstein and Bloom 2004; Marschner 2011; Prado 2021). The presence of Mn-deficient leaf tips (Control: 0 Mn + Si and -Si) (Fig. 6a) is rarely reported and may be indicative of toxic urea accumulation, probably due to low urease activity (Park and Hausinger 1996). This symptom can occur during the main process of N re-assimilation where arginine is hydrolyzed by arginase, forming urea (Siddappa and Marathe 2020). According to the authors, the urea cycle contains Mn-dependent enzymes responsible for the reaction that produces ammonia, which is quickly incorporated into carbon skeletons, forming new amino acids. Thus, it is evident that, by decreasing urease activity, the lack of Mn (Park and Hausinger 1996) increases the biological damage to the plant. In addition, we can see the benefits of applying Mn to soybean plants (Fig. 6b).
We showed for the first time that Mn foliar spraying together with Si increased the accumulation of Mn in the plant, indicating a promising practice to improve the nutritional status of the soybean crop (Fig. 2a). Given this result, it is possible to increase the efficiency of foliar fertilization with Mn in soybean crop due to synergy with Si in the solution. An important practical implication is the possibility of decreasing the concentration of Mn applied via the leaves while maintaining the benefits of Mn in the physiology and production of the soybean crop. This may reflect on the cost of Mn foliar nutrition in soybean crops and on sustainability, as the extra benefits of Si in mitigating abiotic and biotic stresses that can occur in the crop, especially in field conditions are known. In view of the feasibility of using Mn and Si in soybean crops, the use of Si in agriculture should be increased. In areas with commercial cultivation and research studies predominate with the use of Si in Si-accumulating species (poaceae) and with our promising results in non-accumulating plants (soybeans), further research is needed in this group of plants to fundamentalize its use.
Although this species is classified as non-accumulative (foliar content of Si < 0.5%) (Ma and Takahashi 2002; Mitani and Ma 2005), given the low absorption efficiency of Si transporters (Lsi 1 and Lsi 2) (Ma and Yamaji 2015). Optimum Si uptake via foliar spray on non-accumulating plants is known, but only when Si is supplied alone, seen in soybean (Felisberto et al. 2020) and peanut (Fernandes et al. 2021) plants.
The excellent foliar uptake of Si by the soybean plant may have resulted from the quality of the sprayed solution since no unwanted reactions (polymerization) were observed for Si and Mn in solution (Fig. 2). This finding is probably due to the source of Mn-EDTA chelate used, where pairs of electrons from the Mn ion present coordinated bonds to form a complex with the hexadentate EDTA organic ligand, thus avoiding a reaction that could promote the polymerization of Si. The chelated Si-Mn source is efficient and frequently used in foliar sprays on annual crops (Neocleous et al. 2020). Mn applied together with Si was also efficient for providing both elements to Poaceae (Oliveira et al. 2020). Therefore, the first hypothesis can be accepted, as Si associated with Mn applied via foliar spray ensured that both elements were adequately absorbed by soybean leaves, indicating compatibility considering the concentrations and sources of the elements studied.
This feasibility of mixing Si and Mn is scientifically robust, as it was proven that the increased Mn absorption, with or without Si in the solution, effectively induced physiological improvements by decreasing oxidative stress and increasing the quantum efficiency of photosystem II (Fig. 3a), caused by the decreased electrolyte leakage (Fig. 3d) while favoring biometric variables such as leaf area (Fig. 4a), shoot dry matter production (Fig. 4b), and grain yield (Fig. 5a).
We evidenced that Si and Mn added in the solution clearly improved grain yield and protein content of the soybean crop due to the increasing relative chlorophyll index and quantum efficiency of photosystem II, for all Mn concentrations studied. Therefore, two inferences are possible: one would be the effect of Si on control plants and the other on plants that received Mn via foliar. Thus, Si supply benefited plants severely affected by Mn deficiency (control). This fact was also observed by other authors, as Si increases compromised photosynthetic efficiency (Ali et al. 2020) and improves the homogeneous distribution of the micronutrient in the leaf (Hernandez-Apaolaza 2014).
Furthermore, by reducing the apoplastic concentration of Mn in cowpea, Si modifies, decreasing the binding capacity to cell wall cations (Horst et al. 1999) increasing the cell availability of Mn for use in plant metabolism. The second finding indicates that the Si added to the solution enhances the plant's response, favoring production and quality due to the physiological improvements mentioned above, especially when the plant receives Mn via foliar spray. Therefore, this synergistic relationship Mn and Si in foliar spraying of soybean crop has a physiological effect of adding the benefits of both elements, which is still little explored in the literature. This lack of research involving the two elements possibly occurred due to the risks of polymerization of this mixture in the same solution, but which was overcome in this research, as discussed above.
Another important effect of Si rarely reported is the increase in the production of antioxidants, such as phenolic compounds, which stand out mainly in plants with Mn deficiency or insufficient foliar spray (0.29 g L−1 Mn). This was probably because Si promotes an increase in the activity of the enzymes peroxidases, polyphenol oxidases and phenylalanine ammonia-lyase, acting in the metabolic route for the synthesis of phenolic compounds (Mendonça et al. 2013). This increase in antioxidant compounds evidenced the benefit of Mn and Si applied via the foliar, as they were responsible for reducing electrolyte leakage (Fig. 3d), especially in a more severe situation of Mn deficiency and, consequently, favoring the growth of plants. Given this evidence, this research revealed that the main strategic mechanism of the soybean plant cultivated with some degree of Mn deficiency was to use Si to strengthen the culture's antioxidant defense system.
The importance of Mn foliar spraying for soybean crop has been confirmed by other authors who have investigated isolated Mn application and reported an increase in crop grain yield (Alt et al. 2018, Gettier et al. 1985, Ohki et al. 1985, Ohki et al. 1987) and grain quality due to increased protein content (Mann et al. 2002). In this research, we discovered the possibility of expanding this soybean response by adding Si to the Mn solution, an important fact for this crop that uses a lot of foliar fertilization with Mn in commercial crops.
We observed that the maximum grain yield of the oilseed occurred for a concentration of Mn close to 0.64 g L−1 with or without Si, in four sprays along the soybean crop cycle. These optimal values for the Mn concentration in the solution are close to the results reported by Gettier et al. (1985) (0.56 g L−1 of Mn), using two sprays, and lower than the value of Mann et al. (2002) (1 g L−1 of Mn), with three sprays on soybean plants. This difference for defining the optimal Mn concentration for the soybean crop may be due to the spraying frequency and timing, as Mann et al. (2002) concentrated the foliar applications in the vegetative phase (V4, V8 and V10) whereas this research favored the vegetative and reproductive phases (V6, V8, R1, R2). It is possible that the higher spraying frequency in different phenological stages may be more efficient in correcting the deficiency (Ohki et al. 1987) given the low phloem mobility of the micronutrient in the soybean crop (Prado 2021).
Another relevant aspect is the quality of soybean crops production, which is defined by the protein content, as part of the production of this oilseed is destined for animal feed to meet the needs of the animal diet and favor the production of animal protein (Marques 2018). This research indicated that Mn applied via foliar spray helped to increase the protein content in soybeans (Fig. 5b), which corroborates the results obtained by Mann et al. (2002) who highlighted the importance of adequate protein contents in grains. This research demonstrated that it is possible to improve the quality of soybean grains by using Mn foliar spray at a concentration of 0.58 g L−1, with addition of Si in solution. The effect of Si in increasing the protein content of the grain it is probably due in part to the favoring of photosynthesis, as there was an increase in the chlorophyll content and in the quantum efficiency of photosystem II and, according to Tcherkez et al. (2020), it is known that protein synthesis increases with photosynthesis through stimulation of translation initiation.
It was very clear from the culture's responses that the results discussed allow us to accept the second hypothesis as well. Thus, the synergism of Mn and Si in foliar spray observed in Poaceae by Oliveira et al. (2020) is confirmed for the first time in an oilseed (non-Si-accumulating) that is, it absorbs Si but in a relatively low amount.
This research proposes a new strategy to meet the demand for Mn in soybean crop, based on foliar fertilization with Mn concentration that can be enhanced with the addition of Si to the solution, favoring the sustainability of the crop caused by the better use of Mn by the plants, to achieve high productivity and grain quality. Such practices can be carried out in crops that are grown in different regions of the world due to their sensitivity to Mn deficiency, especially in soils with low available Mn content.
The future perspective is promising and more research is expected to explore the synergy of Mn and Si via the foliar using new sources of Mn chelate, such as EDDHA or innovative organic complexes and other species with different abilities to uptake Si to maximize productivity associated with quality.
6 Conclusion
Our finding proposes a new strategy to meet the demand for manganese in soybean crops from foliar fertilization at a concentration of 0.68 g L−1 of manganese, which can be enhanced with the addition of silicon (0.48 g L− 1).
We showed that the synergy of manganese and silicon via foliar spray benefits the soybean crop by increasing the antioxidant defense system.
The exploration of this interaction should expand the use of silicon in agriculture and at the same time improve the nutritional efficiency of manganese.
Change history
10 February 2022
The bibliographic citation for author Ian Lucas de Oliveira Rocha has been corrected from a previous version of the article.
References
Alejandro S, Höller S, Meier B, Peiter E. (2020) Manganese in plants: from acquisition to subcellular allocation. Front. Plant Sci. 11. https://doi.org/10.3389/fpls.2020.00300
Ali N, Réthoré E, Yvin JC, Hosseini SA (2020) The regulatory role of silicon in mitigating plant nutritional stresses. Plants 9:1779. https://doi.org/10.3390/plants9121779
Alt D, Ng SJ, Grusenmeyer J, Lindsey LE (2018) Seed yield and quality of transgenic high-oleic and conventional soybean as influenced by foliar manganese application. Crop Sci 58:874–879. https://doi.org/10.2135/cropsci2017.06.0391
Bataglia O, Furlani A, Teixeira J (1983) Métodos de análise química de plantas-Boletim técnico 78. Campinas: Instituto Agronômico de Campinas (IAC); 56. (português)
Buchelt AC, Teixeira GCM, Oliveira KS, Rocha AMS, Prado RM, Caione G (2020) Silicon contribution via nutrient solution in forage plants to mitigate nitrogen, potassium, calcium, magnesium, and sulfur deficiency. J Soil Sci Plant Nutr 20:1532–1548. https://doi.org/10.1007/s42729-020-00245-7
Coutinho Neto AM, Silva GP, Coutinho ELM, Prado RM (2020) Foliar sources of boron and manganese in soybean and zinc plants in corn plants complexed with polyols in nutritional status and in production. Idesia 38:97–105. https://doi.org/10.4067/S0718-34292020000300097
Dionisio-Sese ML, Science ST (1998) Antioxidant responses of rice seedlings to salinity stress. Plant Sci 135:1–9. https://doi.org/10.1016/S0168-9452(98)00025-9
Epstein E, Bloom A (2004) Inorganic Components of Plants. In: Epstein E, Bloom A (eds) Mineral Nutrition of Plants: Principles and Perspectives, 2nd edn. Sinauer Associates, Sunderland, pp 41–68
Farzadfar S, Zarinkamar F, Hojati M (2017) Magnesium and manganese affect photosynthesis, essential oil composition and phenolic compounds of Tanacetum parthenium. Plant Physiol Biochem 112:207–217. https://doi.org/10.1016/j.plaphy.2017.01.002
Felisberto G, Mello Prado R, Oliveira RLL (2020) Are nanosilica, potassium silicate and new soluble sources of silicon effective for silicon foliar application to soybean and rice plants? Silicon. 1-12.https://doi.org/10.1007/s12633-020-00668-y
Fernandes S, Silva GP, Prado RDM, Rossatto DR (2021) Association of root and leaf silicon application decreases the C/Si ratio, increasing carbon gain and dry mass production in peanut plants. Commun Soil Sci Plant Anal 1–9.https://doi.org/10.1080/00103624.2021.1921187
Gettier SW, Martens DC, Brumback TB (1985) Timing of foliar manganese application for correction of manganese deficiency in soybean. Agron J 77:627–630. https://doi.org/10.2134/agronj1985.00021962007700040026x
Gong X, Wang Y, Liu C, Wang S, Zhao X, Zhou M, Li N, Lu Y, Hong F (2010) Effects of manganese deficiency on spectral characteristics and oxygen evolution in maize chloroplasts. Biol Trace Element Res 136:372–382. https://doi.org/10.1007/s12011-009-8549-9
Hernandez-Apaolaza L (2014) Can silicon partially alleviate micronutrient deficiency in plants? A review. Plant 240:447–458. https://doi.org/10.1007/s00425-014-2119-x
Hoagland DR, Arnon DI (1950) The water-culture method for growing plants without soil. Calif Agric Exp Stn Circular 347:1–32
Horst WJ, Fecht M, Naumann A, Wissemeier AH, Maier P (1999) Physiology of manganese toxicity and tolerance in Vigna unguiculata (L.) Walp. J Plant Nutr Soil Sci 162:263–274
Hussain S, Mumtaz M, Manzoor S, Shuxian L, Ahmed I, Skalicky M, Brestic M, Rastogi A, Ulhassan Z, Shafiq I, Allakhverdiev SI, Khurshid H, Yang W, Liu W (2021a) Foliar application of silicon improves growth of soybean by enhancing carbon metabolism under shading conditions. Plant Physiol Biochem 159:43–52. https://doi.org/10.1016/j.plaphy.2020.11.053
Hussain S, Shuxian L, Mumtaz M, Shafiq I, Iqbal N, Brestic M, Shoaib M, Sisi Q, Li W, Mei X, Bing C, Zivcak M, Rastogi A, Skalicky M, Hejnak V, Weiguo L, Wenyu Y (2021a) Foliar application of silicon improves stem strength under low light stress by regulating lignin biosynthesis genes in soybean (Glycine max (L.) Merr.). J. Haz. Mater. 401:123256. https://doi.org/10.1016/j.jhazmat.2020.123256
Kahle M, Kleber M, Jahn R (2004) Retention of dissolved organic matter by phyllosilicate and soil clay fractions in relation to mineral properties. Org Geochem 35:269–276
Kim YH, Khan AL, Waqas M, Lee IJ (2017) Silicon regulates antioxidant activities of crop plants under abiotic-induced oxidative stress: A review. Front Plant Sci 8:510. https://doi.org/10.3389/fpls.2017.00510
Kirkby EA, Römheld, V (2004) Micronutrients in Plant Physiology: Functions, Uptake and Mobility. The International Fertiliser Society, Proceedings 543, 52 p.
Korndörfer GH, Pereira HS, Nolla A (2004) Análise de silício: solo, planta e fertilizante. Boletim Técnico – Universidade Federal de Uberlândia (UFU). 75. (in portuguese)
Kraska JE, Breitenbeck GA (2010) Simple, robust method for quantifying silicon in plant tissue. Commun. Soil Sci. Plant Anal. 41, 2075–2085. https://doi.org/10.1080/00103624.2010.498537
Lichtenthaler HK, Buschmann C, Knapp M (2005) How to correctly determine the different chlorophyll fluorescence parameters and the chlorophyll fluorescence decrease ratio RFd of leaves with the PAM fluorometer. Photosynthetica 43:379–393. https://doi.org/10.1007/s11099-005-0062-6
Lindsay W (1979) Chemical equilibria in soils. Wiley, New York, p 449
Ma J, Takahashi E (2002) Soil, fertilizer, and plant silicon research in Japan. Elsevier. 15–30.
Ma JF, Yamaji N (2015) A cooperative system of silicon transport in plants. Trends Plant Sci 20:435–442. https://doi.org/10.1016/j.tplants.2015.04.007
Mann EN, Resende PMD, Mann RS, Carvalho JG (2002) Effect of manganese application on yield and seed quality of soybean. Pesq Agropec Bras 37:1752–1764. https://doi.org/10.1590/S0100-204X2002001200012
Marques CL (2018) Controle da qualidade na produção de ração animal. Coimbra - Portugal: Escola Superior de Agronomia de Coimbra (Dissertação de Mestrado), 36 p. (in portuguese).
Marschner H (2011) Marschner’s mineral nutrition of higher plants. Elsevier. 650 p.
Mendonça AO, Tavares LC, Brunes AP, Monzón DLR, Villela FA (2013) Acúmulo de silício e compostos fenólicos na parte aérea de plantas de trigo após a adubação silicatada. Biosci. J. 29: 1154–1162 (in portuguese)
Miao BH, Han XG, Zhang WH (2010) The ameliorative effect of silicon on soybean seedlings grown in potassium-deficient medium. Ann Bot 105:967–973. https://doi.org/10.1093/aob/mcq063
Mitani N, Ma JF (2005) Uptake system of silicon in different plant species. J Exp Bot 56:1255–1261. https://doi.org/10.1093/jxb/eri121
Mousavi SR, Shahsavari M, Rezaei M (2011) A general overview on manganese (Mn) importance for crops production. Aust J Basic Appl Sci 5(9):1799–1803
Nava IA, Gonçalves AC, Schwantes D, Strey L, Roweder FA, Sousa RFB (2012) Efeitos da fertilização foliar com manganês em soja transgênica cultivada no inverno manejada com glifosato. Rev. Recur. Rurais. 8. https://doi.org/10.15304/rr.id786. (in portuguese).
Neocleous D, Nikolaou G, Ntatsi G, Savvas D (2020) Impact of chelated or inorganic manganese and zinc applications in closed hydroponic bean crops on growth, yield, photosynthesis, and nutrient uptake. Agronomy 10:881. https://doi.org/10.3390/agronomy10060881
Niu J, Liu C, Huang M, Liu K, Yan D (2020) Effects of foliar fertilization: Review of current status and future perspectives. J Soil Sci Plant Nutr 1-15.https://doi.org/10.1007/s42729-020-00346-3
Ohki K, Boswell FC, Parker MB, Shuman LM, Wilson DO, Ohki K, Boswell FC, Parker MB, Shuman LM, Wilson DO (1987) Foliar manganese application to soybeans. Commun Soil Sci Plant Anal 18:243–253. https://doi.org/10.1080/00103628709367815
Oliveira KS, Prado RP, Guedes VHF (2020) Leaf Spraying of manganese with silicon addition is agronomically viable for corn and Sorghum Plants. J Soil Sci Plant Nutri 20:872–880. https://doi.org/10.1007/s42729-020-00173-6
Oliveira KS, Prado RM, Checchio MV, Gratão PL (2021) Silicon via nutrient solution modulates deficient and sufficient manganese sugar and energy cane antioxidant systems. Sci Rep 11:16900. https://doi.org/10.1038/s41598-021-96427-z
Papadakis IE, Giannakoula A, Therios IN (2007) Mn-induced changes in leaf structure and chloroplast ultrastructure of Citrus volkameriana (L.) plants. J Plant Physiol 164:100–103. https://doi.org/10.1016/j.jplph.2006.04.011
Park lS, Hausinger RP, (1996) Metal ion interactions with urease and ureD-urease apoproteins. Biochemistry 35:5345–5352. https://doi.org/10.1021/bi952894j
Prado RM (2021) Mineral nutrition of tropical plants. Springer. 392.
Quast DG (1966) Determinação e estudo da quantidade e qualidade da proteína do grão de milho (Zea mays L.). Pesq. Agrop. Bras. EMBRAPA. 1:1. 225–232. (in portuguese)
Reddi AR, Jensen LT, Naranuntarat A (2009) The overlapping roles of manganese and Cu/Zn SOD in oxidative stress protection. Free Radic. Biol. Med. 46:154. 10.1016 / j.freeradbiomed.2008.09.032
Sales AC, Campos CNS, de Souza Junior JP et al (2021) Silicon mitigates nutritional stress in quinoa (Chenopodium quinoa Willd.). Sci. Rep. 11:14665. https://doi.org/10.1038/s41598-021-94287-1
Schmidt SB, Persson DP, Powikrowska M, Frydenvang J, Schjoerring JK, Jensen PE, Husted S (2015) Metal binding in photosystem II super-and subcomplexes from barley thylakoids. Plant Physiol 4:1490–1502. https://doi.org/10.1104/pp.15.00559
Schmidt SB, Jensen PE, Husted S (2016) Manganese deficiency in plants: the impact on photosystem II. Trends Plant Sci 21:622–632. https://doi.org/10.1016/j.tplants.2016.03.001
Siddappa S, Marathe GK (2020) What we know about plant arginases? Plant Physiol. Biochem 156:600–610. https://doi.org/10.1016/j.plaphy.2020.10.002
Silva DL, Prado RM, Tenesaca LFL, Silva JLF, Mattiuz B (2021) Silicon attenuates calcium deficiency in rocket plants by increasing the production of non-enzymatic antioxidants compounds. Sci Hortic 285:110169. https://doi.org/10.1016/j.scienta.2021.110169
Singleton VL, Rossi JA (1965) Colorimetry of total phenolics with phosphomolybdic-phosphotungstic acid reagents. Am J Enol Vitic 16:144–158
Staut LA (2007) Adubação foliar com nutrientes na cultura da soja. Embrapa Agropecuária Oeste-Artigo de divulgação na mídia (INFOTECA-E) (in portuguese).
Tcherkez G, Carroll A, Abadie C, Mainguet S, Davanture M, Zivy M (2020) Protein synthesis increases with photosynthesis via the stimulation of translation initiation. Plant Sci 291:110352. https://doi.org/10.1016/j.plantsci.2019.110352
Vega I, Nikolic M, Pontigo S, Godoy K, Mora MLL, Cartes P (2019) Silicon improves the production of high antioxidant or structural phenolic compounds in barley cultivars under aluminum stress. Agronomy 9:388. https://doi.org/10.3390/agronomy9070388
Acknowledgements
This study was financed in part by the Coordenação de Aperfeiçoamento de Pessoal de Nível Superior—Brasil (CAPES)—Finance Code 001.
Author information
Authors and Affiliations
Contributions
Ian Lucas de Oliveira Rocha: wrote the manuscript, with everyone's contribution. Authors. Renato de Mello Prado and Cassio Hamilton Abreu-Junior contributed to project conceptualization and administration. Ian Lucas de Oliveira Rocha, Kamilla Silva Oliveira and Dalila Lopes da Silva contributed to the investigation, data collection and processing. All authors contributed to the manuscript review. Ian Lucas de Oliveira Rocha is responsible for the manuscript as a whole.
Corresponding author
Ethics declarations
Competition of interests
The authors declare no knowledge of either competing financial interests or personal relationships that might have influenced the work reported in this article.
Additional information
Publisher's note
Springer Nature remains neutral with regard to jurisdictional claims in published maps and institutional affiliations.
Rights and permissions
About this article
Cite this article
de Oliveira Rocha, I.L., de Mello Prado, R., Oliveira, K.S. et al. Foliar spraying of Mn with addition of Si increases phenolic compound, photosynthetic efficiency, productivity and the protein content of the soybean crop. J Soil Sci Plant Nutr 22, 1894–1903 (2022). https://doi.org/10.1007/s42729-022-00780-5
Received:
Accepted:
Published:
Issue Date:
DOI: https://doi.org/10.1007/s42729-022-00780-5