Abstract
Nickel (Ni) uptake pattern may be influenced in the presence of lead (Pb) metal ions in the soil and may mediate the Ni dynamics in root and shoot part of spinach. A pot culture experiment was carried out with four levels of each Pb (0, 100, 150, and 300 mg kg−1soil) and Ni (0, 100, 150, and 300 mg kg−1 soil) in spinach to assess the Pb and Ni contamination interactive effect on Ni dynamics in soil. Study revealed that increasing the concentration of Pb and Ni enhanced the concentration of the respective metals in the spinach root and shoot parts. Increasing the levels of Pb (0–300 mg kg−1) had significantly (p = 0.05) reduced the Ni uptake in shoot and root from 0.195 to 0.033 mg pot−1 and from 0.026 to 0.008 mg pot−1, respectively. The metal dynamics ratios, i.e., bioconcentration factor (BCF), translocation factor (TF), transfer efficiency (TE), and crop removal of Ni were also negatively affected by application of Pb. Increasing the Pb levels in soil from 100 to 300 mg kg−1 significantly (p = 0.05) decreases BCF value from 0.113 to 0.108. Under multi-metal toxicity conditions, high level of Pb in the effluent reduced the Ni uptake by spinach and affects the soil health. The findings are very much useful for planning effective effluent management strategies and guidelines to reduce the metal contamination in food crops under peri-urban areas.
Similar content being viewed by others
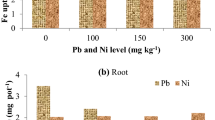
Explore related subjects
Discover the latest articles, news and stories from top researchers in related subjects.Avoid common mistakes on your manuscript.
Introduction
Remediation of heavy metal toxicity is a greatest challenge to produce safe food crops from poor natural resources mainly in developing countries (Qadir et al. 2010; Zhai et al. 2018). However, the increasing global population has also forced to produce more amounts of food grains from the limited land and water resources (Hassan and Aarts 2011; Mahar et al. 2016). Across the globe, agriculture is playing a key role in social and economic development, and also affects the international trade (Saha et al. 2017). In this context, farmers are using poor quality water, i.e., sewage and other contaminated water for mitigating the irrigation demand of the crops mostly in water scarcity regions (Kanwar and Sandha 2000; Ayangbenro and Babalola 2017; Ahsan et al. 2018). Failure of human body organs by consumption of metal contaminated food from metal degraded soils is becoming a common problem in most of the developing countries (Al-Nakshabandi et al. 1997; Qadir et al. 2010; Balkhair and Ashraf 2016). Majority of the heavy metal contaminations are anthropogenic due to mining, smelting, tannery, waste disposal, industries, and vehicle emissions into the environment (Rajendiran et al. 2015). These sources are contaminating the environment, and deteriorate the soil and produce quality (Sachan et al. 2007; Khan et al. 2012). Among the heavy metals, lead (Pb) is very toxic in nature and retention time ranges in soil from 150 to 5000 years (Singh et al. 2016). It reduces mitotic activity (Thounaojam et al. 2012), thereby causing poor growth and yield in plants (Sharma and Dubey 2005; Ramana et al. 2008a, b; Ramana et al. 2009; Dotaniya et al. 2014a, b, c; Bhatia et al. 2017). Poor mental development in children and failure of cardio-vascular system is a common adverse effect in humans due to primary food chain contamination (McLaughlin et al. 1999; Singh et al. 2011). Some of the metals are acting as an essential nutrient element at lower concentration levels and become harmful beyond a critical concentration (Rattan and Goswami 2002). For instance, higher concentrations of nickel (Ni) reduce the RUBISCO activity and decrease the photosynthesis rate in crop plants (Singh et al. 2016). Increasing the concentration of Ni more than 100 mg kg−1 reduced the growth of plants, and chlorosis in leaves is the common symptom (Saha et al. 2017). To toxicity of heavy metals toward phytotoxic concentration, soil enzymatic activities followed the order Cd > Pb > Cr > Ni > Cu > Zn (Saha et al. 2017).
At the present time, most of the industries are located as clusters together to facilitate technology sharing and also reducing the cost of production. In these situations, various heavy metals are discharged from different industrial units into a common effluent channel (Sharma and Dubey 2005; Dotaniya et al. 2014d). This contaminated water is utilized by the farmers in peri-urban areas for food crop cultivation mostly for vegetables production (Khan et al. 2012; Gaur et al. 2014). These heavy metals bring diverse malfunctions in plants, animals, and human systems as well as adversely affected soil microbial process and soil quality (Singh et al. 2016). Studies on the effect of individual metals on living system are well documented by various researchers (Kanwar and Sandha 2000; Sharma and Dubey 2005; Coumar et al. 2016a, b; Raman et al. 2017), but the information on interactive effect of metals, i.e., behavior/dynamics of one metal in the presence of other metal in plant is limited (Hughes 2002; He et al. 2003). Therefore, a study was planned to assess the Pb and Ni metal interactive effect on Ni dynamics in spinach.
Materials and Methods
Physico-Chemical Properties of Experimental Soil
A pot culture experiment was conducted to evaluate the interactive effects of Pb and Ni on Ni dynamics in spinach grown under black cotton soil (Typic Haplustert). Bulk soil was collected from Research farm of ICAR-Indian Institute of Soil Science, Bhopal. The soil was ground and sieved (< 2 mm) and kept in plastic bag for analysis of physico-chemical properties. The experimental soil was clay loam in texture (Bouyoucos hydrometer method 1962). Standard analytical procedures were adopted for the determination of various soil properties, i.e., soil pH and EC (Singh et al. 2005), soil organic C (Walkley and Black 1934), available N (Subbiah and Asija 1956), P (Olsen et al. 1954), K (Hanway and Heidel 1952), and micronutrients (Lindsay and Norvell 1978). Soil pH, EC, organic C, available N, P, and K were 7.82, 0.51 dS m−1, 0.42%, 134, 8.63, and 204 kg ha−1, respectively. The micronutrient concentrations of Zn, Fe, Mn, and Cu were 0.92, 5.42, 5.32, and 1.37 mg kg−1, respectively, in soil. The Pb and Ni metal concentrations were also determined before the application of treatments. Total Pb and Ni were 46.2 and 7.5 mg kg−1, whereas DTPA extractable corresponding metals were 1.23 and 0.69 mg kg−1, respectively.
Pot Experiment
Five kilogram soil was filled in plastic pots (6 kg capacity), and 16 treatment combinations consist with four levels of each Pb (0-absolute control, 100, 150, and 300 mg kg−1 soil) and Ni (0-absolute control, 100, 150, and 300 mg kg−1 soil) were replicated thrice. Three levels of metal application were decided on the basis of data obtained from industrial effluent discharges by various industrial units. Pb and Ni were applied as Pb(NO3)2 (brand MOLYCHEM) and NiCl2.6H2O (brand MERCK), respectively, by dissolving them in required quantity of distilled water and thoroughly mixing. Treated soils were incubated for a month with sufficient moisture to allow equilibrium of metals at field capacity in net house. After 4 months, the soils were again mixed thoroughly, and analyzed for its metal concentrations, soil pH, and EC. No significant difference (p = 0.05) was observed among soil pH, EC, and concentrations of Pb and Ni. The spinach (Spinacia oleracea) crop variety “Palak All Green” was used as a test crop. The recommended doses of fertilizers (N:P2O5:K2O::30:18:18 mg kg−1) were applied for raising the crop. Five seedlings were maintained in each pot throughout the experiment up to 75 days after sowing (DAS). Vegetative matured crop was harvested, and root and shoot portions were separated and stored for metal analysis. Total Pb and Ni concentrations in plant parts were extracted by di-acid (HNO3:HClO4 in the ratio 9:4) digestion and concentration measured by inductively coupled plasma-optical emission spectrometry (ICP-OES) (Model ICP-OES; Perkin Elmer Precisely Optima 2100 DV with 0.001 mg kg−1 detecting limits). On the basis of analytical results, various metal accumulation ratios were computed.
Bioconcentration factor (BCF), defines the metal removal capacity of the plant, was calculated using the expression given by Zhuang et al. (2007)
where Mharvested tissue is a concentration of metal in harvested plant parts (root + shoot) and Msoil are total applied metal levels of respective treatment.
Translocation factor (TF) indicates transfer of metal concentration from root to shoot part, and was quantified by formula proposed by Adesodun et al. (2010)
Translocation efficiency (TE) was calculated with the help of formula described by Meers et al. (2004)
Percent metal removal represented the metal removal capacity of the spinach with respect to metal level. It was calculated as per below formula:
Statistical Analysis
Statistical analysis was done using Factorial Complete Randomized Design with three replications (Gomez and Gomez 1983). Statistical analysis was performed with SAS version 9.3. Two-way analysis of variance (ANOVA) was performed to test the treatment effect, while the significant difference among means of the various treatments was compared with the critical difference (CD) at the 5% (p = 0.05) level of significance.
Results and Discussion
Effect of Pb and Ni levels on Ni Concentration in Shoot and Root
With the increasing level of Ni from 100 to 300 mg Ni kg−1 in soil enhanced the Ni concentration in spinach shoot from 4.37 to 10.05 mg kg−1. Applied level of Ni significantly (p = 0.05) affected the shoot Ni concentration during the course of study (Table 1). On the other hand, increasing the level of Pb from control to highest levels (300 mg kg−1) reduced the Ni concentration in shoots. At 100 mg kg−1 of Pb level, Ni concentration in shoot drastically reduced (36.7%). Furthermore, beyond the Pb level from 150 to 300 mg kg−1, no further reduction of Ni in shoot part of spinach was observed. The negative interaction effect between Pb and Ni might have affected the Ni concentration in the shoot. The minimum Ni concentration was observed in absolute control (0 mg Ni kg−1), and the maximum concentration was recorded in the treatment where the highest level of Ni along with the lowest level of Pb was applied. Increasing the level of Ni in soil also significantly increased the Ni concentration in the roots of spinach crop (Table 2). The root concentration of Ni was significantly increased 11.05, 14.57, and 23.02 mg kg−1 in 100, 150, and 300 mg kg−1 Ni levels, respectively. The concentration of Ni in root was more accumulated when the application of Ni increased from 0 to 100 mg kg−1 soil. The interaction effect between Ni and Pb was observed and found that the increasing level of Pb reduced the Ni concentration in the root. The lowest Ni concentration was measured at the highest level of Pb (300 mg kg−1) with the lowest level of Ni, whereas the maximum concentration was measured in the combination of 300 mg kg−1 Ni and 100 mg kg−1Pb. Nickel is an essential plant nutrient required for completing the life cycle of a plant (Rattan and Goswami 2002). Increasing concentration of beneficial plant nutrient in soil enhanced the concentration of metal in shoot and root parts (Shimada and Ando 1980). Spinach had also accumulated significant amount of Ni in plant biomass. The concentration of metals was higher in the root than in the shoot of the spinach crop, which is also observed in this experiment (Ramana et al. 2009; Alia et al. 2015; Dotaniya et al. 2017c). Lead having greater phytotoxic properties than Ni in spinach crop affects the other metal dynamics in plant parts (Saha et al. 2017). Similar types of results were reported by Chunilall et al. (2004) in spinach during the application of Pb levels. Increasing concentration of metal in root and shoot part could affect metal transfer capacity of the crop as well as cultivar (Ramana et al. 2009). Lead, such as other metallic elements (ME), could be tolerated at low concentration in plant (depending on species and variety); nevertheless, higher concentration will be toxic. Thus, there is a bell shape correlation between toxicity and concentration of ME (Marschner 2011).
Effect of Pb and Ni Levels on Ni Uptake in Plant Parts
Increasing level of Pb from control to 300 mg kg−1 in soil significantly (p = 0.05) decreased the Ni uptake in shoot from 0.195 to 0.062, 0.046, and 0.033 mg pot−1, respectively (Table 3). Increasing concentration of Pb is blocking functional group of enzymes, polynucleotides, and transport system for nutrient ions (Yongsheng et al. 2011). It enhances denaturation and inactivation of enzymatic system in plant (Hassan and Aarts 2011). In higher plants, Pb toxicity that alters plant water relationship causes water stress and wilting, and mediated selectiveness on ions (Ni, Fe, Mn) by roots (Jaishankar et al. 2014). The maximum Ni uptake reduction (68%) was observed due to the application of Pb @ 100 mg kg−1 soil compared to control. Application of Ni enhanced the Ni concentration in shoots of spinach 0.053, 0.074, and 0.125 mg pot−1 in 100, 150, and 300 mg kg−1 soil treatments, respectively. The maximum Ni uptake (0.125 mg pot−1) was observed when the Ni was applied at the highest level (300 mg kg−1) in soil. The interaction effect between Pb and Ni levels revealed that maximum Ni uptake occurred in the treatment that received the highest Ni along with the lowest Pb levels, whereas the minimum Ni uptake was observed with the application of highest level of Pb and lowest level of Ni. The effect of Pb and Ni concentrations had also affected the Ni uptake by root. Similar to Ni uptake by shoots, Ni uptake in roots was also significantly affected by Pb application up to 100 mg kg−1 (Table 4). Thereafter, Ni uptake by root was not significantly (p = 0.05) reduced with increasing levels of Pb. Increasing the Ni levels from control to 100 mg kg−1 soil significantly affected the Ni uptake in root, and further application of higher doses of Ni (100–150 mg kg−1 soil) did not significantly affect the Ni uptake. Effect of increasing levels of Pb and Ni on total Ni uptake by the spinach crop is shown in Table 5. Increasing the level of Pb significantly affected total Ni uptake in spinach. The total Ni uptake was significantly (p = 0.05) reduced with increasing levels of Pb and was observed in the sequence of 300 < 150 < 100 < 0 mg kg−1 Pb. Increasing the level of Ni enhanced the total Ni uptake, due to higher availability of Ni in soil solution and also uptake by roots and shoots of spinach. The highest total Ni uptake (0.146 mg pot−1) was found at the highest level of Ni application. An increment in the photosynthesis rate of plant enhanced due to the application of Ni improved the biomass of shoots and roots (Long et al. 2006). Increasing mineral nutrition (up to 300 mg kg−1 Ni applied level) might have enhanced the growth of the spinach, which resulted in the higher biomass and Ni uptake. Similarly, the enhanced metal uptake by increasing the metal levels had been reported in Gladiolus sp. (Ramana et al. 2008a), tuberose (Ramana et al. 2012), and in marigold and chrysanthemum (Ramana et al. 2008b) grown in Vertisols of central India. Increasing the trace concentration of heavy metals in soil affected the plant growth mostly in the initial phase of germination in crops (Coumar et al. 2016a, b). These metals modified the soil ecology through adverse effects on microbial population and soil enzymatic activities (Casida et al. 1964; Dotaniya et al. 2017a, b; Mandal et al. 2017), and reduced the plant growth and yield parameters (Sharma and Dubey 2005). Decreasing the Ni uptake by application of Pb showed the antagonistic effect that reduces the metal contamination in food chain. It can be a useful strategy in highly polluted soil to minimize the land degradation and improve the soil properties. Such type of interactive effect would show valuable guidelines for minimizing the soil pollution and land degradation (Bhattacharyya et al. 2015).
Effect of Pb and Ni Levels on BCF, Transfer Factor, Translocation Efficiency, and Crop Removal of Ni
The BCF value for the Ni metal was calculated and is described in Table 6. Results revealed that increasing the Ni levels had decreased the BCF value for Ni in all the treatments except at 100 mg kg−1Ni. The control and 150 mg kg−1 level of Ni showed BCF at par, which meant that by increasing the Ni concentration in the soil solution; spinach crop did not take up more Ni like a hyper accumulator plants. Increasing the Pb levels significantly (p = 0.05) decreased the BCF value in all the treatments except control. The value varied 0.162, 0.113, 0.122, and 0.108 in 100, 150, and 300 mg kg−1 Pb in soils, respectively. The interactive effect of all the treatment was also found with respect to BCF value of Ni. It was found that increasing the Ni level control to 100 mg kg−1 enhanced the BCF and again in higher treatment levels; it showed no significant change in BCF at a combination of the highest level of Pb (300 mg kg−1). It indicated that increasing the Pb level hampered the uptake of Ni by spinach crop. The transfer factor refers to the ratio of metal concentration in shoots and root parts of the spinach. Increasing the level of Ni from 100 to 150 mg kg−1 decreased the TF of Ni from 0.444 to 0.388 and again significantly (p = 0.05) increased in 300 mg kg−1 level (Table 7). Increasing the Pb levels significantly (p = 0.05) decreased the TF of Ni in all the treatments from 0.641, 0.479, 0.275, and 0.322 in 0, 100, 150, and 300 mg kg−1 soil treatments, respectively. The interactive effect on TF of Ni revealed that maximum TF factor was reported in the highest level of Ni (300 mg kg−1 soil) with the lowest level of Pb (0 mg kg−1). Increasing levels of Pb from 0 to 300 mg kg−1 significantly (p = 0.05) reduced the TE of Ni in spinach crop (Table 8). The highest reduction was observed in the maximum level of Pb application, i.e., from 35.9 to 20.9%. Ni application enhanced the TE of Ni by 29.4, 26.7, and 30.5% in the treatments 100, 150, and 300 mg kg−1, respectively, compared to control. The interaction effect of Ni and Pb also affected the TE of Ni. Maximum TE was observed at the highest level of Ni with the lowest level of Pb and the minimum in the lowest level of Ni with 100 mg kg−1 Pb level. With the increasing Ni levels up to 100 mg kg−1 enhanced the Ni removal by spinach plants. Beyond that, higher doses of Ni significantly reduced the crop removal of Ni (Table 9). The crop removal was 0.06, 0.05, and 0.04% in 100, 150, and 300 mg kg−1 Ni soil, respectively. The interactive effect of Pb and Ni on crop removal of Ni was also significant (p = 0.05) in many treatment combinations. The maximum Ni removal (0.144%) was with 100 mg kg−1 Ni, when Pb was applied at lowest level, whereas the lowest level of Ni removal (0.014%) was observed in the highest level of both Pb and Ni applied during the growth of the spinach. This mechanism is controlled by the genetic potential of crop plants as well as the nature of the toxic metal. In plants, Ni concentration ranging from 0.1 to 10 mg kg−1 indicates sufficiency for plant nutrition. Most of the essential plant nutrients are readily absorbed and assimilated by plants (Rattan and Goswami 2002); however, this uptake pattern is not obeyed by plants in case of heavy metals. Increasing concentration of metals (Pb and Ni) in soil and subsequently taken up by roots caused growth inhibition and toxicity symptoms (Hall, 2002). By increasing level of Pb, plant develops sequestering mechanism (more accumulation in vacuoles) to reduce the cell damage due to metal toxicity (Sharma and Dubey 2005). In this experiment, poor translocation of Pb and Ni from root to shoot was observed. This might be due to restriction of metal ion transportation from root to shoot part by the active transportation of Pb and Ni ions by the plants (Alia et al. 2015). Lead toxicity mechanisms inhibit plant nutrient uptake kinetics by limiting the functions of enzymes and transportation system related to ion uptake (Jaishankar et al. 2014). All the metal accumulation ratios were negatively performed with increasing concentration of Pb in soil. In metal toxicity conditions, spinach plants accumulated more amount of Pb in root compared to the shoot parts (Jones et al. 1973) and translocated in upper part poorly (Verma and Dubey 2003; Dotaniya et al. 2014d). In most of the cases, Pb ions bind on the root surface of the plant by ion exchange and more uptake by the root part compared to shoot (Jarvis and Leung, 2002; Singh et al. 2016). Nickel is also associated with N metabolism in plants through its influence on enzyme activity; in Ni deficient or lower doses of Ni application, these enhanced in metal accumulation in crop plants (Rattan and Goswami 2002). Higher application of Ni and Pb reduced the plant metabolic process and also metal uptake pattern in plants (Khan et al. 1999). Similar results were also reported in spinach crop (Vallee and Ulmer 1972; Dotaniya et al. 2017c). The plant dynamic parameters of Pb and Ni clearly showed that increasing metal content from contaminant soil to plant was positively supported and reduced the toxicity as self defensive mechanism (Sahu and Dash 2011; Singh et al. 2016). Reducing the metal uptake can be a viable technology to revive the degraded soil and with the help of phytoremediation techniques contaminated soil can also be ameliorated for crop production (Reddy 2003; Dotaniya et al. 2017c).
Conclusions
This waste water contains huge amount of organic and inorganic loads mainly heavy metals, which reduce food quality, soil health, and ecosystem services. This study quantified the interactive effect of Pb and Ni on Ni dynamics for screening suitable metal prevention strategies to improve soil health. Increasing the concentration of Pb in soil reduced the Ni uptake in shoot and root from 0.195 to 0.033 and 0.026 to 0.008 mg pot−1, respectively. It showed antagonistic effect between Ni and Pb concentrations during the course of study. Different metal accumulation ratios indicated negative interaction between the Pb and Ni, and affected the crop growth and metal concentration of respective elements. These types of study enhance the understanding of metal dynamics in spinach crop and helped to formulate the safe management strategies for industrial waste mostly in developing countries.
References
Adesodun JK, Atayese MO, Agbaje TA, Osadiaye BA, Mafe OF, Soretire AA (2010) Phytoremediation potentials of sunflowers (Tithonia diversifolia and Helianthus annuus) for metals in soils contaminated with zinc and lead nitrates. Water, Air, Soil Pollu 207:195–201
Ahsan M, Younis A, Jaskani MJ, Tufail A, Riaz A, Schwinghamer T, Tariq U, Nawaz F (2018) Heavy metal accumulation imparts structural differences in fragrant Rosa species irrigated with marginal quality water. Ecotoxicol Environ Saf 159:240–248
Alia N, Sardar K, Said M, Salma K, Sadia A, Sadaf S, Toqeer A, Miklas S (2015) Toxicity and bioaccumulation of heavy metals in spinach (Spinacia oleracea) grown in a controlled environment. Int J Environ Res Public Health 12(7):7400–7416
Al-Nakshabandi GA, Saqqar MM, Shatanawi MR, Fayyad M, Al-Horani H (1997) Some environmental problems associated with the use of treated wastewater for irrigation in Jordan. Agric Water Manage 34(1):81–94
Ayangbenro AS, Babalola OO (2017) A new strategy for heavy metal polluted environments: a review of microbial biosorbents. Intl J Environ Res Pub Health 14(1):94–98
Balkhair KS, Ashraf MA (2016) Field accumulation risks of heavy metals in soil and vegetable crop irrigated with sewage water in western region of Saudi Arabia. Saudi J Biol Sci 23(1):S32–S44
Bhatia M, Babu RS, Sonawane SH, Gogate PR, Girdhar A, Reddy ER, Pola M (2017) Application of nanoadsorbents for removal of lead from water. Intl J Environ Sci Tech 14(5):1135–1154
Bhattacharyya R, Ghosh BN, Mishra PK, Mandal B, Rao CS, Sarkar D, Das K, Anil KS, Lalitha M, HatiKM Franzluebbers AJ (2015) Soil degradation in India: challenges and potential solutions. Sustain 7:3528–3570. https://doi.org/10.3390/su7043528
Bouyoucos GL (1962) Hydrometer method improved for making particle size analysis of soils. Agron J 54:464
Casida LE, Klein DA, Santoro T (1964) Soil dehydrogenase activity. Soil Sci 98:371–376
Chunilall V, Kindness A, Jonnalagadda SB (2004) Heavy metal uptake by spinach leaves grown on contaminated soils with lead, mercury, cadmium, and nickel. J Environ Sci Health, Part B 39(3):473–481
Coumar MV, Parihar RS, Dwivedi AK, Saha JK, Lakaria BL, Biswas AK, Rajendiran S, Dotaniya ML, Kundu S (2016a) Pigeon pea biochar as a soil amendment to repress copper mobility in soil and its uptake by spinach. BioRes 11(1):1585–1595
Coumar MV, Parihar RS, Dwivedi AK, Saha JK, Rajendiran S, Dotaniya ML, Kundu S (2016b) Impact of pigeon pea biochar on cadmium mobility in soil and transfer rate to leafy vegetable spinach. Environ Monit Assess 188:31
Dotaniya ML, Das H, Meena VD (2014a) Assessment of chromium efficacy on germination, root elongation, and coleoptile growth of wheat (Triticum aestivum L.) at different growth periods. Environ Monit Assess 186:2957–2963
Dotaniya ML, Meena VD, Das H (2014b) Chromium toxicity on seed germination, root elongation and coleoptile growth of pigeon pea (Cajanus cajan). Legume Res 37(2):225–227
Dotaniya ML, Saha JK, Meena VD, Rajendiran S, Coumar MV, Kundu S, Rao AS (2014c) Impact of tannery effluent irrigation on heavy metal build-up in soil and ground water in Kanpur. Agrotechnol 2(4):77
Dotaniya ML, Thakur JK, Meena VD, Jajoria DK, Rathor G (2014d) Chromium pollution: a threat to environment. Agric Rev 35(2):153–157
Dotaniya ML, Meena VD, Rajendiran S, Coumar MV, Saha JK, Kundu S, Patra AK (2017a) Geo-accumulation indices of heavy metals in soil and groundwater of Kanpur, India under long term irrigation of tannery effluent. Bull Environ Conta Toxic J 98(5):706–711
Dotaniya ML, Rajendiran S, Meena VD, Saha JK, Coumar MV, Kundu S, Patra AK (2017b) Influence of chromium contamination on carbon mineralization and enzymatic activities in Vertisol. Agric Res 6(1):91–96
DotaniyaML Rajendiran S, Coumar MV, Meena VD, Saha JK, Kundu S, Kumar A, Patra AK (2017) Interactive effect of cadmium and zinc on chromium uptake in spinach grown on Vertisol of Central India. Intl J Environ Sci Tech. https://doi.org/10.1007/s13762-017-1396-x
Gaur N, Flora G, Yadav M, Tiwari A (2014) A review with recent advancements on bioremediation-based abolition of heavy metals. Environ Sci Process Impacts 16:180–193
Gomez KA, Gomez A (1983) Statistical procedures for agricultural research, 2nd edn. Wiley, New York
Hall JL (2002) Cellular mechanisms for heavy metal detoxification and tolerance. J Exp Bot 53(366):1–11
Hanway JJ, Heidel H (1952) Soil analysis methods as used in Iowa state college soil testing laboratory. Iowa Agric 57:1–31
Hassan Z, Aarts MGM (2011) Opportunities and feasibilities for biotechnological improvement of Zn, Cd or Ni tolerance and accumulation in plants. Env Exp Bot 72:53–63
He B, Yang XE, Ni WZ, Wei YZ, Ye HB (2003) Pb uptake, accumulation, subcellular distribution in a Pb-accumulating ecotype of Sedum alfredii (Hance). J Zhejiang Uni Sci 4(4):474–479
Hughes MF (2002) Arsenic toxicity and potential mechanisms of action. Tox Lett 133:1–16
Jaishankar M, Tseten T, Anbalagan N, Bb Mathew, Beeregowda KN (2014) Toxicity, mechanism and health effects of some heavy metals. Interdiscip Toxicol 7(2):60–72
Jarvis MD, Leung DWM (2002) Chelated lead transport in Pinus radiate: an ultrastructural study. Environ Exp Bot 48:21–32
Jones LHP, Clemant CR, Hopper MJ (1973) Lead uptake from solution by perennial ryegrass and its transport from root to shoot. Plant Soil 38:403–414
Kanwar J, Sandha M (2000) Waste water pollution injury to vegetable crops: a review. Agric Rev 21(2):133–136
Khan NK, Watanabe M, Watanabe Y (1999) Effect of different concentrations of urea with or without nickel addition on spinach (Spinacia oleracea E.) growth under hydroponic culture. Soil Sci Plant Nutri 45(3):569–575
Khan HA, Arif IA, Al Homaidan AA (2012) Distribution pattern of eight heavy metals in the outer and inner tissues of ten commonly used vegetables. Intl J Food Prop 15(6):1212–1219
Lindsay WL, Norvell WA (1978) Development of a DTPA soil test for zinc, iron, manganese and copper. Soil Sci Soc Am J 42:421–448
Long SP, Zhu XG, Naidu SL, Ort DR (2006) Can improvement in photosynthesis increase crop yields. Plant, Cell Environ 29(3):315–330
Mahar A, Wang P, Ali A, Awasthi MK, Lahori AH, Wang Q, Li R, Zhang Z (2016) Challenges and opportunities in the phytoremediation of heavy metals contaminated soils: a review. Ecotox Environ Saf 126:111–121
Mandal A, Thakur JK, Sahu A, Bhattacharjya S, Manna MC, Patra AK (2017) Plant–microbe interaction for the removal of heavy metal from contaminated site. In: Choudhary D, Varma A, Tuteja N (eds) Plant-microbe interaction: an approach to sustainable agriculture. Springer, Singapore
Marschner H (2011) Marschner’s mineral nutrition of higher plants, 3rd edn. Academic Press, Saarbrücken
McLaughlin MJ, Parker DR, Clark JM (1999) Metal and micronutrients-food safety issues. Field Crops Res 60:143–163
Meers E, Hopgood M, Lesage E, Vervaeke P, Tack FMG, Verloo M (2004) Enhanced phytoextraction: in search for EDTA alternatives. Intl J Phyto 6(2):95–109
Olsen SR, Cole CV, Watanable FS, Dean LA (1954) Estimation of available phosphorus in soil by extraction with sodium bicarbonate. Circ USDA 939
Qadir M, Wichelns D, Raschid-Sally L, McCornick PG, Drechsel P, Bahri A, Minhas PS (2010) The challenges of wastewater irrigation in developing countries. Agri Water Manage 97(4):561–568
Rajendiran S, Dotaniya ML, Coumar MV, Panwar NR, Saha JK (2015) Heavy metal polluted soils in India: status and countermeasures. JNKVV Res J 49(3):320–337
Raman NM, Asokan S, Sundari NS, Ramasamy S (2017) Bioremediation of chromium (VI) by Stenotrophomonas maltophilia isolated from tannery effluent. Intl J Environ Sci Tech. https://doi.org/10.1016/j.sjbs.2015.09.023
Ramana S, Biswas AK, Ajay Rao AS (2008a) Phytoextraction of lead by marigold and chrysanthemum. Indian J Plant Physiol 13(3):297–299
Ramana S, Biswas AK, Ajay Singh AB, Rao AS (2008b) Tolerance and bioaccumulation of cadmium and lead by gladiolus. Natl Acad Sci Lett 31(11 & 12):327–332
Ramana S, Biswas AK, Ajay Rao AS (2009) Phytoremediation of cadmium contaminated soils by marigold and chrysanthemum. Natl Acad Sci Lett 32(11&12):333–336
Ramana S, Biswas AK, Ajay Singh AB, Ahirwar NK (2012) Phytoremediation of chromium by tuberose. Natl Acad Sci Lett 35(2):71–73
Rattan RK, Goswami NN (2002) Essential nutrients and their uptake by plants. In: Sekhon GS, Chhonkar PK, Das DK, Goswami NN, Narayanasamy G, Poonia SR, Rattan RK, Sehgal J (eds) Fundamental of soil science. Indian Soc Soil Sci, New Delhi, India, pp 308–332
Reddy VR (2003) Land degradation in India: extent, costs and determinants. Economic and Political Weekly 38:4700–4713
Sachan S, Singh SK, Srivastava PC (2007) Buildup of heavy metals in soil-water-plant continuum as influenced by irrigation with contaminated effluent. J Environ Sci Engin 49(4):293–296
Saha JK, Rajendiran S, Coumar MV, Dotaniya ML, Kundu S, Patra AK (2017) Soil pollution—an emerging threat to agriculture. Springer, Singapore
Sahu HB, Dash S (2011) Land degradation due to Mining in India and its mitigation measures. In Proceedings of the Second International Conference on Environmental Science and Technology, Singapore, 26–28 February 2011
Sharma P, Dubey RS (2005) Lead toxicity in plants. Brazilian J Plant Physiol 17(1):35–52
Shimada N, Ando T (1980) Role of nickel in plant nutrition: effect of nickel on the assimilation of urea by plants. J Soil Sci Plant Nutr 1:493–496
Singh D, Chhonkar PK, Dwivedi BS (2005) Manual on soil, plant and water analysis. Westville, New Delhi
Singh R, Gautam N, Mishra A, Gupta R (2011) Heavy metals and living systems: an overview. Indian J Pharma 43(3):246–253
Singh S, Parihar P, Singh R, Singh VP, Prasad SM (2016) Heavy metal tolerance in plants: role of transcriptomics, proteomics, metabolomics and lonomics. Fron Plant Sci 6:1143–1149
Subbiah BV, Asija GL (1956) A Rapid procedure for the determination of available nitrogen in soils. Curr Sci 25:259–260
Thounaojam TC, Panda P, Mazumdar P, Kumar D, Sharma GD, Sahoo L et al (2012) Excess copper induce doxidative stress and response of antioxidants in rice. Plant Physiol Biochem 53:33–39
Vallee BL, Ulmer DD (1972) Biochemical effect of mercury, cadmium and lead. Ann Rev Biochem 41:91–128
Verma S, Dubey RS (2003) Lead toxicity induces lipid peroxidation and alters the activities of antioxidant enzymes in growing rice plants. Plant Sci 164:645–655
Walkley AJ, Black IA (1934) An examination of the Degtjareff method for determining soil organic matter and a proposed modification of the chromic acid titration method. Soil Sci 37:29–38
Yongsheng W, Qihui L, Qian T (2011) Effect of Pb on growth, accumulation and quality component of tea plant. Proc Engin 18:214–219
Zhai X, Li Z, Huang B, Luo N, Huang M, Zhang Q, Zeng G (2018) Remediation of multiple heavy metal-contaminated soil through the combination of soil washing and in situ immobilization. Sci Total Environ 635:92–99
Zhuang P, Yang QW, Wang HB, Shu WS (2007) Phytoextraction of heavy metals by eight plant species in the field. Water Air Soil Pollu 184:235–242
Acknowledgements
The authors are grateful to Mrs. Seema Sahu and Mr. S. K. Rai, Technical officers, ICAR-IISS, Bhopal, for their valuable help during the analysis of metal and also special thanks due to Dr. Tapan Adhikari, ICAR-IISS, Bhopal, for his technical suggestions during the course of study.
Author information
Authors and Affiliations
Corresponding author
Ethics declarations
Conflict of interest
The authors declare that they have no conflict of interest.
Rights and permissions
About this article
Cite this article
Pipalde, J.S., Dotaniya, M.L. Interactive Effects of Lead and Nickel Contamination on Nickel Mobility Dynamics in Spinach. Int J Environ Res 12, 553–560 (2018). https://doi.org/10.1007/s41742-018-0107-x
Received:
Revised:
Accepted:
Published:
Issue Date:
DOI: https://doi.org/10.1007/s41742-018-0107-x