Abstract
In this study, the influence of drilling parameters on circularity error, tool tip temperature and flank wear is investigated while drilling of Ti–6Al–4V alloy specimens with dissimilar cutting tool materials under dry machining conditions. In addition, optimal control factors for circularity error, tool tip temperature and flank wear have been determined using Taguchi–Grey relational analysis. Rotational speed of the spindle, feed rate and drill bit material are considered as control factors. Numerous drilling experimental runs have been performed employing L27 orthogonal array on a CNC vertical machining centre with 12Ø-mm-diameter holes on 10-mm-thick plates. An infrared thermal camera FLIR E60 is employed to record the temperature at tool chip interface, and Kistler 8793 tri-axial accelerometer is used to get hold of vibration data in real time. Analysis of variance has been carried out to ascertain the most substantial control factors among rotational speed, feed rate and drill bit material and also to establish the effects of the same over circularity error (Cr), temperature (T) and flank wear (VB).
Similar content being viewed by others
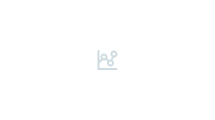
Avoid common mistakes on your manuscript.
1 Introduction
In metal cutting machining, the main issues that affect cutting tool life are relative vibrations between the tool and the workpiece, chip temperature or tool tip temperature along with machining parameters like feed rate (f), spindle rotational speed (N), tool geometry, depth of cut (d), etc. Thus, it turns out to be important for the manufacturing industry to discover the appropriate intensities of cutting process factors for attaining optimum tool life. In metal cutting, heat generation is always a key topic to be studied [1]. As a part of process enhancement, it is certainly beneficial to proactive monitoring of quality directly in the machining process instead of the product; hence, the chances of parts with defects can be reduced or even eliminated.
Drilling is the most significant step in the whole of machining process. With an objective to enhance metal cutting procedures to bring down the cost of parts, the required framework level is used in metal cutting process model [2]. For better performance of a cutter, it is necessary to model the interactions of tool chip interface. Various methodologies are proposed for better cutter performance such as mechanistic, empirical, numerical and analytical. It has been accounted that one-third course of action of material subtraction processes performed in manufacturing firms is drilling operation [3]. For this motive, drilling is indispensable and all things considered adopted material removal procedure. Nevertheless, tool failure might yield in drilling operations as an outcome of tool wear. Consequently, in-process forecasting of tool wear in drilling operations should be explored [4]. An experimental investigation is presented on how the quality of hole is influenced by the parameters of drilling, i.e. feed rate and spindle rotational speed. The assessment included considering the size of hole, circularity error, burrs at the exit and entry and chip developments. Moreover, the quality of hole is investigated by establishing the involvement of parameters of cutting using ANOVA as well as conclusions are derived statistically [5]. Kivak et al. [6] studied the influence of cutting parameters on tool wear and the quality of the hole, i.e., circularity of hole diameter while the drilling Inconel 718 with uncoated and coated drill bits. It was concluded that the quality of the hole and the performance of the drill bit are very poor at higher rotational speed and feed rates. A severe wear of tool amplification is noticed at higher cutting speeds. The multiple responses of arduous interrelationships can be solved using GRA [7]. An advanced optimization was carried out in drilling operation performed on metal matrix composites by altering different cutting parameters with GRA of orthogonal array [8]. The multiple responses obtained by different parameters of drilling operation are optimized in this study such as torque, cutting force and roughness of the surface.
In this work, high-speed steel (HSS) drill, tungsten carbide drill and titanium nitride (TiN) coated are examined for the duration of dry drilling of Ti–6Al–4V alloy under dry machining condition. The drilling experiments were accomplished on 10-mm-thick aerospace quality workpiece material. The experimental runs are performed to probe the prominent outcome of the ideal operation of drilling framework and drilling cutter category on the interface temperature, quality of the hole and vibration factor displacement. By using Taguchi technique in GRA performance characteristics, the available experimental results were optimized.
2 Proposed methodology in the present work
In the present work, difficult-to-cut material like Ti–6Al–4V alloy was used as workpiece material. An attempt is made to investigate the effect and performance of different cutting tool materials in dry drilling operation. Analysis of infrared thermal images and regression analysis carried out in this work will validate the cutting tool performance physiognomies. The performance analyses have shown that the spindle rotational speed and feed rate are the most prevailing factors for the tool wear and cutting temperature, respectively. The confirmation test has certified the validity of the regression model industrialized in the present work. This kind of work is uncommon in the field of machining operations, particularly in drilling. Figure 1 gives the methodology in the present work. The test set-up used for investigation is a 3-axis CNC drilling machine with high-speed 3.5 KW spindle which is capable of drilling up to 18,000 RPM is presented in Fig. 2.
According to methodology proposed, drilling operation is selected to generate holes on most commonly used materials in aircraft and automobile industry such as titanium alloys (Ti–6Al–4V) of dimensions (150 mm × 150 mm × 10 mm). Steps planned to generate experimental data are listed below:
The drilling operation is performed using a 2-flute drill bit on 3-axis high drilling machine as per combinations listed in Table 6.
The workpiece is fixed firmly on the work table as presented in Fig. 2.
An infrared thermal camera FLIR E60 is employed to collect the temperature at tool chip interface in real time.
Every test is initiated with a new drill bit, and temperature at tool chip interface is acquired using thermal images, and machining is stopped after drilling the fifth hole in every test condition for circularity error under coordinate measuring machine (CMM) and tool wear measurement with direct method.
Inspected the drilled holes for roundness measurement under CMM.
Experimental runs are derived through Taguchi L27 orthogonal array, and the same procedure has been adopted to acquire experimental data.
The above listed steps have been repeated to carry out experiments in real time.
Later, ANOVA is employed to determine the most influential process input parameter and the fraction of participation each contributing process parameter on the attributes of quality has been derived. Then, the optimum machining parameters are identified utilizing Taguchi’s S/N ratio charts. The GRA in Taguchi method is adopted to perform multi-objective optimization for process parameters. After that, effect of machining parameters on cutting temperature, circularity error and tool wear is analysed by varying the tool material. Finally, regression analysis is carried out to determine the correlation of cutting temperature, circularity error and tool wear with drilling process parameters.
The multiple responses are assessed by a grey relational grade (GRG). Proportionately, the multiple response optimization will be transformed into an individual relational optimization grade. This proposed methodology gives ample scope for applying the combined Taguchi’s with GRA method for optimization of drilling parameters as a part of drill bit tool performance evaluation. Hence, the current study attempts to present the application of GRA in choosing optimum order for drilling on multi-performance features, specifically the circularity error of the drilled hole, temperature and tool wear. Furthermore, the most substantial factors and the order of prominence of the manageable factors for drilling process have been generated.
2.1 Drill tool and workpiece materials employed in the experiment
The experiments are carried out on Ti–6Al–4V alloy material, and the chemical composition is tabulated in Table 1. Drill bit tools made up of three different types namely high-speed steel, tungsten carbide (WC) and tungsten carbide with physical vapour deposition (PVD) TiN coating are cast-off in the experimental investigation. Specifications of the drill bit tools are shown in Table 2, and their properties are presented in Table 3. Chemical composition of the cutting tool materials is given in Table 4.
2.2 Equipment details and specifications
As part of the investigation, experiments have been carried out on a three axis high-speed CNC drilling machine and its specifications are mentioned as follows: CNC machine is programmed with Win CUT software package which runs on standard G/M codes.
Spindle motor of this machine is rated at 3.5 KW with a maximum spindle rotational speed up to 18,000 rpm. Kistler model 8793 accelerometer was mounted on spindle housing to enable vibration data acquisition for process monitoring in real time. Discussions related to vibration signal acquisition and signal features are intentionally not included in the present paper. Temperature rise during drilling is measured using FLIR E60 thermal camera [9]. Thermal camera is kept at a constant distance, i.e. 1 m away from the machining zone all through the investigation. Emissivity of Ti–6Al–4V is 0.35. The investigational test set-up is as shown in Fig. 2.
2.3 Experiment design
The design of experiments (DOE) facilitates the designers to resolve both the interactive and individual effects of many aspects simultaneously that can disturb the productivity in any design. In addition, DOE also offers a complete understanding of interaction among design elements. The DOE is a well-ordered course of action for scheduling experimentations so that attained data can be explored to yield compelling, nearly exact and impartial conclusions. In the present study, three control factors with three levels are taken into consideration for investigation. Table 5 presents the selected levels of the process parameters for the investigation.
Identifying the objectives of the experiment is the initial points of DOE. In order to acquire maximum information possible, a well-defined investigational design should be used. Full factorial method is used for the design of experiments using Minitab-17 software. In full factorial design, if the number of levels is identical for individual factors, then the possible number of trials, N, can be expressed as:
where L = number of levels for each factor and m = number of factors. The experimentations have been done as per the orthogonal array L27 (33) as presented in Table 6, to estimate the relationship among the input and the output parameters response variables [10].
3 Modelling of output parameters
A statistical tool called Minitab version 17 is used for experimental data statistical analysis. The optimum machining parameters are identified utilizing Taguchi’s S/N ratio charts. ANOVA is employed to determine the most influential process input parameter, and the fraction of participation each contributing process parameter on the attributes of quality has been derived. The GRA in Taguchi method is adopted to perform multi-objective optimization for process parameters. The primary intension of the present work is to minimize the circularity error, temperature and flank wear. Therefore, in view of the quality features, the “smaller the better” has been preferred for the output response characteristics.
The method of least squares is primarily cast-off for the regression analysis to compute the regression coefficients. In addition, factual functional relationship can be established between Yxt and the set of process parameters in a multiple linear regression model. The process variable of linear function will demonstrate the output response variables. The first-order approximating function is presented as follows:
The second-order mode must employ the higher degree polynomial:
One of these models is used in engineering problems for their implementation. Although it is uncertain that the polynomial model will provide exact outcomes for the true functional association over the complete space of the input drilling parameters, nevertheless for a comparatively small zone, they regularly work fairly well.
4 Results and discussion
With an increase in machining time, flank wear, temperature at the tip of the tool and circularity error are measured. The circularity error index (Cr) of the drilled hole represents the quality of the hole and can be determined through experimentation only. Experimental findings obtained concerning circularity error (Cr), temperature (T) and flank swear (VB) are shown in Table 6.
From the observations presented in results in Table 6, it is established that circularity error drops with rise in rotational speed of the spindle from 4200 to 10,600 rpm, but it augments with raise in feed rate from 159 to 400 mm/min.
4.1 Effect of cutting parameters on circularity error (C r)
A GLOBAL performance DEA CMM that is the most suitable for performing metrology-related measurements has been used. The new PC-DMIS adaptive scanning function permits the users of any level to grasp superlative scanning performance with accuracy from 1.5 + L/333 μm. High exactness is ensured in temperature range 16–26 °C. A global performance coordinate measuring machine DEA (07075) is employed to measure the circularity error of drilled holes [11].
From Table 7, it is identified that the spindle rotational speed (N) is the principal effecting drilling parameter which influences the circularity error succeeded by drilling cutter feed rate and material type [12]. Spindle rotational speed affects the circularity error by 39.57%, feed rate by 19.06% and drill bit material by 23.22%. In the present model, the p value < 0.005 illustrates that the drill tool material, feed rate and rotational speed are the key process parameters. Where, DF degree of freedom, Seq.SS sequence sum of square, Adj.SS adjacent sum of squares, Adj.MS adjacent mean square, F Fisher’s value P probability of significance.
Figure 3 shows that a minutest quantity of circularity error transpired at the highest rotational speed considered in the study, i.e. 10,600 rpm and a squat feed rate of 159 mm/min with coated tungsten carbide (WC-C) drill bit.
4.2 Effect of machining parameters on cutting temperature
Figure 4 gives the thermal images which indicate the temperature variations with respect to different drill bit materials investigated in the present study. Figure 4a shows the drilling zone with work piece and drill bit set-up, and Fig. 4b gives the usage of FLIR E60 thermal camera for temperature measurement in real time. Temperature variations with HSS drill while drilling holes on Ti–6Al–4V specimens are presented in Fig. 4c, d. Figure 4c indicates the initial stage, and Fig. 4d represents the final stage with HSS drill tool. Similarly, Fig. 4e (uncoated WC drill bit tool) and Fig. 4g (PVD-TiN-coated WC drill bit tool) shows the temperature rise at initial stages. Whereas Fig. 4f (uncoated WC drill bit tool) and Fig. 4h (PVD-TiN-coated WC drill bit tool) presents the temperature rise levels at ending stages of respective machining condition programmed in the investigation.
From Table 8, it is identified that the drill bit material type (DM) is the key cutting parameter which influences the temperature followed by the spindle rotational speed (N) and feed rate (f). The drill bit material type affects the temperature by 91.54%, the rotational speed by 5.64% and feed rate by 0.44%.
From Table 8, the parameter p value < 0.005 specifies that the spindle rotational speed and drill tool material remain the major influential parameters of process in the model, whereas for temperature, the feed rate was observed to remain a less significant parameter. The outcome of variable rotational speed of the spindle, feed rate and drill tool material on temperature is displayed in Fig. 5. It can be observed from Fig. 6 that the temperature increases at augmented rotational speed from 4200 to 10,600 rpm and also ascends with amplified feed rate from 159 to 400 mm/min.
From Fig. 5, it is clear that the temperature rise is the highest while drilling the work piece with high-speed steel drill bit tool among three different drill bits, irrespective of machining combination. As expected [13], cutting temperature increased with the elevation of rotational speed in titanium drilling with PVD-coated drill as well, but this tendency is more predominant with HSS drill bit. The reason may be that material removal rate increased significantly with increase in the feed rate which resulted in increased heat generation [14].
4.3 Effect of cutting parameters on flank wear
Drill bit tool’s flank wear can be measured by direct method as shown in Fig. 1. For a drill bit, flank wear is represented with (VB) in mm, which can be calculated according to the following relation:
where \(\alpha\) is the rake angle of the drill tool (in degrees), \(\gamma\) is the helix angle of the drill bit (in degrees) and \(\Delta D\) is the change in the diameter of the drill bit (mm) due to tool wear. From Table 9, it is found that the drill bit material type is the chief impelling cutting process parameter which influences the drill bit flank wear (VB) followed by the rotational speed of the spindle (N) and feed rate (f) [15]. The drill bit material type affects the flank wear by 78.79%, the rotational speed by 14.61% and feed rate by 1.3%. The statistical parameter p value < 0.05 implies that the drill bit material type and rotational speed are the two foremost process parameters in the model, and it is also identified that the influence of feed rate is very minimal in the process.
Figure 6 shows the outcome of variable feed rate, spindle rotational speed and drill tool material on flank wear values. From Fig. 6, it can be observed that the wear of the flank is amassed with rise in rotational speed of the spindle from 4200 to 10,600 rpm. Flank wear values exhibit similar trend with increase in feed rate from 159 to 400 mm/min.
From Fig. 6, it is clear that the flank wear values are found to be small as long as machining is carried out with coated tungsten carbide drill.
4.4 Phases aimed at optimization by grey relational analysis (GRA)
In this work, GRA along with the Taguchi technique is used to optimize the process parameters of drilling. Computing the resultant responses is the foremost step, i.e. S/N ratio values either smaller is better or larger is better type problem based on the output variables [16, 17]. The normalized original order will attain the required objective value. Otherwise, original order can simply be normalized using straightforward methodology, i.e. allow the values of the inventive order be divided by the starting value of the order:
where Xi*(K) is the order after the data pre-processing and X0i is the desired value of X0i (K), where i = 1,2,3…m; k = 1,2,3,…n. Where m is the experimental data item number, n is the parameter number and X0i (K) denotes the original order [18]. Normalized S/N ratio value of the response variables and their results are tabulated in Table 10.
The next step in the grey relational analysis is to quantify the grey relation grade (GRG). The GRG is defined as the measure of relevancy among two orders or two systems. In the measurement of local grey relation, all the orders are compared with the reference order X0i (K). After processing the data once, the Kth performance characteristics of the GRC in the ith experiment can be operated by:
where ∆oi (K) is the reference order Xo*(K) deviation and the comparability order Xi*(K). ξ is the coefficient of identification or distinctive well-defined in the range of 0 ≤ ξ ≤ 1 (this value can be in tune with the real-world requirements of the system). If the value of ξ is the small and the distinguished ability is the large, once grey relational coefficient (GRC) is determined, take the average of the GRC as the GRG. The following relationship is used to calculate GRG:
The GRA is carried out by evaluating the GRC for normalized S/N ratio values, and the resulting GRG values are shown in Table 11 using Eqs. (6) and (7).
4.5 Determination of optimum process parameter combination
From the analysis of drilling process, it is clearly evident that the lower circularity error and lesser cutting temperature value as well as lower value of tool flank wear provide drilled holes with better quality. Consequently, the data orders circularity error, cutting temperature and tool flank wear pinpoint the “smaller-the-better” characteristics. Higher GRG indicates better machinability combination hence the quality of the product is better. Consequently, on the basis of higher GRG or the rank, the optimal process parameters level can be achieved.
4.6 Identification of significant factor
In order to realize the remarkable factors on measurement, analysis of variance ANOVA for GRG is used as an analytical tool by observing the association among the proposed factor and response variable.
Taguchi method cannot choose the outcome of distinct drilling process parameters on the entire process; therefore, the fraction of involvement is premeditated by means of ANOVA on its behalf. Empirical Eq. (7) is utilized to compute average GRG values and is presented in Table 11. The specific effects of input factors on GRG are presented in Fig. 7. The utmost influencing factor on the GRG is identified as drill bit material type (68%), followed by the rotational speed (16%) and feed rate (7%), and these results are tabulated in Table 12.
4.7 Prediction of optimum condition
At the optimum condition, an anticipated mean is given by:
where \(\overline{{V_{3} }}\), \(\overline{{DB_{2} }}\) and \(\overline{{F_{1} }}\) are the average values of GRG concerning to the best operational condition input process parameters. \(\overline{{T_{\text{AGG}} }}\) is the average GRG of the complete experimentations. Consequently, the optimum condition predicted mean from Eq. (9) is
5 Mathematical modelling
The regression analysis [19] is used to determine the correlation of cutting temperature; circularity error and tool wear with drilling process parameters. In the present work, to forecast the minimum cutting temperature, tool wear and circularity error under every condition with an objective to evaluate the tool performance level, regression analysis is used. The second-order equations of the circularity error, cutting temperature and tool wear are presented in Table 13.
6 Confirmation of the experimentation
The confirmation experimentation is carried out at the optimal conditions to confirm the quality features while drilling of Ti–6Al–4V alloy. The response values by the confirmation experimentation [20] succession at the optimal condition are Cr = 0.009 μm, T = 90 °C and VB = 0.053 mm. The GRG value as per the above discussion is 0.9512. This outcome is inside the 95% confidence level of the anticipated optimal condition, and moreover, GRA value of confirmation experiment enhanced by 2% from the forecasted mean value. Therefore, the GRA built on Taguchi method for the optimization of the multi-response problems is a very beneficial tool for forecasting the circularity error, cutting temperature and drill bit tool wear in the drilling of Ti–6Al–4V alloy specimens.
7 Conclusions
Drilling experimentations have been carried out with high-speed steel, tungsten carbide and TiN-coated tungsten carbide twist drill and Ti–6Al–4V alloy as work material. Circularity error, cutting temperature and drill bit tool wear values have been collected under different machining conditions. The subsequent conclusions are drawn:
Grey relational analysis together with Taguchi method for the optimization of the multi-response problems is a very beneficial means for forecasting the circularity error, cutting temperature and tool wear in the drilling.
From the GRA analysis, it is discovered that cutting tool material, rotational speed of the spindle and feed rate are protuberant issues which disturb the drilling of difficult to cut material like Ti–6Al–4V alloy.
Drill bit material influences (DM = 73.67%) more, followed by rotational speed (N = 16.87%) and feed rate (f = 7.86%). The best performance features are attained with TiN-coated tungsten carbide twist drill bit when drilling with higher feed of 400 mm/min and higher rotational speed of 10,600 rpm at constant cutting point angle.
Confirmation test outcomes demonstrated that the determined optimal condition of drilling process parameters to fulfil the real necessities of drilling operation.
References
Ezugwu EO (2005) Key improvements in the machining of difficult-to-cut aerospace super alloys. Int J Mach Tools Manuf 45(12–13):1353–1367
Mukherjee I, Ray PK (2006) A review of optimization techniques in metal cutting processes. Comput Ind Eng 50(1–2):15–34
Trent EM, Wright PK (2000) Metal cutting. Butterworth-Heinemann, Oxford
Prasanna J, Karunamoorthy L, Raman MV, Prashanth S, Chordia DR (2014) Optimization of process parameters of small hole dry drilling in Ti–6Al–4V using Taguchi and grey relational analysis. Measurement 48:346–354
Giasin K, Ayvar-Soberanis S (2017) An investigation of burrs, chip formation, hole size, circularity and delamination during drilling operation of GLARE using ANOVA. Compos Struct 159:745–760
Kivak T, Habali K, Şeker U (2012) The effect of cutting parameters on the hole quality and tool wear during the drilling of Inconel 718. Gazi Univ J Sci 25(2):533–540
Haq AN, Marimuthu P, Jeyapaul R (2008) Multi response optimization of machining parameters of drilling Al/SiC metal matrix composite using grey relational analysis in the Taguchi method. Int J Adv Manuf Technol 37(3–4):250–255
Shunmugesh K, Kavan P (2017) Investigation and optimization of machining parameters in drilling of carbon fiber reinforced polymer (CFRP) composites. Pigm Resin Technol 46(1):21–30
Prasad BS, Prabha KA, Kumar PG (2017) Condition monitoring of turning process using infrared thermography technique—an experimental approach. Infrared Phys Technol 81:137–147
Sultan AZ, Sharif S, Kurniawan D (2015) Effect of machining parameters on tool wear and hole quality of AISI 316L stainless steel in conventional drilling. Proceedia Manuf 2:202–207
Reddy YRM, Prasad BS (2016) Simulation of form tolerances using CMM data for drilled holes-an experimental approach. J Prod Eng 19(2):77–83
Kushnoore S, Noel D, Kamitkar N, Satish Kumar M (2016) Experimental investigations on thrust, torque and circularity error in drilling of aluminium alloy (Al6061). Am J Mech Ind Eng 1(3):96–102
Wei Y, An Q, Ming W, Chen M (2016) Effect of drilling parameters and tool geometry on drilling performance in drilling carbon fiber–reinforced plastic/titanium alloy stacks. Adv Mech Eng 8(9):168781–4016670281
Gupta V, Pandey PM, Mridha AR, Gupta RK (2017) Effect of various parameters on the temperature distribution in conventional and diamond coated hollow tool bone drilling: a comparative study. Proceedia Eng 184:90–98
Sanjay C, Neema ML, Chin CW (2005) Modeling of tool wear in drilling by statistical analysis and artificial neural network. J Mater Process Technol 170(3):494–500
Yunus M, Alsoufi MS, Munshi SM (2016) Taguchi-Grey relation analysis for assessing the optimal set of control factors of thermal barrier coatings for high-temperature applications. Mech Adv Mater Mod Process 2(1):4
Tosun N (2006) Determination of optimum parameters for multi-performance characteristics in drilling by using grey relational analysis. Int J Adv Manuf Technol 28(5–6):450–455
Markopoulos AP, Habrat W, Galanis NI, Karkalos NE (2016) Modelling and optimization of machining with the use of statistical methods and soft computing. In: Design of experiments in production engineering. Springer, Cham, pp 39–88
Saha A, Mandal N (2013) Optimization of machining parameters of turning operations based on multi performance criteria. Int J Ind Eng Comput 4(1):51–60
Prasad BS, Babu MP (2017) Correlation between vibration amplitude and tool wear in turning: numerical and experimental analysis. Eng Sci Technol Int J 20(1):197–211
Acknowledgements
This work is supported by the Science and Engineering Research Board, Department of Science Technology, Govt. of India under Empowerment and Equity Opportunities for Excellence in Science Scheme with a Grant No: SB/EMEQ-265/2014. Authors would like to thank mentor Sri. P.V.S. Ganesh Kumar, Scientist-H, Associate Director, Naval Science Technological Laboratory Visakhapatnam, India for providing the experimentation test facility and necessary equipment.
Author information
Authors and Affiliations
Corresponding author
Additional information
Technical Editor: Lincoln Cardoso Brandao.
Publisher's Note
Springer Nature remains neutral with regard to jurisdictional claims in published maps and institutional affiliations.
Rights and permissions
About this article
Cite this article
Prasad, B.S., Sai Ravi Kiran, D.S. Experimental investigation to optimize tool performance in high-speed drilling: a comparative study. J Braz. Soc. Mech. Sci. Eng. 41, 535 (2019). https://doi.org/10.1007/s40430-019-2049-4
Received:
Accepted:
Published:
DOI: https://doi.org/10.1007/s40430-019-2049-4