Abstract
This paper presents an overview of research pertaining to solid acid catalysts for esterification reactions. Prominence has been given to the literatures that have been appeared during the last two decades. A variety of reactions catalyzed by solid acid catalysts have been tabulated according to their broad classification; industrially important reactions have been outlined. Examples, where the use of various solid acid catalysts have led to an improvement in the selectivity of the desired products, have also been discussed. Various catalyzed esterification reactions using different approaches and previous kinetic studies have been reviewed. Types, preparation and synthesis of various solid acid catalysts have been reviewed and discussed. Suggestions have been summarized for their implementation in future work.
Similar content being viewed by others
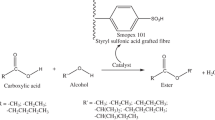
Explore related subjects
Discover the latest articles, news and stories from top researchers in related subjects.Avoid common mistakes on your manuscript.
Introduction
Esterification reaction is one of the most important processes in organic synthesis usually catalyzed in liquid phase. It is also an industrially important reaction as organic esters are significant intermediates in the synthesis of fine chemicals, drugs, plasticizers, food preservatives, cosmetics and auxiliaries. Mineral acid catalysts such as hydrofluoric acid, sulphuric acid or Lewis acid catalysts like tin octoate are used to produce by industrial batch process. Ecofriendly heterogeneous catalysts are in demand for esterification, e.g. heterogeneous catalysts viz., ion-exchange resin, zeolite, triolic acid, sulphate oxides, hydrous zirconium oxide and supported heteropoly acid, e.g. Esterification of acetic acid with ethanol over zeolite, sulphonic acid functionalized zeolite type silica for esterification of glycerol with fatty acids [1, 2].
Esterification Reactions
Catalysis Esterification Reactions
Homogeneous Acid-Catalyzed Esterification
Traditionally, homogeneous acid catalysts such as AlCl3, HF, and H2SO4 are commonly used in the esterification process industry. However, the production of large volumes of toxic waste which possesses environmental risk and the cost inefficiency are some of the drawbacks [3]. Homogeneous acid catalysts have many disadvantages in industrial and laboratory processes, e.g., in terms of handling, corrosiveness, difficult separation, and toxicity of waste [4].
Heterogeneous Acid-Catalyzed Esterification
In recent years, the search for environmentally benign chemical processes or methodologies has received much attention, and the development of heterogeneous catalysts for fine chemical synthesis has become a major area of research. The potential advantages of these materials over homogeneous systems (simplified recovery and reusability, the potential for incorporation in continuous reactors and micro reactors) could lead to novel environmentally benign chemical procedures for academia and industry [5].
Homogeneous acid catalysts have many disadvantages in industrial and laboratory processes, e.g., in terms of handling, corrosiveness, difficult separation and toxicity of waste. Most novel solid acid catalysts are based on a silica support, as they offer excellent chemical and thermal stability, high surface area and good accessibility of reactive centers and also organic moieties can be anchored to their surface to provide heterogeneous solid acid sites. Sulfonic-acid-functionalized silica (SiO2–Pr–SO3H) is a highly efficient heterogeneous nanoporous solid acid catalyst which can be easily synthesized and used in esterification reactions [3].
Keeping in view the importance of heterogeneous catalysis, a mild and efficient method for the one-pot synthesis of 3,4-dihydropyrimidinones/thiones in the presence of covalently anchored sulfonic acid onto silica gel, which has been reported [6]. Various types of alcohols and phenols are converted to the corresponding ether using a solid silica-based sulfonic acid [7].
The heterogeneous catalysts can be used in place of homogeneous catalysts, e.g. use of solid catalysts (sulfonated hyper cross-linked polystyrene resin, sulfonated polyvinyl alcohol and sulfated zirconia) for transesterification of triglycerides and esterification of free fatty acid for biodiesel production [8]. Oils with high amount of Free Fatty Acid (FFA) are becoming one of the most promising alternatives to produced biodiesel; due to its low cost. Different catalysts, such as solid resins, zeolite and enzymes were tested for the direct esterification reaction of pure oleic acid with different alcohols [9].
Enzymatic Esterification
In the case of enzymatic esterification reactions, water is produced during the reaction due to hydrophobic solvents and water phase in the system. A subject of study was the synthesis of geranyl acetate, one of the most known aromatic compound. A hydrophobic (polypropylene) matrix was shown to be a much better carrier in the reactions performed in an organic solvent than a hydrophilic (polyamide) membrane being tested [10]. Biocatalytic synthesis of esters using Rhizopus oryzae resting cells has also been reported [11].
Supercritical and Subcritical Alcohol Esterification
To predict the performance of packed-bed plug flow bioreactor operating under supercritical conditions, the reaction kinetic data can be provided e.g. kinetics of esterification of oleic acid and ethanol using Biocatalyst Lypozyme. It was found that under higher alcohol concentrations, the reaction experienced substrate inhibition. The latter was identified to be competitive inhibition under moderate alcohol concentrations and non-competitive inhibitions under high alcohol concentrations [12].
Microwave Assisted Esterification
Inspired by the concept of Ionic Liquids (ILs), study showing modified Mukaiyama’s reagent, 2-chloro-1-methylpyridinium iodide, from ionic solid into liquids by changing its anion can be useful for microwave assisted ‘greener’ trans-esterification. The microwave irradiation was more effective in esterifying N-acetyl-l-phenylalanine than the conventional reflux method for its esterification [13].
Amberlyst-15 has been demonstrated to be an effective catalyst for the Fischer type synthesis of esters in a heterogeneous, aqueous system. Even the factory-dried Amberlyst-15 resin contains traces of moisture. In addition, the Amberlyst-15 is highly hygroscopic and readily adsorbs water from the air upon short exposure to ambient conditions. In studies of microwave-assisted chemical conversions it is important to concentrate on possible factors influencing reaction progress. Since 2.45 GHz microwaves are “tuned” to heat water with high efficiency. In principle, water present in the catalytic resin could facilitate superheating of the resin, leading to an increased reaction rate under microwave radiation and also facilitate proton transfer which influence the reaction kinetics. To test the effect of water contained within the resin upon reaction progress, rates of reactions have been measured. These reactions were heated by microwave radiation and classical heating, in the presence of catalytic resin loaded with varying amounts of water [14].
Trans-Esterification Reactions
Trans-esterification (alcoholysis) is a reversible reaction of a fat or oil with an alcohol to form esters and glycerol, catalyzed by base/alkalis, acids or enzymes. Excess alcohol is required to favor the reaction in the forward direction [8]. Novel water tolerant sulfonic acid based periodic mesoporous organo silicas having either phenylene or ethyl as bridge and methyl propyl sulfonic acid as functionalized group, which have been developed for efficient biodiesel production via direct trans-esterification of various oils (sunflower, canola, corn, refined olive and extracted oil from olive sludge) with methanol. By comparing the catalytic performance of these acids with well-known (SBA–Pr–SO3H), it was revealed that catalyst bearing an ethyl bridging group is a more reactive catalytic system in biodiesel production [15]. The use of homogeneous sulfuric acid was used to prevent soap formation in biodiesel production using either esterification or trans-esterification. It has been reported as a promising and recyclable catalyst for trans-esterification of various vegetable oils, by emphasizing the possibility to control both acidity and hydrophobicity of the catalysts [15].
Previous Kinetics Studies of Esterification Reactions
Catalytic activity of esterification reactions are generally confirmed by H-NMR and IR spectroscopy [1]. Shah et al. [16] reported esterification of FFAs in acid oil (a byproduct of oil refining) to obtain biodiesel using sulfonic acid-functionalized silica (SiO2–Pr–SO3H) as promising solid-acid catalyst. The conditions affecting conversion to Fatty Acid Methyl Esters (FAME), for example reaction temperature, reaction time, catalyst concentration, and methanol-to-oil molar ratio, were investigated and optimized. The highest conversion obtained under the optimized conditions was 96.78 %. Shah et al. [16] made the assumptions, which include (1) The negligible rate of the non-catalyzed reaction compared with that of the catalyzed reaction (2) The oil phase reaction occurred (3) constant concentration of methanol due to high oil-to-methanol molar ratio and (4) reversible heterogeneous esterification of FFA. Rate constants of the reaction at different temperatures were determined on the basis of the increase in the amount of FAME as a function of time [16].
The kinetics of acetic acid esterification with ethyl alcohol, in vapor phase, have been studied in the presence of decationized zeolite catalysts [17]. Different solid acid catalysts were used to assess their efficacy in esterification reaction of ethanol and maleic acid [18]. Esterification kinetics of acetic acid with isobutanol in 1,4-dioxan as a solvent without adding a catalyst and catalyzed by ion-exchange resins were carried out using a batch reactor. The effect of catalyst type, stirrer speed, the effects of reaction temperature and catalyst loading on the initial reaction rate, the effect of speed of agitation and the conversion of acetic acid versus time were also investigated [19]. Four homogeneous acidic catalysts were tested for their ability to catalyze the esterification reaction of oleic acid and oleyl alcohol to produce oleyl acetate [20]. Yadav et al. [18] reviewed and reported that p-toluene-sulfonic acid as homogenous catalyst which can be used for esterification of maleic anhydride and ethanol. Esterification of different acids (acetic, propanoic and pentanoic) with alcohols (methanol, ethanol, 1-propanol, butanol and 2-butanol) was studied in the presence of heterogeneous and homogeneous catalysts [21]. An optimal continuous procedure was developed for the esterification of ethanol with maleic acid by using sulfonic acid functionalized silica as catalyst in a packed bed reactor [22].
The esterification of ethylene glycol with acetic acid was investigated in a batch reactor in presence of a strongly acidic cation exchange resin (Amberlyst 15). The reaction of ethylene glycol with acetic acid in presence of cation-exchange resin proceeds in two consecutive reversible steps as shown in Fig. 1 [23]. A complete kinetic modeling, and simulation were carried out to design a catalytic distillation column for removing acetic acid from wastewater [16]. Sulfonated polyvinyl alcohol catalyst has been studied in the same way the esterification of oleic acid and rearrangement of α-pinene. The activity of the catalyst was compared with sulfonated macro reticular polystyrene resin Amberlyst-35 and Nafion. In the esterification reaction, sulfonated polyvinyl alcohol showed a better activity than Nafion and the Amberlyst-35 resin catalyst [8, 9]. The esterification of salicylic acid with methanol was carried out over a series of Ce4+ modified cation-exchange resins. The effect of different reaction conditions was studied on the conversion of salicylic acid, and the optimal reaction parameters were obtained. The reusability of Ce4+ modified cation-exchange resins was also studied by using salicylic acid and methanol as model substrates. The mechanism was proposed for the esterification of salicylic acid with methanol over Ce4+ modified cation-exchange resins [24]. Ketalization of acetone with 1,4- and 1,2-diols was carried out in the presence of cation exchange resin catalysts to obtain corresponding cyclic dioxepins, dioxepanes and dioxolanes. The reaction was equilibrium controlled and low conversions were achieved in batch mode, and kinetics was explained by a pseudo homogeneous model. The effects of different configurations as well as various parameters were studied in detail to arrive at optimum conditions [25]. The reactive distillation provides an attractive alternative for reaction/separation processes with reversible reactions, especially for etherification and esterification. The esterification of acetic acid with five different alcohols, ranging from C1 to C5, have been studied [26].
Esterification of ethylene glycol with acetic acid in the presence of solid acid catalyst. Reprinted with permission of Ref. [23]
Types, Preparation and Synthesis of Catalyst for Esterification Reactions
The factors to be considered when synthesizing catalyst are the optimum structure for efficient use, main characteristics of the catalysts and their preparation method as well as auxiliary steps be carried out to achieve the best characteristics of the resulting catalyst [27].
Polymer Supported Sulfonic Acid Resins
Synthetic ion exchange materials based on coal and phenolic resins were first introduced for industrial use during the 1930s. A few years later resins consisting of polystyrene with sulphonate groups to form cation exchangers or amine groups to form anion exchangers were developed (Fig. 2). These two kinds of resin are still the most commonly used resins today [28].
Some examples of ion exchange resins: a a strongly acidic sulphonated polystyrene cation exchange resin, b a strongly basic quaternary anion exchange resin. Reprinted with permission of Ref. [28]
Conventional ion exchange resins consists of a cross-linked polymer matrix with a relatively uniform distribution of ion-active sites throughout the structure. A cation exchange resin with a negatively charged matrix and exchangeable positive ions (cations) is shown in Fig. 3. Ion exchange materials are sold as spheres or sometimes granules with a specific size and uniformity to meet the needs of a particular application [29].
Cation exchange resin schematic showing negatively charged matrix and exchangeable positive ions. Reprinted with permission of Ref. [29]
Diethoxymethane was prepared in batch mode as well as in continuous reactive distillation column from ethanol and aqueous formaldehyde in the presence of a macroporous cation-exchange resin Indion-130 [30].
Diethyl maleate is an important intermediate extensively used in the production of latex emulsion polymers, thermoplast and thermoset plastics. Yadav et al. [18] found and delineated the efficacy of Indion-170, Amberlyst-36, Amberlyst-15 as most effective heterogeneous catalysts for the esterification of maleic acid with ethanol [18]. The activities are in order of cation-exchange capacity on dry basis for Amberlyst-36, and Amberlyst-15 are 5.40 and 4.8 mequiv./g, respectively, whereas these values are reported less than 0.5 mequiv./g for clays. The characteristic properties of the cation exchanged resins are given in Table 1 [18].
Chakrabarti et al. presented a review of the literature pertaining to catalysis by cation exchange resins emphasizing to the literature during nineties [31]. A variety of reactions catalyzed by cation exchange resins have been tabulated according to their broad classification; industrially important reactions have been also delineated. Examples, where the use of ion exchange resin catalysis has led to an improvement in the selectivity of the desired products, have also been discussed. Approaches for the interpretation of kinetic data and modeling have been reviewed. The use of ion exchange resins for the removal of impurities from mixtures and the separation of close-boiling compounds by exploiting their different chemical reactivity has been highlighted [31].
All the reactions generally catalyzed by mineral acids, can be carried out in the presence of cation exchange resin as catalyst with some of the advantages. The physical properties of relevant cation exchange resins are listed in Table 1. Chakrabarti et al. reported many more reactions catalyzed by ion exchange resins in Table 2 which lists [31].
Various ion exchange resin have been reported for the separation of close boiling compound, such as separation of isobutylene/1-butene, m-cresol/p-cresol, 2,6-xylenol/p-cresol henol/o-chlorophenol, o-ethylphenol/guaiacol, a-naphthol/3-naphthol, ethanol/isopropanol, 2-nitrobenzaldehyde/3-nitro-benzaldehyde, isopropanol/tert-butanol 2-methylbutanal/methyl isopropyl ketone. These resins have also been reported for the removal of formaldehyde impurity from aquepyl ketoneous solution of butynediol, p-cresol impurity from 2,6-xylenol, a-naphthol impurity from naphthol. Acid-catalyzed reactions are industrially important and the proper choice of the catalyst is vital to make a process cost-effective. The acid-catalyzed reactions can be carried out with both homogeneous and heterogeneous catalysts. Heterogeneous catalysts have certain prominent advantages over their homogeneous counterpart, such as easy separation of the catalyst, better selectivity and reusability of the catalyst. Heterogeneous catalysts which are generally employed for acid-catalyzed reactions zeolites, ion exchange resins, acid-activated clays [31]. The kinetics of the esterification reaction of propionic acid with 1-propanol over the ion-exchange resin has been studied [32].
Sulfonated Poly (Styrene-Co-divinylbenzene) Ion Exchange Resins
Hypercrosslinked resin catalysts differ from conventional cross-linked polystyrene in having much more extensive cross-linking, by methylene groups linked with neighboring styrene units. In fact, if applied to polystyrene resins with existing permanent macro porosity, the additional crosslinking results in additional micro porosity in a hierarchical pore structure of catalyzed [8]. Polystyrene-supported sulfonic acid catalysts have been extensively studied in the direct esterification of FFA and similar reactions. Most studies of polystyrene sulfonic acid resins have been based on those with permanent macro porosity such as Amberlysts-15, 35 and 36 (sometimes referred to as “macroreticular” resins), surprisingly, that some “gel” resins can exhibit slightly higher activities. Conventional macro porous polystyrene resins rely on polymer crosslinking with divinylbenzene and the incorporation of an inert porogen compound at the polymerization stage to impart permanent porosity. They are synthesized by carrying out a second crosslinking reaction on polystyrene/divinylbenzene using typically chloro dimethyl ether with a suitable Friedel–Crafts catalyst [8]. Acidic cation exchange resin supported polymer materials are well established and widely reported in literatures for use in liquid phase reactions. Commercially available resin catalysts are produced in the form of spherical beads with diameters of 0.5–1.0 mm. Commercially produced ion exchange resins exist in two main forms, gel and macro porous. Gel type resins are polymerized as uniform beads with smooth external surfaces. Macro porous resins are polymerized as beads in the presence of additional compound known as porogens which is to be trapped within the polystyrene and after polymerization is complete they are dissolved out leaving spherical beads with a degree of permanent porosity [33].
Sulfonated Fluorinated Polymer Resins
Per-fluorinated sulfonic acid based ion exchange resins are currently of high interest when compared to the commercially available sulfonated polymeric supported resins. The comparatively higher acid strength is believed to be due to the presence of the highly electronegative fluorinated regions of the resin. Nafion is a copolymer of tetra fluoro ethene and perfluoro-2-(fluoro sulfonyl ethoxy) propyl vinyl ether. It has been found to increase many reaction when compared to traditional homogenous catalysts, including alkylations, acylations, isomerizations, esterifications, hydrations, dehydrations, nitrations and etherifications. The resin is commercially available in bead form having a small surface area of less than 0.02 m2/g, and a concentration of only 0.8 mmol/g acid sites. The subsequent surface area of the catalyst was greatly increased, despite its much lower concentration of acid sites at 0.1 mmol/g, and yet there was an increased accessibility to the active acid sites due to the sol–gel synthesis of a porous material support. The silica supported sulfonated Nafion composite is also commercially available and was researched here as another benchmark comparison to not only the sulfonated polymeric resins, but also to the synthesized silica supported materials [33].
Sulfonated Silica Gels
The grafting route was employed in the synthesis of Sulfonic Acid Functionalized Silica (SAFS) SiO2–Pr–SO3H. The procedure is summarized in following Fig. 4. All these modified catalysts can be characterized by Fourier Transformation Infrared Spectroscopy (FTIR), Thermo Gravimetric Analysis (TGA), Field Emission Scanning Electron Microscope (FESEM), BET surface analysis and X-Ray Diffraction (XRD). These characterizations confirm that the surface of inorganic substances is modified satisfactorily.
Silica supported sulfonic acid catalysts can be a better alternative to commercially available sulfonic acid resins and zeolites. Sulfonic acid resins such as Amberlyst-15 suffer from low surface area and thermal stability while zeolites provide some reaction products with high conversion and selectivity [5]. However, these catalysts also suffer from diffusion restrictions due to small pore diameter which makes them unsuitable for liquid phase reactions, especially with bulky molecules such as 1,3-dioxane and 1,3-dioxolanes. To overcome this drawback, a number of silica supported “green catalysts” (non-volatile, recoverable, and easy-to-synthesize) such as sulfonic acid or MCM-41 sulfonic acid have been employed in esterification, processes. However, the tedious work-up procedure (several hours of refluxing), use of hazardous chemicals such as tetraethoxy silane, poly(ethylene glycol)-block-poly(propylene glycol)-block-poly(ethylene glycol), cetyl trimethyl ammonium bromide etc., either as the silica source or template, are considered the main drawbacks of this type of catalysts. One-step process was used to synthesize a silica supported acid catalyst using rice husk ash as a cheap silica source in a short preparation time (32 min) under ambient conditions [5]. The waste by-product silica after thermal activation (1 h at 800 °C temperature) is possible to use in hardened cement paste having 7 MPa strength [34]. Several types of sulfonic acid-functionalized silica has been synthesized and applied as an alternative to traditional sulfonic resins in catalyzing chemical transformations [7].
Sulfonic-acid-functionalized silica have been used as an efficient heterogeneous acid catalyst for the preparation of amides by reaction of various benzylic, allylic and tertiary alcohols with various nitriles in good to excellent yields under solvent-free conditions. The simplicity of the reaction, recovery of catalyst without loss of reactivity, high yield of products, and short reaction time represent improvements over many existing methods. For preparation of the SiO2–Pr–SO3H as solid acid catalyst, functionalization of SiO2 with –SO3H group was performed by direct synthesis or post grafting. As shown in Fig. 5, SiO2 was functionalized with 3-mercaptopropyltrimethoxysilane (MPTMS), then the thiol groups of the product were oxidized to sulfonic acid by hydrogen peroxide [3].
Preparation of SiO2–Pr–SO3H as solid acid catalyst. Reprinted with permission of Ref. [3]
The reaction of 3-mercaptopropylsilica and chlorosulfonic acid in chloroform afforded silica bonded S-sulfonic acid which was used as a catalyst for the room temperature synthesis of quinoxaline derivatives from 1,2-diamino compounds and 1,2-dicarbonyl compounds [8]. A one-pot method was also employed to immobilize sulfonic acid onto silica obtained from rice husk ash using MPTMS to form a solid catalyst [5]. Gupta et al. reported a novel covalent anchored sulfonic acid onto the surface of silica which was further investigated for the Bignelli reaction for the synthesis of 3,4-dihydropyrimidinones/thiones [6]. To study the reusability of SiO2–Pr–SO3H, this catalyst was regenerated by recovering from drying, washing with acetone and hexane, and then allowed for filtration. The reutilization tests revealed reduction in catalytic activity determined by acid–base titration. This attrition of catalytic activity may be caused by deposition of reactants inside pores of the catalyst support, although deactivation of active sites by esterification of sulfonic acid groups are, to some extent, also responsible. FTIR spectra of SiO2–Pr–SO3H before and after use shown in Fig. 6a illustrates the bands at 1092, 800, and 464 cm−1 which are assigned to Si–O–Si stretching and bending vibrations, respectively, of SiO2. Weak absorbance at 1470 and 1420 cm−1 was assigned to bending vibrations of methylene groups, and broad absorbance observed in the region 2850–3000 cm−1, corresponding to the methylene stretching vibrations of the propyl chains, indicated incorporation of the organic moiety. Furthermore, disappearance of a weak peak at 2580 cm−1 (assigned to S–H stretching) confirms complete oxidation of thiol groups to sulfonic acid groups. These results are in accordance with the TGA analysis. The peaks corresponding to the S=O stretching vibrations of sulfonic acid were normally observed in the range 1000–1200 cm−1. The presence of sulfonic acid groups is also apparent from the presence of weak bands at 1370 cm−1, assigned to asymmetric stretching of SO2 moieties. For all the samples, the peaks associated with the noncondensed Si–OH groups at 470 cm−1 are present, and the strong peak at approximately 1630 cm−1 is mainly from the bending vibration of adsorbed H2O [16].
a FTIR spectra of SiO2–Pr–SO3H before and after the third reuse, b TGA analysis of thiol and sulfonic acid-functionalized silica. Reprinted with permission of Ref. [16]
To examine the extent of oxidation of the thiol functionality, thermal analysis curves of the thiol and organic sulfonic acid-functionalized silica were obtained (Fig. 6b). The TGA plot of both materials confirmed the incorporation of organic functionality in the silica framework and complete oxidation of the thiol groups to sulfonic acid. The TGA plot of SiO2–Pr–SO3H shows sharp weight loss below 100 °C and at 460 °C, which correspond to loss of physisorbed water and decomposition of organic sulfonic acid groups, respectively; Additional weight loss in the region of 360 °C was observed for SiO2–Pr–SH; this is attributed to loss of organo-sulfur fragments. Complete oxidation of thiol groups to sulfonic acid groups was further confirmed because negligible weight loss at 360 °C was observed (Fig. 6b). Furthermore, weight loss at 450–540 °C corresponds to 10 % incorporation of organic functionality into the silica framework, which is in accordance with the theoretical value [16].
Sulfonated Silica Gels (Grafting Route)
Commercially available amorphous silica is prepared by a sol–gel technique using an aqueous silicate solution with subsequent acidification, neutralization and polymerization reactions to form the silica hydrogel, or from reaction of silicon alkoxides with water, and the subsequent hydrolysis and self-condensation reactions to form the polysilicate. Functionalization of the amorphous silica gel can be achieved by grafting the desired functional groups to the silica surface. It is reported that various types of organo silane functional group ethers such as MPTMS, 2-4-chlorosulfonyltrimethoxysilane and 2-4-chlorosulfonylphenylethyltrimethoxysilane (CSPTMS) was grafted. Bi-functionalization of the material can also be achieved by the grafting of an additional phenyl group using phenyl triethoxy silane. Oxidation and acidification of the thiol groups to sulfonic acid was achieved by oxidation with hydrogen peroxide and acidification with sulfuric acid [33].
Propyl Sulfonic Acid Functionalized Silica Gel
SiO2–Pr–SO3H is a highly effective, reusable, and environmentally benign catalyst for biodiesel production from waste low-cost oil feedstock with a high FFA content. Surface functionalization of silica gel was carried out by overnight evacuation, drying, reflux heating, stirring, isolation by filtration, washing hot toluene several times, and dried in oven at 110 °C for 5 h [16].
Oxidation
For oxidation of the thiol group of the functionalized silica (SiO2–Pr–SH), a tenfold excess 30 % H2O2 have been used as oxidizing agent. The solid product (SiO2–Pr–SO3H) was isolated by filtration, washing with distilled water, and then suspended in 10 % H2SO4 solution for 4 h to ensure complete protonation of all sulfonic acid groups. Finally, the product was isolated by filtration, washed with water then ethanol, and air dried [16]. SiO2–Pr–SO3H catalyst was prepared by taking mixture of SiO2 in dry toluene with MPTMS, followed by their reflux, filtration, washing and drying. Then, resulting 3-mercaptopropylsilica (MPS) was oxidized with H2O2 and H2SO4 in methanol for 24 h, filtered and washed with H2O and acetone. The modified SiO2–Pr–SO3H was dried, which can be used as a solid acid catalyst in the organic synthesis [3].
Acidification
One-step preparation of solid silica sulfonic acid was also reported by mixing RHA with NaOH at 80 °C for 30 min followed by addition of MPTMS, titration with nitric acid under constant stirring, addition of H2O2 + ethanol mixture, centrifuging, separation, washing with acetone and drying and finally grounding to a fine powder [5].
Sulfonated Mesoporous Silicas (Co-condensation Route)
Hybrid hierarchically organized, monolithic silica gels comprising periodically arranged mesopores and a cellular macroscopic network have been prepared via a co-condensation approach of tetrakis (2-hydroxyethyl) orthosilicate and chloro methyl trimethoxysilane or 3-(chloropropyl)-triethoxysilane, respectively. Subsequent conversion of the chloro into azido groups via nucleophilic substitution with NaN3, followed by a Cu(I)-catalyzed 1,3-dipolar cyclo addition with several alkynes was successfully conducted within the monolithic gel structure [35].
Sulfonated mesoporous silica gels have been produced by co-condensation sol-gel synthesis routes, which enable the incorporation of the organic functional groups within the silica structure during synthesis with pore directing surfactant agents. This tailoring of the pore size also enables the material to be synthesized more specifically with regards its organic functionalization for control of the materials surface properties, hydrophilicity/hydrophobicity modification, alteration of the surface reactivity of the material. MPTMS was functionalized hexagonally structured mesoporous material [33]. Mesoporous type catalyst material can be synthesized via a co-condensation of alkoxy silanes and 3-mercapto propyl trimethoxy silane which is further oxidized and acidified to yield propyl sulfonic acid groups anchored onto the silica framework [33].
Inorganic Solid Supported Acid Catalysts
Alumina, zirconia, chromia, iron oxide, etc. pillared clays have attracted interest as solid acid catalysts for several acid-catalyzed reactions [1]. Molecularly imprinted organic–inorganic hybrid composite materials with controlled swelling and adsorption properties were prepared through copolymerization and the sol–gel process by using methacrylic acid, methacryloxy propyl trimethoxy silane and Tetraethyl orthosilicate (TEOS) [36]. Esterification of maleic anhydride with ethanol [2] and methanol [37] over Al-MCM- (Si/Al = 50,100 and 150) and zeolite was reported in the liquid phase at different temperature. Monoesterification of maleic anhydride to monoethyl maleate was found to be fast and independent in the presence of catalyst. The absence of isomerization of diethyl maleate to diethyl fumarate over Al-MCM-41 molecular sieves is a significant advantage. Al-MCM-41 is convenient replacement for hazardous sulphuric acid used in the industrial esterification of maleic anhydride with ethanol [2, 37]. A comparative study of activated carbon with evaluation of Fuller’s earth for the adsorption of mercury from aqueous solutions has been also reported [38]. Removal characteristics of Reactive Black 5 dye can be obtained by using surfactant-modified activated carbon [39] Effect of hydrophobic and hydrophilic properties of solid acid catalysts on the esterification of maleic anhydride with ethanol was also studied [2]. A novel sustainable process based on catalytic reactive distillation is proposed for manufacturing biodiesel via fatty acid esterification using metal oxides as solid acid catalysts which are investigated [40]. The pros and cons of manufacturing biodiesel via fatty acid esterification using solid acid catalysts were investigated. The most promising candidates were found to be the sulfated metal oxides that can be used to develop a sustainable esterification process based on catalytic reactive distillation [41].
Acid Treated Clays
Porous solids, particularly clay catalysts have attracted considerable attention as catalysts for a variety of organic reactions. Acid activated clays are used as catalysts, catalyst beds, adsorbents, and bleaching earth in industry. Acid activated clays constitute a widely available inexpensive solid source of protons, which are effective in a number of novel and industrially significant reactions and processes. Smectite clays and their modified forms were used as efficient catalysts in the esterification of alkenes with carboxylic acids. Esterification reactions on Fe-montmorillonite clay has been also reported. Highly efficient esterification of carboxylic acids with alcohols by montmorillonite-enwrapped titanium as a heterogeneous acid catalyst has been studied. Esterification of succinic anhydride with p-cresol and dicarboxylic acids to diesters were studied over cation exchanged montmorillonite clay catalysts.
The surface of inorganic clays like bentonite, montmorillonite and kaoline were modified by acid treatment methodology in two steps, surface activation and protonation of activated surface, as summarized in Fig. 7.
H-exchanged Indian bentonite and cation-exchanged clays were prepared by using AlCl3, FeCl3, CrCl3, MnCl2, or ZnCl2, with clay at room temperature [1]. Lemic et al. [42] successfully achieved a surface modification of sepiolite with quaternary amines [42]. Nanocomposites of polystyrene, acrylonitrile butadiene styrene copolymer and high impact polystyrene were prepared with two new homologous benzimidazolium surfactants used as organic modifications for the clays [43]. As a result of the cross-linking process between sodium alginate, Aluminium pillared clay (Al-Mont-PILC) or surfactant-modified pillared clay (CTAB-Al-Mont-PILC) and divalent cation (Ca2+), gel-like beads were obtained under different ratios of PILCs versus alginate. These clays have been utilized for removal of a neutral/anionic biocide (pentachlorophenol) and a cationic dye (safranine) from aqueous solutions [44]. Hexadecyltrimethyl ammonium HDTMA+ pillared montmorillonites were obtained by pillaring different amounts of the HDTMA bromide into sodium montmorillonite (Na–Mt) in an aqueous solution [45]. Blachier et al. for better understanding of the interaction between a polycationic quaternary amine polymer and different clay minerals: montmorillonite and kaolinite. For this, adsorption isotherms on the clay surfaces were measured which revealed that cation exchange plays an important role in the adsorption process [46].
The influence of structural characteristics of series of surfactant–clay complexes on their sorption capacities toward naphthalene were examined [47]. Li et al. reported the preparation of sodium dodecyl sulfate modified iron pillared montmorillonite and its application for the removal of aqueous Cu(II) and Co(II) [48]. Simillarly, Eren et al. reported method of adsorption of basic dye from aqueous solutions by modified sepiolite [49]. A cationic surfactant modified bentonite has been used for an adsorption study of textile dye Reactive Blue 19 dye [50]. Bentonite has been studied extensively because of its strong adsorption capacity [51]. The adsorption of anionic surfactants SDS and sodium bis [2-ethylhexil] sulfosuccinate (AOT) on hydrotalcite (HT) and the adsorption of 2,4-dichlorophenol (DCP) on HT and its modified forms from aqueous solutions was studied [52]. Fly ash is currently being generated at a rate of million tons every year and represents an important waste problem. Bentonite and molasses are used in a wide range of applications. Based on the experimental data, it was seen that Orhaneli fly ash and molasses waste could be evaluated for Zn2+ adsorption from wastewater, environmentally [53]. HDTMA-modified natural mordenite and HDTMA-modified natural clinoptilolite have been proposed for the removal of As(V) from aqueous solution [54].
A novel class of cationic gemini surfactants were prepared and used as modifiers for sodium montmorillonite (MMT-Na). These modified materials have the potential for removal of environment pollutants such as pesticides, phenol, etc. or being used as antimicrobial materials [55]. Removal of copper (Cu2+) and zinc (Zn2+) from aqueous solutions is investigated using Cankırı bentonite, a natural clay. It is concluded that natural clay can be used as an effective adsorbent for removing Cu2+ and Zn2+ from aqueous solutions [56]. The removal of the pollutant Supranol Yellow was studied by using different clays: clay exchanged with sodium and hydroxyaluminic polycation pillared clays in the presence or absence of non-ionic surfactant. Sorption study of an acid dye from an aqueous solutions using modified clays is also reported [57]. Adsorption of Supranol Yellow from aqueous solution by surfactant-treated aluminum/chromium-intercalated bentonite [58] was also reported.
Two-step kinetic study on the adsorption and desorption of reactive dyes at cationic polymer/bentonite [59] and copper ions on Na-montmorillonite [60] were studied. Adsorption properties of Congo Red from aqueous solution onto surfactant-modified montmorillonite [61] have been also reported. Cationic polyelectrolyte/bentonite prepared by ultrasonic technique and its use as adsorbent for Reactive Blue K-GL dye [62]. Effect of sonication on the particle size of montmorillonite clays [63] and adsorption of Direct Red 2 on bentonite modified by cetyltrimethyl ammonium bromide were also studied [64]. Adsorption of acid blue from aqueous solutions onto Na–bentonite and DTMA–bentonite [65] was also studied. The removal of phenol from aqueous solutions by adsorption using surfactant-modified bentonite and kaolinite [66] and simultaneous sorption of phosphate and phenanthrene to inorgano–organo-bentonite from water [67] were studied. Mechanism of p-nitrophenol adsorption from aqueous solution by HDTMA+-pillared montmorillonite was studied for implications for water purification [68] and removal of phenol from aqueous solutions by adsorption onto organomodified bentonite [69] were studied. A high-surface-area clay catalyst surfactant-treated montmorillonite [70] was also reported. Preparation of bentonite supported nano titanium dioxide photo catalysts by electrostatic self-assembly method [71] have been accounted. Adsorption equilibrium and kinetic studies of strontium on Mg-bentonite, Fe-bentonite and illite/smectite [72] have also important considerations.
Mesostructured materials belonging to a new class of solid acids known as porous clay heterostructures have been prepared by chemical modification of a natural clay, by using a cationic surfactant, a neutral amine, and an equimolar mixture of bis(triethoxysilyl) benzene and TEOS. This mixtures was stirred at 50 °C with CTAB, separated from the solution and washed with water until pH 7. The air-dried ion-exchanged clay was then stirred overnight with a mixture of TEOS and BTEB in the presence of a neutral amine as co-surfactant, which was followed by 4–12 h mixing, filtration and drying [73].
Conclusions
A literature survey revealed that propyl sulfonic acid-functionalized silica was found to be a promising solid-acid catalyst in view of its simple preparation, easy separation, reusability, and high activity. To enhance the reusability of the catalyst, further work must be conducted on regeneration of active sites. As alternatives, stepwise processes using microstructured reactors, lignin-derived carbonaceous catalysts, solid super acid catalyst, and a variety of enzymes have been used.
One possible way of overcoming such drawbacks (high reaction temperature, active site leaching, longer reaction time, high catalyst cost, and catalyst deactivation) is to bind sulfonic acid groups to inert support materials to obtain solid-acid catalysts. In such solids, the reactive centers are highly mobile, similar to those of homogeneous catalysts, and at the same time they have the advantage of recyclability, similar to heterogeneous catalysts.
On the basis of this idea, several types of sulfonic acid-functionalized silica have been synthesized and applied as alternatives to traditional sulfonic resins for catalyzing chemical transformations. The esterification of maleic acid with ethanol was studied by using different solid acid catalysts, i.e. Amberlyst-15 here, which was reported active catalyst as compared to Amberlyst-36.
The cationic ion exchange resins offer distinct advantages as catalyst for acid-catalyzed reactions, compared to the use of homogeneous catalysts and other heterogeneous catalysts. A variety of reactions can be carried out with cationic ion exchange resins as the catalyst. Efforts are being made to develop perfluorosulfonic acid resin with high surface area fibres, employing organo polysiloxane substrates rather than polystyrene, to boost their activity and thermal stability. Modified ion exchange resin may have the requisite potential for different industrially important reactions. It would be desirable to develop catalysts having large pore sizes so that bulkier molecules, such as terpenes, can be reacted via esterification, etherification and hydration. These catalysts can be used in an optimization study to determine the best reaction conditions by means of a statistical experimental design.
References
B. Vijaykumar, N. Mahadevaiah, G. Nagendrappa, B.S. Jai Prakash, Esterification of stearic acid with p-cresol over modified Indian bentonite clay catalyst. J. Porous Mater. 19, 201–210 (2012)
M. Bhagiyalakshmi, S. Vishnu Priya, J. Herbert Mabel, M. Palanichamy, V. Murugesan, Effect of hydrophobic and hydrophilic properties of solid acid catalysts on the esterification of maleic anhydride with ethanol. Catal. Commun. 9, 2007–2012 (2008)
G.M. Ziarani, A. Badiei, Z. Dashtianeh, P. Gholamzadeh, N.H. Mohtasham, Application of Sio2–Pr–SO3H as an efficient catalyst in the Ritter reaction. Res. Chem. Intermed. 39, 3157–3163 (2013)
D. Niknam, M. Saberi, M. Sejad, Silica bonded s-sulfonic acid: a recyclable catalyst for the synthesis of quinoxaline at room temperature. Molecules 14, 1915–1926 (2009)
A. Afarook, A.S. Batagarawa, B.M. Hello, C.S. Al-Juaid, One-step synthesis of solid sulfonic acid catalyst and its application in the acetalization of glycerol: crystal structure of -5-hydroxy-2-phenyl-1,3-dioxane trimer. Chem. Pap. 66(11), 1048–1058 (2012)
R. Gupta, S. Paul, R. Gupta, Covalently anchored sulfonic acid onto silica as an efficient and recoverable interphase catalyst for the synthesis of 3,4-dihydropyrimidinones/thiones. J. Mol. Catal. A Chem. 266, 50–54 (2007)
B. Karimi, M. Khalkhali, Solid Silica-based sulfonic acid as an efficient and recoverable interphase catalyst for selective tetrahydropyranylation of alcohols and phenols. J. Mol. Catal. A Chem. 232, 113–117 (2005)
E. Andrijanto, The Study of Heterogeneous Catalysts for Biodiesel Synthesis. Ph.D. Thesis. The University Of Huddersfield, October 2012
J.M. Marchetti, A.F. Errazu, Comparison of different heterogeneous catalysts and different alcohols for the esterification reaction of oleic acid. Fuel 87, 3477–3480 (2008)
A. Trusek-Holownia, A. Noworyta, An integrated process: ester synthesis in an enzymatic membrane reactor and water sorption. J. Biotechnol. 130, 47–56 (2007)
M. Jonh, C. Ramon, T. Mercè, Kinetic study of palmitic acid esterification catalyzed by rhizopus oryzae resting cells. Acta Biol. Colomb. 14(1), 161–172 (2009)
R. Goddard, J. Bosley, B. Al-Duri, Esterification of oleic acid and ethanol in plug flow (packed bed) reactor under supercritical conditions investigation of kinetics. J. Supercrit. Fluids 18, 121–130 (2000)
H. Zhao, Z. Song, J.V. Cowins, O. Olubajo, Microwave-assisted esterification of n-acetyl-l-phenylalanine using modified mukaiyama’s reagents: a new approach involving ionic liquids. Int. J. Mol. Sci. 9, 33–44 (2008)
K.G. Kabza, B.R. Chapados, J.E. Gestwicki, J.L. Mcgrath, Microwave-induced esterification using heterogeneous acid catalyst in a low dielectric constant medium. J. Org. Chem. 65, 1210–1214 (2000)
B. Karimi, H.M. Mirzaei, A. Mobaraki, Periodic mesoporous organosilica functionalized sulfonic acids as highly efficient and recyclable catalysts in biodiesel production. Catal. Sci. Technol. 2, 828–834 (2012)
K.A. Shah, J.K. Parikh, K.C. Maheria, Use of sulfonic acid-functionalized silica as catalyst for esterification of FFA in acid oil for biodiesel production: an optimization study. Res. Chem. Intermed. (2015). doi:10.1007/S11164-013-1253-6
E. Santacesaria, D. Gelosa, P. Danise, S. Carra, Vapor-phase esterification catalyzed by decationized y zeolites. J. Catal. 80, 427–436 (1983)
G.D. Yadav, M.B. Thathagar, Esterification of maleic acid with ethanol over cation-exchange resin catalysts. React. Funct. Polym. 52, 99–110 (2002)
A. Izci, F. Bodur, Liquid-phase esterification of acetic acid with isobutanol catalyzed by ion-exchange resins. React. Funct. Polym. 67, 1458–1464 (2007)
R. Gottipati, Preparation and characterization of microporous activated carbon from biomass and its application in the removal of chromium(VI) from aqueous phase, Ph. D. Theses, National Institute of Technology Rourkela, India, January 2012
A. Jurj, Study of biodiesel fuel production through enzymatic methods, Ph.D. Theses, Babes-Bolyai University Cluj-Napoca, 2011
R.S. Sirsam, G.A. Usmani, Preparation and characterization of sulfonic acid functionalized silica and its application for the esterification of ethanol and maleic Acid. J. Inst. Eng. Ser. E 97(1), 75–80 (2016)
V.P. Yadav, A.S.K. Maity, P. Biswas, R.K. Singh, Kinetics of esterification of ethylene glycol with acetic acid using cation exchange resin catalyst. Chem. Biochem. Eng. Q. 25(3), 359–366 (2011)
M. Zhang, W.S. Zhu, H.M. Li, H. Shi, Y.S. Yan, Z.G. Wang, Esterification of salicylic acid using Ce4+ modified cation-exchange resin as catalyst. J. Chil. Chem. Soc. 57(4), 1477–1481 (2012)
S.P. Chopade, Ion-exchange resin-catalyzed ketalization of acetone with 1,4- and 1,2-diols: use of molecular sieve in reactive distillation. React. Funct. Polym. 42, 201–212 (1999)
Y.T. Tang, Y.W. Chen, H.P. Huang, C.C. Yu, Design of reactive distillations for acetic acid esterification. AIChE J. 51(6), 1683–1699 (2005)
N.A. Pakhomov, R.A. Buyanov, Current trends in the improvement and development of catalyst preparation methods, kinetics and catalysis. Kinet. Katal. 46(5), 711–727 (2005)
Material extracted from http://nzic.org.nz/ChemProcesses/water/13D.pdf
Fundamentals of ion exchange: Dowex ion exchange resins, http://msdssearch.dow.com/PublishedLiteratureDOWCOM/dh_0032/0901b803800326ca.pdf?filepath=liquidseps/pdfs/noreg/177-01837.pdf&fromPage=GetDoc
S.P. Chopade, M.M. Sharma, Reaction of ethanol and formaldehyde: use of versatile cation-exchange resins as catalyst in batch reactors and reactive distillation columns. React. Funct. Polym. 32, 53–64 (1997)
A. Chakrabarti, M.M. Sharma, Cationic ion exchange resins as catalyst. React. Polym. 20, 1–45 (1993)
S.H. Ali, A. Tarakmah, S.Q. Merchant, T. Al-Sahhaf, Synthesis of esters: development of the rate expression for the Dowex 50 w8-400 catalyzed esterification of propionic acid with 1-propanol. Chem. Eng. Sci. 62, 3197–3217 (2007)
A.D. Davison, Synthesized sulfonic solid acid catalysts for liquid phase reactions, Doctoral thesis, University of Huddersfield (2008), http://eprints.hud.ac.uk/5014/
D. Vaičiukynienėa, V. Vaitkevičiusa, A. Kantautasb, V. Sasnauskasa, Utilization of by-product waste silica in concrete-based materials. Mater. Res. 15(4), 561–567 (2012)
M. Keppelera, N. Husing, Space-confined click reactions in hierarchically organized silica monoliths. New J. Chem. 35, 681–690 (2011)
Y.K. Lv, J.Q. Zhang, Y.D. He, J. Zhang, H.W. Sun, Adsorption-controlled preparation of molecularly imprinted hybrid composites for selective extraction of tetracycline residues from honey and milk. New J. Chem. 38, 802 (2014)
M. Bhagiyalakshmi, K. Shanmugapriya, M. Palanichamy, B. Arabindoo, V. Murugesan, Esterification of maleic anhydride with methanol over solid acid catalysts: a novel route for the production of hetero esters. Appl. Catal. A Gen. 267, 77–86 (2004)
J.U.K. Oubagaranadin, N. Sathyamurthy, Z.V.P. Murthy, Evaluation of fuller’s earth for the adsorption of mercury from aqueous solutions: a comparative study with activated carbon. J. Hazard. Mater. 142, 165–174 (2007)
H.D. Choi, M.C. Shin, D.H. Kim, C.S. Jeon, K. Baek, Removal characteristics of reactive black 5 using surfactant-modified activated carbon. Desalination 223, 290–298 (2008)
A.A. Kiss, A.C. Dimian, G. Rothenberg, in Biodiesel Production by Integrated Reactive-Separation Design 17th European Symposium On Computer Aided Process Engineering—ESCAPE17V, ed. by Plesu, P.S. Agachi
A.A. Kiss, F. Omota, A.C. Dimian, G. Rothenberg, The heterogeneous advantage: biodiesel by catalytic reactive distillation. Top. Catal. 40(1–4), 141–150 (2006)
J. Lemic, M.T.C. Anovic, M. Djuricic, T. Stanic, Surface modification of sepiolite with quaternary amines. J. Colloid Interface Sci. 292, 11–19 (2005)
M.C. Costache, M.J. Heidecker, E. Manias, R.K. Gupta, C.A. Wilkie, Benzimidazolium surfactants for modification of clays for use with styrenic polymers. Polym. Degrad. Stab. 92, 1753–1762 (2007)
M. Lezeharia, J.P. Baslya, M. Baudua, O. Bouras, Alginate encapsulated pillared clays: removal of a neutral/anionic biocide (pentachlorophenol) and a cationic dye (safranine) from aqueous solutions. Colloids Surf. A Physicochem. Eng. Asp. 366, 88–94 (2010)
Q. Zhoua, H.P. Hea, J.X. Zhua, W. Shen, R.L. Frost, P. Yuan, A mechanism of p-nitrophenol adsorption from aqueous solution by HDTMA+-pillared montmorillonite—implications for water purification. J. Hazard. Mater. 154, 1025–1032 (2008)
C. Blachier, L. Michot, I. Bihannic, O. Barrès, A. Jacquet, M. Mosquet, Adsorption of polyamine on clay minerals. J. Colloid Interface Sci. 336, 599–606 (2009)
R. Zhua, L. Zhua, J. Zhua, L. Xua, Structure of surfactant–clay complexes and their sorptive characteristics toward hocs. Sep. Purif. Technol. 63, 156–162 (2008)
S.Z. Li, P.X. Wua, Characterization of sodium dodecyl sulfate modified iron pillared montmorillonite and its application for the removal of aqueous Cu(II) And Co(II). J. Hazard. Mater. 173, 62–70 (2010)
E. Eren, O. Cubuk, H. Ciftci, B. Eren, B. Caglar, Adsorption of basic dye from aqueous solutions by modified sepiolite: equilibrium, kinetics and thermodynamics study. Desalination 252, 88–96 (2010)
A. Özcan, Ç. Ömeroğlu, Y. Erdoğan, A.S. Özcan, Modification of bentonite with a cationic surfactant: an adsorption study of textile dye reactive blue 19. J. Hazard. Mater. 140, 173–179 (2007)
Y. Chen, C. Zhu, Y. Sun, H. Duan, W. Ye, D. Wu, Adsorption of La(III) onto GMZ bentonite: effect of contact time, bentonite content, pH value and ionic strength. J. Radioanal. Nucl. Chem. 292, 1339–1347 (2012)
E. Klumppa, C. Contreras-Ortega, P. Klahre, F.J. Tino, S. Yapar, C. Portillo, S. Stegen, F. Queirolo, M.J. Schwuger, Sorption of 2,4-dichlorophenol on modified hydrotalcites. Colloids Surf. A Physicochem. Eng. Asp. 230, 111–116 (2004)
S. Kolemen, N.B. Acarali, N. Tugrul, E. Moroydor, E.M. Derun, S. Piskin, The zinc adsorption study by using orhaneli fly ash, bentonite, and molasses in wastewater. Water Air Soil Pollut. 224, 1367 (2013)
P. Chutia, S. Kato, T. Kojima, S. Satokawa, Adsorption of As(V) on surfactant-modified natural zeolites. J. Hazard. Mater. 162, 204–211 (2009)
L. Zhou, H. Chena, X. Jiang, F. Lua, Y. Zhou, W. Yin, X. Ji, Modification of montmorillonite surfaces using a novel class of cationic gemini surfactants. J. Colloid Interface Sci. 332, 16–21 (2009)
S. Veli, B. Alyuz, Adsorption of copper and zinc from aqueous solutions by using natural clay. J. Hazard. Mater. 149, 226–233 (2007)
Z. Bouberka, S. Kacha, M. Kameche, S. Elmaleh, Z. Derriche, Sorption study of an acid dye from an aqueous solutions using modified clays. J. Hazard. Mater. B119, 117–124 (2005)
Z. Bouberka, A. Khenifi, H. Ait Mahamed, B. Haddou, N. Belkaid, N. Bettahar, Z. Derriche, Adsorption of supranol yellow 4 GL from aqueous solution by surfactant-treated aluminum/chromium-intercalated bentonite. J. Hazard. Mater. 162, 378–385 (2009)
Q. Li, Q.Y. Yue, Y. Sub, B.Y. Gaoa, J. Li, Two-step kinetic study on the adsorption and desorption of reactive dyes at cationic polymer/bentonite. J. Hazard. Mater. 165, 1170–1178 (2009)
G. Ozdemir, S. Yapar, Adsorption and desorption behavior of copper ions on Na-montmorillonite: effect of rhamnolipids and pH. J. Hazard. Mater. 166, 1307–1313 (2009)
L. Wanga, A. Wanga, Adsorption properties of congo red from aqueous solution onto surfactant-modified montmorillonite. J. Hazard. Mater. 160, 173–180 (2008)
Q. Li, Q.Y. Yue, Y. Sub, B.Y. Gao, L. Fu, Cationic polyelectrolyte/bentonite prepared by ultrasonic technique and its use as adsorbent for reactive Blue K-GL Dye. J. Hazard. Mater. 147, 370–380 (2007)
A.L. Poli, T. Batista, C.C. Schmitt, F. Gessner, M.G. Neumann, Effect of sonication on the particle size of montmorillonite clays. J. Colloid Interface Sci. 325, 386–390 (2008)
B. Zohra, K. Aicha, S. Fatima, B. Nourredine, D. Zoubir, Adsorption of direct red 2 on bentonite modified by cetyltrimethylammonium bromide. Chem. Eng. J. 136, 295–305 (2008)
A.S. Özcan, B. Erdem, A. Özcan, Adsorption of acid blue 193 from aqueous solutions onto Na–bentonite And DTMA–Bentonite. J. Colloid Interface Sci. 280, 44–54 (2004)
U.F. Alkaram, A.A. Mukhlis, A.H. Al-Dujaili, The removal of phenol from aqueous solutions by adsorption using surfactant-modified bentonite and kaolinite. J. Hazard. Mater. 169, 324–332 (2009)
J. Ma, L. Zhu, Simultaneous sorption of phosphate and phenanthrene to inorgano–organo-bentonite from water. J. Hazard. Mater. 136, 982–988 (2006)
Q. Zhou, H.P. Hea, J.X. Zhu, W. Shen, R.L. Frost, P. Yuan, Mechanism of p-nitrophenol adsorption from aqueous solution by HDTMA+-pillared montmorillonite—implications for water purification. J. Hazard. Mater. 154, 1025–1032 (2008)
H.B. Senturka, D. Ozdesa, A. Gundogdua, C. Durana, M. Soylak, Removal of phenol from aqueous solutions by adsorption onto organomodified Tirebolu bentonite: equilibrium, kinetic and thermodynamic study. J. Hazard. Mater. 172, 353–362 (2009)
Y.Z. Yao, S. Kawi, Surfactant-treated K10 montmorillonite: a high-surface-area clay catalyst. J. Porous Mater. 6, 77–85 (1999)
W. Cheng, S.H.I. Huisheng, L.I. Yan (2012) Preparation of bentonite supported nano titanium dioxide photocatalysts by electrostatic self-assembly method. J. Wuhan Univ. Technol. Mater. Sci. Ed. 27(4), 603–607 (2012)
M. Galambos, A. Krajňák, O. Rosskopfová, E. Viglašová, R. Adamcová, P. Rajec, Adsorption equilibrium and kinetic studies of strontium on Mg–bentonite, Fe–bentonite and illite/smectite. J. Radioanal. Nucl. Chem. 298, 1031–1040 (2013)
C.D. Nunes, J. Pires, A.P. Carvalho, M.J. Calhorda, P. Ferreira, Synthesis and characterization of organo-silica hydrophobic clay heterostructures for volatile organic compounds removal. Microporous Mesoporous Mater. 111, 612–619 (2008)
Acknowledgments
Authors are thankful to TEQIP-II, Ministry of Human Resource Development, Government of India, New Delhi, for providing facilities to write the review paper.
Author information
Authors and Affiliations
Corresponding author
Rights and permissions
About this article
Cite this article
Sirsam, R., Hansora, D. & Usmani, G.A. A Mini-Review on Solid Acid Catalysts for Esterification Reactions. J. Inst. Eng. India Ser. E 97, 167–181 (2016). https://doi.org/10.1007/s40034-016-0078-4
Received:
Accepted:
Published:
Issue Date:
DOI: https://doi.org/10.1007/s40034-016-0078-4