Abstract
Surface cross-linking of acrylic-based water absorbent particles was conducted to enhance swollen gel strength. Partially neutralized acrylic acid was synthesized via solution polymerization, and then modification of superabsorbent polymer was imposed on the surface of its particles using bisphenol A diethylene glycidyl ether (BADGE) and 3,4-epoxycyclohexylmethyl-3,4-epoxycyclohexane carboxylate (CAE) resins. The two-step surface treatment was conducted initially, by homogenous soaking in treatment solution containing cross-linker and solvent (90 wt% acetone and 10 wt% deionized water) and then heating at 170 °C, to complete reaction, subsequently. ATR-FTIR spectroscopy, rheometry analysis, swelling capacity measurements in deionized water and saline solution (0.9 wt% NaCl) and swelling kinetics measurments were also employed for accurate investigation. Effect of both epoxy resins content as the effective parameter has been examined. Addition of a little amount of cross-linker raised the storage modulus about 30 % but the higher amounts of the reagents increased it up to 100 %. Both resins could significantly improve the absorbency under load. Heating duration was another effective parameter which has been investigated for BADGE resin. Two different trends have been observed for variant heating intervals. Network structure has been revealed by means of average M c calculating by the modified rubber elasticity theory. Besides, swelling kinetics of BADGE-treated sample was approximated by employing Voigt-based viscoelastic model and its parameters have been derived and compared with diethylene glycol diglycidyl ether-treated sample, as well.
Similar content being viewed by others
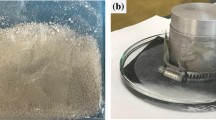
Explore related subjects
Discover the latest articles, news and stories from top researchers in related subjects.Avoid common mistakes on your manuscript.
Introduction
Superabsorbent polymers as hydrophilic light cross-linked three-dimensional networks are capable of absorbing and retaining deionized water, up to 1000 times of their weight [1]. Their vast variety of applications in different fields, mainly in hygienic [2] and non-hygienic [2, 3] have made them immensely popular. The manufacturing capacity of superabsorbent polymers is about 2.2 million tons per year and still there is a strong demand to raise this capacity [4]. Therefore, superabsorbent polymer is an attractive field to conduct a research.
Strength of swollen gel is of great importance comparing with its other features. Various strategies such as preparation of cyclic structure formation [5], interpenetrating polymer networks [6–8], composite hydrogels [9–11], nanocomposite superabsorbent polymers [8, 11] and surface cross-linked superabsorbent polymers [12–14] have been utilized to enhance this characteristic. Except surface cross-linking of conventional superabsorbent which is mainly cited in patents, the other techniques have been probed quite thoroughly. Recently, self-cross-linking ability of N,N-dimethylacrylamide (DMAA) has been resulted in both superior mechanical properties and free absorbency, as well [15].
Surface treatment increases cross-link density in the outer most layers, which results in higher modulus, and consequently higher absorption under loud (AUL) [1, 16]. Additionally, the high-density shell could maintain the shape of swollen superabsorbent particle and prevents gel blockage [1, 17]. Surface cross-linking has been investigated in controlling the drug diffusion, as well as, the initial burst release [18, 19].
Although, there are numerous studies in synthesis and characterization of superabsorbent polymers, only few of them have been aimed surface cross-linking of the superabsorbent particles and interfered its parameters [20–22] but main challenges have been still remained. Ma et al. [20] prepared SAP via solution polymerization. The SAP particles were surface cross-linked afterward, using ethylene glycol diglycidyl ether and modified with inorganic salt to achieve high-saline solution absorbency. In two other reports, in situ surface cross-linking carried out via inverse suspension polymerization by divinyl benzene (hydrophobic reagent) [21] and bis (methacryloylamino)-azobenzene [22]. Their effects on the swelling properties and SAP morphology were investigated [22]. In another observation [17], the assets of photo-induced surface cross-linking versus thermal induction was also explored. However, effects of cross-linker type and other important features have not been investigated, particularly.
Bisphenol A diglycidyl ether (BADGE) and cycloaliphatic diepoxide (CAE) resins could be appealing candidates to run condensation reaction [23, 24]. The strong affinity between the carboxylic acid pendant group of acrylic acid and the glycidyl group in an intermediate temperature [25] provides epoxy groups as cross-linking agents with favorite quality. Along with that BADGE resin is one of the most commercially used resins mainly for coating with great properties [23]. CAE resin is also a promising resin which has lower viscosity, higher photo stability after curing, and greater compressive strength compared to BADGE [26, 27]. To the best of our knowledge, these resins have not been used for surface treatment of conventional acrylic-based superabsorbent, despite their spread use in coating technology for cross-linking of acrylic latex [23, 25].
In this study, at first superabsorbent polymer was synthesized via solution polymerization, then the surface cross-linking of SAP particles was carried out using treatment solution which contained acetone (90 wt%) and deionized water (10 wt%) as solvent and epoxy resins as solutes. The reaction between functional groups was proved by ATR-FTIR spectroscopy. The effects of type and concentration of cross-linker in the treatment solution and curing time have been examined using rheometry analysis of swollen gel, in addition to equilibrium swelling capacity measurements in saline solution and deionized water. Network structure was analyzed by theory of modified rubber elasticity. Finally swelling kinetics of samples treated by BADGE resin was evaluated, using Voigt-based model and compared with the diethylene glycol diglycidyl ether (DEGDGE)-treated sample in the presence of a non-modified gel as control. The swelling kinetic parameters have been estimated, as well.
Experimental
Materials
Acrylic acid (AA) as monomer, ammonium persulfate (APS) and tetramethyl ethylene diamine (TMEDA) as an efficient initiator pair and sodium hydroxide (NaOH) for neutralization procedure were provided from Merck, Germany. Polyethylene glycol diacrylate (PEGDA, M w: 400 g/mol) as an interior cross-linker was supplied from Rahn, Switzerland. Bisphenol A diglycidyl ether resin (BADGE; EEW: 185–196 g/eq, EPIRAN-06, Iran) and 3,4-epoxycyclohexylmethyl 3,4-epoxycyclohexane carboxylate (CAE, weight per epoxide: 126–135 g, Zhangxin Chemical Co., China), and acetone (Merck, Germany) were utilized as surface cross-linking agents and solvent, respectively. Sodium chloride (Merck, Germany) solution and deionized water for swelling media were provided as well. All materials were used as received.
Synthesis of superabsorbent
The cross-linked partially neutralized poly(acrylic acid) was synthesized by solution polymerization using APS and TMEDA as an initiating pair [28]. At first, acrylic acid (15 g) was neutralized by NaOH solution (6.25 g NaOH in 28 mL of deionized water) at low-temperature conditions (provided by an ice water bath). The acrylic acid was neutralized about 75 % to form sodium acrylate. On the next step, prepared solutions of TMEDA (0.1 g in 2 mL deionized water), APS (0.2 g in 2 mL deionized water), and PEGDA (0.01 g in 2 mL deionized water) were added to partially neutralized acrylic acid while the solution was magnet stirred. After a certain time, gelation occurred and then the sample was placed in 90 °C air circulated oven for 6 h. Finally, the dried gel was ground by a mini hammer grinder.
Surface treatment
Preferred amount of cross-linking agent dissolved in a solution composed of acetone (90 wt%) and water (10 wt%) and called the treatment solution. In the next step, the dried SAP particles were soaked in the treatment solution. The total weight of treatment solution for every sample was a fixed amount (i.e., 20 g); thus, the weight percentage of each component could be changed by reagent content variation. The concentration of SAP particles in solvent was constant (1 g SAP in 20 g treatment solution). The particles were incubated at room temperature for 30 min. Then, the filtered particles were cured at 170 °C for certain time.
Effect of cross-linker concentration has been explored by BADGE and CAE (BA and CA series), at 170 °C for 3 h, whereas time effect on SAP properties was examined only using BADGE in two concentrations (0.5 and 0.05 g in 20 g of treatment solution). Overall procedure of this work has been presented in Scheme 1.
ATR-FTIR characterization
The FTIR spectra were taken on Bruker Instruments (ABB-Bomem MB-100, Germany). The ATR-FTIR (Specac, Golden-gate, UK) were employed to probe the surface of the superabsorbent particles. They were obtained at wavenumber range of 600–4000 cm−1 and resolution of 1 cm−1.
Free swelling measurements
Swelling capacity was measured using sieve method [2]. The powdered samples (0.1 g) were permitted to swell in 100 mL deionized water (DW) or in saline solution (0.9 wt% NaCl) at room temperature. The swollen particles were separated by sieve and a sponge was applied to dry the remained aqueous. Then, swelling capacity in g/g was calculated through Eq. (1) as follows:
where W d and W s stand for weights of initial dry sample and swollen SAP sample, respectively.
Saline-absorbency under load
Weighed dried SAP sample (0.5 g) was uniformly dispersed on the surface of polyester gauze which had been located on a macro-porous sintered glass filter plate placed in a Petri dish [2]. A cylindrical solid load (Teflon, d = 60 mm) was put on the dry SAP particles while it could be freely slipped in a glass cylinder. Desired load (applied pressure 0.3 psi) was placed on the sample. Then, 0.9 wt% saline solution was added to the Petri dish. After 30 min, the maximally swollen particles were removed and re-weighed. Absorbency under load (AUL) value was calculated by Eq. (1), as well.
Swelling kinetics measurments
To measure the swelling rate, 0.100 g of dried polymer (mesh 35–100) was dispersed in 100 mL of deionized water under well-stirring condition (vortex has been made). The swelling capacity was measured by Eq. (1) at definite intervals to draw a profile of swelling against time for individual samples.
Rheological analysis
A Paar-Physical oscillatory rheometer (MCR 300, Germany) was utilized to perform the rheological measurements of samples at 25 °C with parallel plate geometry (plate diameter of 25 mm, gap of 3 mm) [2]. The storage modulus (G′) was recorded as a function of angular frequency at constant shear strain 0.2 % (to be in linear viscoelastic zone). All samples were scaled (0.2 g), then they swelled in either 5 mL deionized water or 3 mL saline solution (0.9 wt%).
Results and discussion
ATR-FTIR
The surface treatment was executed using BADGE and CAE resins. The outline of reaction is shown in Scheme 2. The glycidyl groups of both epoxy resins have the affinity to react with the carboxylic pendant groups of superabsorbent [25]. The order of reactivity for CAE resin is directly opposite to that of BADGE resin, which is; carboxylic acid > alcohols > amines [24]. In the majority of epoxy resin reactions, several different catalysts, e.g., bases, metal ions and Lewis acid were added. In this special surface treatment, no extra catalyst was added. In fact, carboxylate anions produced in neutralization process were the catalyst that raises the selectivity of reaction routes in Scheme 2 at intermediate temperature [25, 27, 29].
Surface cross-linking provided a shell-like area [1] with higher cross-link density as shown in Scheme 1. To prove the reaction in both cases, ATR-FTIR was employed, as it is an accurate technique for surface characterization. Figure 1 illustrates ATR-FTIR spectra of the unmodified superabsorbent sample and those treated by BADGE and CAE.
As shown in Scheme 2a, treatment by BADGE introduced aromatic groups to the surface of the sample, resulting in the significant changes in FTIR spectra (Fig. 1 spectrum b) which were possibly associated with the aromatic groups. The band at 1509 cm−1 is related to the aromatic groups of the surface cross-linker and the other bands (1607, 1580 cm−1) have overlapped with carboxyl bands at 1563 and 1684 cm−1 [30]. At wavenumbers higher than 3000 cm−1, the hydroxyl band shifted from 3459 to 3513 cm−1 on account of reduction in dimeric and oligomeric hydrogen-bonded carboxylic acid [31] and formation of weaker hydrogen-bonded alcohols. For cycloaliphatic epoxy resin, CAE, (Fig. 1 spectrum c; Scheme 2b) the same shifting occured from 3459 to 3536 cm−1 for the hydroxyl groups which have been formed on the cyclohexane rings.
Goswami et al. [32] reported that the carbonyl group band of CAE appeared at 1725 cm−1. Presence of this group has broadened and made a double-peak band at 1685 cm−1. Furthermore, at fingerprint region, the ether band of CAE has appeared at 1100 cm−1 [33] and this alteration has changed the spectra of this region in FTIR spectrum (Fig. 1 spectrum c). Based on these results, the treatments were successfully carried out.
Rheometry and swelling analysis
Theory
The dynamic oscillatory rheometry is one of the most reliable techniques to measure modulus especially for rubber-like particles. Providing a linear viscoelastic zone of the SAP, the correct complex shear modulus G * will be obtained (it is independent of applied strain) and is defined according to Eq. (2) as follows [34]:
where G′ and G″ are storage and loss moduli, respectively [35]. Elastic modulus (G′) provides useful information about strength of swollen superabsorbent, and is advantageous to estimate cross-link density and chain molecular weights between each two cross-links (M c) [1, 34, 35]. The elastic modulus of gel (G′) in a homogenous network of Gaussian chains is related to M c and cross-link density [calculated by Eq. (3)] through Eq. (4) as follows [1, 35]:
where T, R and ρ are the absolute temperature, the gas constant and the density of polymer, respectively (ρ = 1.41 g/cm3) [33]. The front factor A equals 1–2/φ for phantom network where φ is the functionality of polymer (here φ = 4). Value of \(\upsilon_{2}^{0}\) is the volume fraction of polymer during preparation and υ 2 is the volume fraction of polymer at which the measurement of shear modulus is made [for Eq. (4)] which is determined as follows [36]:
where V r is the molar volume of the polymer repeat unit (V r = 47.5 mL/mol) and C 0 (mol/L) is initial molar concentration of the monomer [37].
Effect of epoxy resin content
In this study, the two different epoxy resins, CAE and BADGE, have been used in surface cross-linking process. It should be noted that the modified samples have both interior (PEGDA) and exterior (CAE and BADGE) cross-linkings (Scheme 1). The interior cross-linking was the one which is in typical SAP providing high free absorbency but low absorbency under load (AUL) whereas the exterior cross-linking created a highly cross-linked area around the SAP particles. It can increase deformation stability and AUL value (a practical value), as well.
All the samples were prepared by homogenous soaking in the treatment solution where the ratio of acetone (90 wt%) to water (10 wt%) was kept constant. Acetone was chosen due to its low solubility parameter [δ acetone = 10 (cal/g3)1/2] which is useful to retain the network collapse [38]. Solute movement through hydrogel primarily depends on the macromolecular mesh size of the polymer network (M c), polymer chain mobility, radius of the solute and its binding to polymer in the case of charged polymer [39].
Since acetone to water ratio provided really small absorbency close to zero and lots of physical cross-links due to entanglements made by SAP particles similar to hydrogel, in this case hydrogel approximation was appropriate. Thus, the depth of reagent penetration was controlled. In addition, solubility parameter of mixture [δ mix = 11.056 (cal/g3)1/2] was much lower than critical solubility parameter [δ c = 17.5 (cal/g3)1/2] in which network collapsed. Moreover, acetone has a great ability to dissolve both epoxy resins in the presence of minute amount of water.
Table 1 describes the effect of BADGE resin content (BA series samples) on absorbency in deionized water and saline solution (0.9 wt% NaCl), the storage modulus (G′) in 1 rad/s and M c calculated using Eq. (4). As the epoxy resin content increased, the storage modulus rose and consequently average M c decreased. Additionally, the free swelling capacity can be decreased by both surface treatment and increase in the cross-linker content. Decline in free absorbency and upturn in storage modulus, generally indicates higher absorbency under load which is desired [1, 16, 17].
The same trend has been observed for CAE resin-treated samples (CA series) in Table 2. The cross-linked samples by CAE resin had more absorbency compared to BADGE resin-treated samples. This discrepancy was more obvious in higher contents of cross-linking agents. It should be mentioned that BADGE treatment solution had lower moles of reagent than CAE treatment solution but the full extent of available reagents do not participate in surface cross-linking reaction in soaking method. Furthermore, based on Flory-Rehner theory three main contributions including mixing (π mix), elastic (π el) and ionic (π ion) are interfering in the equilibrium swelling of SAP particles [40]:
In the case of swelling in deionized water, the ionic part contribution is the same for the whole samples because of equal degree of neutralization. Thus, the crucial parameters are network conformation and polymer–solvent interaction. In fact, storage modulus is an approach to reveal the network structure and investigate elastic contribution. Here, the elastic modulus at low frequencies has been considered owing to contribution of only chemical cross-links in modulus measurement.
It has been observed that the storage modulus of the samples treated by BADGE were higher than those of the treated ones by CAE (at the same concentration) despite the presence of more moles of CAE in treatment solution and higher affinity for carboxylic acid groups compared to BADGE. Hence, the cross-link density of samples in the CA series was lower than the BA series.
Comparing the above two cases with DEGDGE-treated samples (DE series), it has been elicited that the DE series showed higher modulus with higher absorbency even at low concentrations. For instance, presence of only 0.25 g of DEGDGE in treatment solution increased the storage modulus values (at 1 rad/s) from 578 to 1350 Pa while the absorbency for deionized water and saline solution were 178.49 and 44.35 (g/g), respectively. The point is that even the highest amount of epoxy resins did not cause the lowest storage modulus for DEGDGE. In this specific case, it had higher cross-link density and consequently higher diffusion of DEGDGE molecules into the outermost layers of the particle. Therefore, diffusion is of great importance in adjustment of modulus values and strength of gel.
Amsden [39] has reported that for diffusion of solute into the hydrogels, Cukier model is the most fitted one. In this model, volume fraction of polymer (by the power of 0.75) and radius of solute are related exponentially to normalized diffusion coefficient. Different cross-linker types could affect the radius of solute and polymer–solvent coefficient which is constant in this model. Besides, the permeability could be expressed in terms of solubility and diffusivity. Thus, DEGDGE molecules could permeate easier than BADGE and CAE molecules due to lower radius and water miscibility.
But what about BADGE and CAE molecules? CAE molecules are smaller than BADGE ones; thus, they displayed less contribution in cross-linking reaction. Therefore, steric hindrance of the functional groups is also important which has not been regarded in the Cukier model. Moreover, length of cross-linker could be an inhibitive factor due to larger radius whereas the larger molecules could raise the possibility of finding adjacent carboxylic acid groups to run condensation reaction and, in consequence, certain length of cross-linker could prevent the creation of free dangling groups.
Since elastic contribution had negative effect on swelling, it is clear that all the samples faced decrease in absorbency but decrease levels were not the same for all series. Furthermore, the π mix had an influence on swelling; either positive or negative depends on polymer–solvent interactions [40]. In fact, the prepared polymers were copolymers of the introduced groups; as a result, the less water miscibility of BADGE and CAE compared to DEGDGE had resulted in more reduction in absorbency of BA and CA series.
Despite decrease in free absorbency, the absorbency under load (AUL) values for both series 50–100 % increased (Fig. 2). The highest value of AUL at optimum concentration for both reagents illustrated that the balance between absorbency and gel strength was strongly desired in real situations and applications. Moreover, the CAE resin could enhance the AUL much better than BADGE due to reasonable degree of cross-linking density at shell-like area.
According to the above discussions, various parameters exert an influence on absorbency and swollen gel strength, such as different mole fraction, distance between functional groups, the steric hindrance of functional groups in the reactions, the water solubility, polarity and the ability of hydrogen bond formation [36]. Furthermore, formation of osmotic passive areas may affect the absorbency in the same manner [41].
Figure 3 shows a comparison between the storage modulus variation of the same samples swollen in deionized water and saline solution for both epoxy resins in terms of angular frequency. The only difference between them was their concentration in water and saline solution. The storage modulus in saline solution, despite of lower level of solvent, was slightly lower than the storage modulus in deionized water. Besides, at higher frequencies, the incline of storage modulus of swollen SAP in deionized water was higher than that in saline solution.
In oscillatory rheometry, the relation between the angular frequency and the effect of physical cross-links on storage modulus is linear [34]. Ion pairs in polyelectrolyte attract each other due to dipole–dipole interactions and shape multiplets. Therefore, they act as additional (physical) cross-links in superabsorbent [40, 41]. This effect along with the presence of the free ends may contribute in the sharp decline in deionized water absorbency. However, these coulombic interactions between charged monomers could be screened by both counter and salt ions [42]. In rheometry of the swollen SAP by saline, maybe the presence of salt ions screen dipole–dipole interactions and consequentially reduced physical cross-link density.
Effect of reaction time
Effect of time on surface cross-linking using BADGE resin at two different contents, 0.5 (BA0.5) and 0.05 g (BA0.05) was investigated by the swelling ratio and the storage modulus as a function of angular frequency. The swelling capacity alterations of the surface-treated superabsorbent in deionized water and saline solution (0.9 wt% NaCl) for BA0.5 and BA0.05 in terms of the curing periods are depicted in Fig. 4. The deionized water absorbencies for both samples decreased about 120 g/g during first 90 min whereas on the next 90 min, the water absorbencies declined only about 50 and 15 g/g for BA0.5 and BA0.05, respectively.
Figure 5 describes the storage modulus of the swollen gel in deionized water of the latter samples as a function of angular frequency. The main increase in storage modulus occurred after 90 min and that increment was more obvious at higher content of reagents while the main reduction in absorbency occurred sometime earlier than 90 min. If cyclization and anhydride formation during heating resulted in higher storage modulus, the same trend should have happened, whereas the increment level in G′ for higher content of BADGE (Fig. 5a) was more significant than that for the lower content (Fig. 5b). As a result, the cross-linking reaction has given rise to higher modulus.
Introduction of BADGE reduced the swelling capacity (Fig. 4) due to less water miscibility at the first 90 min. However, cross-linking reactions had not been done thoroughly and there were still some free dangling groups and modulus value did not meet higher level. After 90 min, the cross-linking reaction (Scheme 2) was completed. Besides, in final intervals T g of SAP rose drastically and molecular diffusion was more prohibited; thus, even formed hydroxyl groups (Scheme 2) may contribute in condensation reaction and raised the modulus further [24].
Swelling kinetics
Swelling rate was another dominant factor for SAPs, which controlled the flow of fluid between particles and avoided gel blockage in cross-linked SAP. In fact, higher swelling rate increased the possibility of gel blockage because it narrowed down liquid convection and diffusion mechanisms to diffusion only, during swelling [17]. Swelling kinetics of surface cross-linked and unmodified SAP particles was examined by means of Voigt-based viscoelastic model as follows [43]:
Where S t is the amount of swelling (g/g) as a function of time, the equilibrium swelling capacity S e is the swelling at infinite time or maximum water-holding capacity, and the rate parameter r is the time required to reach 0.63 S e which is S r . The swelling kinetics variation is shown in Fig. 6 and amount of r, S e, and swelling rate (SR = S r /r) are presented in Table 3.
According to Fig. 6, the rate of swelling of untreated sample which was calculated by curve fitting, was 1.37 g g−1s−1, whereas for the treated gels using DEGDGE and BADGE were 0.79 and 0.664 g g−1s−1, respectively. This observation correlates with the higher cross-linking density on the shell and lower diffusion of solution into the SAP particles. It should be mentioned that the standard method [43] used for measurement (applying vortex during swelling by high-speed stirring) omitted the gel blockage effect and the pure swelling rates were measured.
Conclusion
The superabsorbent polymer was prepared via solution polymerization of partially neutralized acrylic acid and dried at 90 °C for 6 h [28]. Subsequently, the surface modification was carried out using treatment solution including deionized water, acetone and cross-linking agents (BADGE or CAE), then the particles were cured at 170 °C for 3 h. Increase in cross-linker content led to higher storage modulus and subsequently higher absorbency under load value which was coincided with lower free absorbency. These available and inexpensive cross-linkers could significantly enhance the gel strength about 100 and 88 % for BADGE and CAE, respectively. BADGE cross-linker has yielded elevated storage modulus and higher absorbency in contrast with the CAE reagent, despite stronger affinity of CAE for carboxylic groups and higher amounts of available moles at the same concentration. Curing duration led to various levels of changes in elastic modulus and swelling capacity values. Swelling kinetics parameters had been estimated based on Voigt-based viscoelastic model. The BADGE-treated samples had the lowest swelling rate, 0.664 g g−1s−1 which could prohibit gel blockage phenomenon. To conclude, steric hindrance, length and water miscibility of cross-linker could be considered as decisive factors.
References
Buchholz FL, Graham AT (1998) Modern superabsorbent polymer technology. Wiley Sons, New York
Zohuriaan-Mehr MJ, Kabiri K (2008) Superabsorbent polymer materials: a review. Iran Polym J 17:451–477
Zohuriaan-Mehr MJ, Omidian H, Doroudiani S, Kabiri K (2010) Advances in non-hygienic applications of superabsorbent hydrogel materials. J Mater Sci 45:5711–5735
http://www.nonwovens-industry.com/issues/2013-11-01/view_far-east-report/a-look-at-the-top-superabsorbent-polymer-makers-in-the-world/. Accessed 18 July 2014
Christenson EM, Anseth KS, Van den Beucken JJ, Chan CK, Ercan B, Jansen JA, Laurencin CT, Li WJ, Murugan R, Nair LS, Ramakrishna S, Tuan RS, Webster TJ, Mikos AG (2007) Nanobiomaterial applications in orthopedics. J Orthop Res 25:11–22
Myung D, Waters D, Wiscman M, Duhamel PE, Noolandi J, Ta CN, Frank CW (2008) Progress in the development of interpenetrating polymer network hydrogels. Polym Adv Technol 19:647–657
Peak CW, Nagar S, Watts RD, Schmidt G (2014) Robust and degradable hydrogels from poly (ethylene glycol) and semi-interpenetrating collagen. Macromolecules 47:6408–6417
Kheirabadi M, Bagheri R, Kabiri K (2015) Structure, swelling and mechanical behavior of a cationic full-IPN hydrogel reinforced with modified nanoclay. Iran Polym J 24:379–388
Li MC, Ge X, Cho UR (2013) Mechanical performance, water absorption behavior and biodegradability of poly (methyl methacrylate)-modified starch/SBR biocomposites. Macromol Res 21:793–800
Zhu H, Yao X (2013) Synthesis and characterization of poly (acrylamide-co-2-acrylamido-2-methylpropane sulfonic acid)/kaolin superabsorbent composite. J Macromol Sci Pure 50:175–184
Rodrigues FHA, Spagnol C, Pereira AGB, Martins AF, Fajardo AR, Rubira AF, Muniz EC (2014) Superabsorbent hydrogel composites with a focus on hydrogels containing nanofibers or nanowhiskers of cellulose and chitin. J Appl Polym Sci. doi:10.1002/APP.39725
Nogi K, Ishizaki K, Konishi K (2012) Polyacrylic acid (salt)-type water absorbent resin and method for producing of same. US Patent 0157650
Daniel T, Exner KM, Massonne K, Riegel U, Weismantel M (2007) Method for cross-linking hydrogels with morpholine-2,3-diones. US Patent 7183360
Flohr A (2010) Superabsorbent polymers having radiation activatable surface cross-linkers and method of making them. US Patent 7700663
Cipriano BH, Banik SJ, Sharma R, Rumore D, Hwang W, Briber RM, Raghavan SR (2014) Superabsorbent hydrogels that are robust and highly stretchable. Macromolecules 47:4445–4452
Ramazani-Harandi MJ, Zohuriaan-Mehr MJ, Yousefi AA, Ershad-Langroudi A, Kabiri K (2009) Effects of structural variables on AUL and rheological behavior of SAP gels. J Appl Polym Sci 113:3676–3686
Jockusch S, Turro NJ, Mitsukami Y, Matsumoto M, Iwamura T, Lindner T, Flohr A, di Massimo G (2009) Photoinduced surface cross-linking of superabsorbent polymer particles. J Appl Polym Sci 111:2163–2170
Thote AJ, Chappell JT Jr, Gupta RB, Kumar R (2005) Reduction in the initial-burst release by surface crosslink-king of PLGA microparticles containing hydrophilic or hydrophobic drugs. Drug Dev Ind Pharm 31:43–57
Huang X, Chestang BL, Brazel CS (2002) Minimization of initial burst in poly (vinyl alcohol) hydrogels by surface extraction and surface-preferential cross-linking. Int J Pharm 248:183–192
Ma S, Liu M, Chen Z (2004) Preparation and properties of a salt-resistant superabsorbent polymer. J Appl Polym Sci 93:2532–2541
Huang J, Huang ZM, Bao YZ, Weng ZX (2006) Synthesis and characterization of reinforced acrylic-based superabsorbents cross-linked with divinylbenzene. J Appl Polym Sci 100:1594–1600
Mudiyanselage TK, Neckers DC (2008) Highly absorbing superabsorbent polymer. J Appl Polym Sci 46:1357–1364
Wicks ZW, Jones FN, Pappas SP (1999) Organic coating science and technology, 2nd edn. Wiley, New York 11
Wu S, Soucek MD (2000) Cross-linking of acrylic latex coatings with cycloaliphatic diepoxide. Polymer 41:2017–2028
Shechter L, Wynstra J (1956) Glycidyl ether reactions with alcohols, phenols, carboxylic acids, and acid anhydrides. Ind Eng Chem 48:86–93
Wu S, Jorgensen JD, Soucek MD (2000) Synthesis of model acrylic latexes for cross-linking with cycloaliphatic diepoxides. Polymer 41:81–92
Teng G, Soucek MD (2001) Synthesis and characterization of cycloaliphatic diepoxide cross-linkable core-shell latexes. Polymer 42:2849–2862
Kabiri K, Hesarian S, Jamshidi A, Zohuriaan-Mehr MJ, Boohendi H, Poorheravi MR, Hashemi SA, Ahmad-Khanbeigi F (2011) Minimization of residual monomer content of superabsorbent hydrogels via alteration of initiating system. J Appl Polym Sci 120:2716–2723
Merfeld G, Molaison C, Koeniger R, Acar AE, Mordhorst S, Suriano J, Irwin P, Warner RS, Gray K, Smith M, Kovaleski K, Garrett G, Finley S, Meredith D, Spicer M, Naguy T (2005) Acid/epoxy reaction catalyst screening for low temperature (120 ◦C) powder coatings. Prog Org Coat 52:98–109
Nikolic G, Zlatkovic S, Cakic M, Cakic S, Lacnjevac C, Rajic Z (2010) Fast fourier transform IR characterization of epoxy GY systems cross-linked with aliphatic and cycloaliphatic EH polyamine adducts. Sensors 10:684–696
Dong J, Ozaki Y, Nakashima K (1997) Infrared, Raman, and near-infrared spectroscopic evidence for the coexistence of various hydrogen-bond forms in poly(acrylic acid). Macromolecules 30:1111–1117
Goswami TH, Nandan B, Alam S, Mathur GN (2003) A selective reaction of polyhydroxy fullerene with cycloaliphatic epoxy resin in designing ether connected epoxy star utilizing fullerene as a molecular core. Polymer 44:3209–3214
Pavia DL, Lampman GM, Kriz JS (1979) Introduction to spectroscopy: a guide for students of organic chemistry. WB Saunders Co., Philadelphia
Nielsen LE, Landel RF (1994) Mechanical properties of polymers and composites, vol 2. Marcel Dekker Inc, New York
Gundogan N, Melekaslan D, Okay O (2002) Rubber elasticity of poly (N-isopropylacrylamide) gels at various charge densities. Macromolecules 35:5616–5622
Es-haghi H, Bouhendi H, Bagheri-Marandi GH, Zohurian-Mehr MJ, Kabiri K (2012) Rheological properties of microgel prepared with long-chain crosslinkers by a precipitation polymerization method. J Macromol Sci Phys 51:880–896
Yazici I, Okay O (2005) Spatial inhomogeneity in poly (acrylic acid) hydrogels. Polymer 46:2595–2602
Chen J, Shen J (2000) Swelling behaviors of polyacrylate superabsorbent in the mixtures of water and hydrophilic solvents. J Appl Polym Sci 75:1331–1338
Amsden B (1998) Solute diffusion within hydrogels. Mechanisms and models. Macromolecules 31:8382–8395
Okay O, Duramz S (2002) Charge density dependence of elastic modulus of strong polyelectrolyte hydrogels. Polymer 43:1215–1221
Zeldovich KB, Khokhlov AR (1999) Osmotically active and passive counterions in inhomogeneous polymer gels. Macromolecules 32:3488–3494
Dobrynin AV, Colby RH, Rubinstein M (1995) Scaling theory of polyelectrolyte solutions. Macromolecules 28:1859–1871
Omidian H, Hashemi SA, Sammes PG, Meldrum I (1998) A model for the swelling of superabsorbent polymers. Polymer 39:6697–6704
Author information
Authors and Affiliations
Corresponding author
Rights and permissions
About this article
Cite this article
Moini, N., Kabiri, K. Effective parameters in surface cross-linking of acrylic-based water absorbent polymer particles using bisphenol A diethylene glycidyl ether and cycloaliphatic diepoxide. Iran Polym J 24, 977–987 (2015). https://doi.org/10.1007/s13726-015-0386-4
Received:
Accepted:
Published:
Issue Date:
DOI: https://doi.org/10.1007/s13726-015-0386-4