Abstract
The epithelial–mesenchymal transition (EMT) is a fundamental process governing morphogenesis in multicellular organisms and has recently been implicated in promoting carcinoma invasion and metastasis. Besides their therapeutic effects, accumulating evidences suggest that chemotherapeutic agents also induced EMT and enhanced the malignancy of treated cancer cells; however, the mechanism(s) still remains unclear. Here, we investigated the role of β-catenin signaling in doxorubicin (Dox)-induced EMT in human gastric cancer cell line BGC-823. We found that the transient treatment of Dox induced EMT and enhanced the in vitro migration ability of cancer cells. We also found that β-catenin signaling was activated upon Dox treatment. Inhibition of β-catenin by indomethacin (Indo) or siRNA suppressed Dox-induced EMT and decreased cancer cell migration ability. Our results showed that β-catenin signaling was critical to Dox-induced EMT. Indo and other β-catenin inhibitors may have a potential implication in prevention of gastric cancer metastasis.
Similar content being viewed by others
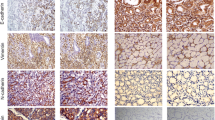
Avoid common mistakes on your manuscript.
Introduction
Metastasis is responsible for as much as 90 % of cancer-associated mortality, yet it remains the most poorly understood component of cancer pathogenesis [1]. During metastasis dissemination, cancer cells from a primary tumor locally invade the surrounding tissues, translocate through the lymph and blood systems to distant tissues, survive and adapt to the foreign microenvironment of these tissues, and finally form a macroscopic secondary tumor [2].
Epithelial–mesenchymal transition (EMT) is a process by which epithelial cells undergo dramatic morphology changes marked by loss of their polarity and cell–cell contacts [3]. The EMT process is vital for morphogenesis during embryonic development and tissue remodeling. Substantial evidences suggest that EMT also plays a critical role for tumor cells to leave an organized epithelial layer and gain the property of invasiveness. Such changes usually initiate metastasis in various types of human cancers [4, 5]. For example, pancreatic cancer with EMT features has more aggressive behaviors and is associated with poor patient survival [6]. Lung cancer cells resistant to gefitinib and erlotinib also display mesenchymal phonotype, suggesting that EMT might be a determinant of insensitivity to EGFR inhibition in lung cancer [7]. The molecular hallmarks for EMT are downregulation of epithelial cell adhesion molecules, such as E-cadherin, α-catenin, and γ-catenin, and upregulation of vimentin, fibronectin, α-smooth muscle actin, and other molecules of mesenchymal components. Diverse intra- and extracellular signals have been reported to induce EMTs in various carcinomas. In response to stimulation, transcription factors, such as Twist, Snail, Slug, ZEB1, and ZEB2, are induced to orchestrate EMT programs, which confer cancer cells the traits of drug resistance, self-renewal and invasiveness [8, 9].
The canonical Wnt/β-catenin signaling pathway has been linked with many cancers [10, 11]. In an unstimulated cell, endogenous β-catenin interacts with E-cadherin at membrane to mediate cell adhesion. Activated β-catenin translocates to the nucleus, where it interacts with lymphoid enhancer/T cell transcription factors (LEF/TCF) and regulates the expression of genes, including c-myc and cyclin D1 [12]. By binding to the promoter region of ZEB1, β-catenin induces ZEB1 expression and plays an important role in EMT [13]. Although the detailed mechanism by which the β-catenin signaling facilitates EMT is not well understood, it is believed that the regulation of components in cell adhesion and migration by complex with LEF/TCF is essential [14].
Besides their therapeutic effects, chemotherapeutic agents were also shown to enhance the malignancy of treated cancer cells [15]. Recently, EMT was reported to mediate anticancer drugs which induced cancer cell invasion [16, 17]. Here, we demonstrated that β-catenin signaling played a key role in doxorubicin (Dox)-induced EMT in human gastric cancer cells, and inhibition of β-catenin by siRNA or indomethacin (Indo), a selective β-catenin inhibitor, effectively suppressed this process.
Methods
Cell cultures and reagents
Human gastric cancer cell line BGC-823 was obtained from the Institute of Biochemistry and Cell Biology, Shanghai Institute for Biological Science, Chinese Academy of Sciences. Cells were maintained in RPMI 1640 containing penicillin (100 U/ml), streptomycin (100 mg/ml), and 10 % fetal bovine serum (FBS) at 37 °C in a humidified incubator supplemented with 5 % CO2 in air. Cell morphology was observed by light microscopy. Dox and Indo were purchased from Meilun Pharmaceutical (Dalian, China) and dissolved in double-distilled water (1.0 g/ml) and DMSO (10 M), respectively, for storage and diluted with phosphate-buffered saline (PBS) before use.
Primary antibodies used in this study included mouse monoclonal antibody to β-catenin (Santa Cruz, California, USA), mouse monoclonal antibody to β-actin (Abmart, Shanghai, China), rabbit polyclonal antibody to E-cadherin (Biosynthesis Biotechnology, Beijing, China), and rabbit polyclonal antibody to vimentin (Biosynthesis Biotechnology, Beijing, China).
Immunofluorescence
Cells were plated on sterilized coverslips in 24-well plates. After fixation with 4 % paraformaldehyde in PBS, cells were permeabilized in 0.1 % Triton X-100 and blocked with 0.5 % goat serum in PBS for 30 min at room temperature. After washing with PBS, the cells were incubated with specific primary antibodies at 4 °C overnight and washed with PBS. Then, the cells were incubated with FITC-conjugated secondary antibodies for 1 h at room temperature and stained with DAPI. The images were visualized with an Olympus microscope (Olympus IX71-F22FL/PH).
Western blotting
To prepare the whole-cell extract, cells were washed with PBS once and harvested by scraping them in RIPA lysis buffer. Protein content was determined by the Bradford assay (Beyotime Institute of Biotechnology, Haimen, China). The extracted proteins were separated in a 10 % SDS-polyacrylamide gel electrophoresis and transferred to PVDF membranes. The membranes were first blocked with 5 % (w/v) nonfat dry milk in TBST and then probed with the indicated primary antibodies with gentle shaking at 4 °C overnight. After washing four times, the membranes were incubated with the HRP-conjugated secondary antibodies for 1 h. The signals were detected using an enhanced chemiluminescence detection kit (Thermo Scientific, Illinois, USA).
RNA isolation and reverse transcription PCR
Total RNA was isolated using Trizol reagent (Invitrogen, California, USA) according to the manufacturer’s specifications and quantified by spectrophotometer measurement. RNA (2 μg) was reverse transcribed to cDNA with TIANScript M-MLV (Tiangen Biotech, Beijing, China) according to the manufacturer’s protocol. Primer pairs for human Twist, Snail, Slug, Lef1, c-myc, and glyceraldehyde 3-phosphate dehydrogenase (GAPDH) were as follows: Twist 5′-GGG AGT CCG CAG TCT TAC-3′ (sense) and 5′-CCT GTC TCG CTT TCT CTT T-3′ (antisense); Snail 5′-CAG ACC CAC TCA GAT GTC AA-3′ (sense) and 5′-CAT AGT TAG TCA CAC CTC GT-3′ (antisense); Slug 5′-GGT CAA GAA GCA TTT CAA C-3′ (sense) and 5′-GGT AAT GTG TGG GTC CGA-3′ (antisense); Lef1 5′-CGT GCA AAG CCA AGG CAG CG-3′ (sense) and 5′-AGC TGC CCC ACA GCC TGC TA-3′ (antisense); c-myc 5′-CGA CCC GGA CGA CGA GAC CT-3′ (sense) and 5′-GTT CGG GCT GCC GCT GTC TT-3′ (antisense); and GAPDH 5′-ACC ACA GTC CAT GCC ATC TC-3′ (sense) and 5′-TCC ACC ACC CTG TTG CTG TA-3′ (antisense). The products were separated in 2 % agarose gel. Real-time quantitative RT-PCR (qPCR) analyses were performed using SYBR Green-based detection (Tiangen Biotech, Beijing, China) according to the manufacturer’s protocol on a Rotor-Gene 3000 instrument (Corbett Life Science). The following primers were used for qPCR: β-catenin 5′-TGG TGC CCA GGG AGA ACC CC-3′ (sense) and 5′-TGT CAC CTG GAG GCA GCC CA-3′ (antisense); Twist 5′-TCA GCA GGG CCG GAG ACC TA-3′ (sense) and 5′-TCC ACG GGC CTG TCT CGC TT-3′ (antisense); and β-actin 5′-AGC CTC GCC TTT GCC GAT CC-3′ (sense) and 5′-ACA TGC CGG AGC CGT TGT CG-3′ (antisense). Relative expression of gene of interest was normalized to β-actin and calculated with 2−ΔΔCt method.
Cancer cell in vitro migration assays
The Transwell assay was performed as previously described [18]. Briefly, 2 × 103 cells suspended in RPMI 1640 containing 1.0 % FBS was added into the upper chambers, which were separated from the lower wells by 8-μm-pore-size polycarbonate membranes, and RPMI 1640 containing 10 % FBS was placed in the lower wells. After incubation for 48 h, the cells remained in the upper chamber were scratched carefully with cotton swabs and washed with PBS. The membrane was then fixed in methanol and stained with crystal violet dye. The stained areas were measured with Image J programme (National Institutes of Health, USA) and normalized to control groups. For wound-healing assay, 1 × 105 cells were plated into 6-well plates. When the monolayer reached confluence, a single scrape was made using a sterile pipette tip. The rate of wound closure was investigated by photographing 48 h later.
Inhibition of β-catenin expression by RNAi
The β-catenin siRNA oligonucleotides (sense 5′-AGC UGA UAU UGA UGG ACA Gtt-3′ and antisense 5′-CUG UCC AUC AAU AUC AGC Utt-3′) were synthesized from RiboBio (Guangzhou, China). A negative control siRNA (sense 5′-UGA AGG AGU UCC UGA UCU Utt-3′ and antisense 5′-AAG AUC AGG AAC UCC UUC Att-3′) was used as scramble siRNA. siRNA transfection was performed in the 6-well plates (Wuxi NEST Biotechnology Co., Ltd., China) using Lipofectamine 2000 (Invitrogen) according to the manufacturer’s instruction. Briefly, when the cells reached 50 % confluence, they were transfected with serum-free RPMI 1640 containing 50 nM scramble siRNA or β-catenin siRNA for 4 h, followed by recovery in medium containing serum and 0.1 μg/ml Dox. For wound-healing assay, the transfected cells were allowed to grow for 24 h in serum containing medium to reach 90 % confluence; the scrapes were made, and 0.1 μg/ml Dox was added.
Methylthiazol tetrazolium assay
Cells were plated into 96-well plates at 2,000 cells per well and allowed to adhere overnight. The culture was then replaced with fresh medium containing Dox and/or Indo. After 48 h, methylthiazol tetrazolium (MTT) was added into each well. The number of viable cells was determined by the OD value at 570 nm.
Statistical analysis
All experiments were repeated at least in triplicate. Data were reported as the mean ± SEM. Statistical analyses were performed using Student’s t test (P < 0.05; P < 0.01).
Results
Transient Dox treatment induced EMT in BGC-823 cells
To model the acute response of human gastric cancer cells to chemotherapeutic drug, we treated BGC-823 cells with 0.4 μg/ml Dox for 48 h, followed by growing in drug-free medium for another 24 h. After that, the drug survived cells (DSCs) showed a dramatic morphologic change. Normal BGC-823 cells had a cobblestone-like epithelial morphology. However, after treatment with Dox, the DSCs displayed a spindle-like shape which was known as mesenchymal cell characteristic (Fig. 1a).
Transient Dox treatment induced EMT. a Dox (0.4 μg/ml) induced a dramatic morphologic change from cobblestone-like (left) to spindle-like (right) shape (the bars represent 100 μm). b Dox treatment increased vimentin expression while repressed E-cadherin expression. c Dox treatment induced Twist transcription (the bars represent 25 μm)
To further confirm that the DSCs had undergone EMT, we measured the expression of EMT markers in the DSCs. Comparing with control groups, the expression level of E-cadherin decreased, and that of vimentin increased in the DSCs, both in a dose-dependent manner (Fig. 1b–d). Furthermore, the mRNA level of mesenchymal transcription factor Twist enhanced significantly in the DSCs, whereas the abundance of Snail and Slug mRNA were not appreciably different between the DSCs and control groups (Fig. 1e). These observations showed that transient treatment with Dox induced EMT in BGC-823 cells.
Dox-induced EMT was associated with enhanced migration ability
The cytoskeleton remodeling during EMT confers cancer cell a slim morphology, which facilitates cell mobility. We performed Transwell assay to examine the migration abilities of BGC-823 cells treated with or without Dox. Figure 2a, b showed that 0.1 and 0.4 μg/ml Dox added in the upper chambers derived more cells to migrate through the micropore membranes into the lower chambers as compared with control groups. Wound-healing assay also showed that 0.4 μg/ml Dox in the culture medium accelerated the wound-healing process (Fig. 2c). These data demonstrated that Dox-induced EMT was associated with enhanced migration ability.
β-Catenin signaling was involved in Dox-induced EMT
One of the signals initiating EMT is the canonical Wnt/β-catenin pathway. To elucidate the mechanism underlying Dox-induced EMT, we measured the expression level of β-catenin in DSCs. As shown in Fig. 3a, Dox induced β-catenin expression in a dose-dependent manner. We also found that mRNA levels of Lef1 and c-myc, two pivotal transcription factors in β-catenin signaling, were both upregulated after Dox treatment (Fig. 3b). These results suggested that β-catenin signaling was involved in Dox induced EMT.
Indo inhibited β-catenin signaling in DSCs
Nonsteroidal anti-inflammatory drugs (NSAIDs) were reported to be selective inhibitors of β-catenin signaling [19, 20]. To investigate whether Indo, a member of the NSAIDs, could suppress Dox-induced β-catenin signaling, we treated BGC-823 cells with Dox in combination with Indo. To our surprise, β-catenin expression was elevated when 50 μM Indo was added into culture medium. However, when treated together with Dox, Indo significantly repressed β-catenin expression, and the repression was proportional to Dox concentrations (Fig. 4a). We proposed that other factor(s) induced by Dox was required for Indo to repress β-catenin expression.
Indo suppressed Dox-induced EMT
To investigate the effects of Indo on Dox-induced EMT, we treated BGC-823 cells with Dox and/or Indo. As shown in Fig. 4b–d, Dox-induced E-cadherin downregulation and vimentin and Twist upregulation were restored by Indo treatment. Meanwhile, 50 μM Indo also alleviated Dox induced in vitro migration (Fig. 5a, b). MTT assay showed that the reduced migration ability was not due to the combinational cytotoxic effects of Dox and Indo (Fig. 5c). Taken together, these data demonstrated that Indo suppressed Dox-induced EMT and in vitro cancer cell migration.
β-Catenin repression disrupted Dox-induced EMT
To further confirm the role of β-catenin in Dox-induced EMT, we used siRNA to inhibit the expression of β-catenin (Fig. 6a, b). After transfection of 50 nM β-catenin siRNA, the cells were cultured in medium containing 0.4 μg/ml Dox for 48 h. Knockdown of β-catenin restored the expression of E-cadherin and suppressed the expression of vimentin and Twist (Fig. 6c, d), which showed a similar effect as Indo on Dox-induced EMT. Moreover, Dox-induced cancer cell migration was also blocked by β-catenin siRNA (Fig. 6e). These data showed that β-catenin signaling played a critical role in Dox-induced EMT.
β-catenin repression disrupted Dox-induced EMT. a, b Dox-induced β-catenin expression (0.1 μg/ml) was repressed by siRNA. c, d β-catenin siRNA antagonized the effects of 0.1 μg/ml Dox on E-cadherin, vimentin, and Twist expression. e β-catenin siRNA blocked 0.1 μg/ml Dox-induced cancer cell migration. The bars represent 100 μm
Discussion
Chemotherapy is a systemic treatment involving the use of chemical agents to stop cancer cells from growing. Although chemotherapy effectively eliminates cancer cells, its opposite effects that enhance the malignancy of the treated cancers have also been reported [15, 21]. Recently, increasing evidence has showed that cancer cells exposed to chemotherapeutic drugs undergone EMT consistent with phenotypic changes, including multidrug resistance, and enhanced invasive abilities. However, the detailed mechanism(s) still remains unclear.
In the present study, we showed that β-catenin signaling was activated by transient Dox treatment (Fig. 3a, b), whereas inhibition of β-catenin by siRNA or Indo disrupted Dox-induced EMT and cancer cell migration (Figs. 4 and 6). These data demonstrated that β-catenin signaling played a critical role in transient Dox-induced EMT in BGC-823 cells. Ectopic expression of either Snail or Twist was sufficient to induced EMT in human mammary cells; however, their mechanisms are different [22, 23]. Indeed, we found that the mRNA level of Twist, but not that of Snail and Slug, was elevated in response to Dox treatment (Fig. 1e), indicating the unique role of Twist in Dox-induced EMT (Fig. 1c). Moreover, we found that inhibition of β-catenin expression decreased Twist transcription, suggesting that Twist was a target of β-catenin signaling (Figs. 4d and 6d).
Clinical trials confirmed the effectiveness of NSAIDs in cancer prevention, but the antineoplastic mechanisms are still in dispute [24–26]. Studies from colorectal cancer patients suggest that the anticancer effect of Indo and other NSAIDs is related to attenuating β-catenin signaling pathway [19, 20]. In contrast to these findings, we found that 50 μM Indo upregulated the expression of β-catenin, vimentin, and Twist and enhanced cancer cell migration ability (Figs. 4 and 5a), suggesting that Indo was capable of inducing EMT in BGC-823 cells. However, when Dox was added together with Indo into the culture, β-catenin expression was significantly repressed, and Dox-induced EMT was disrupt (Fig. 4). We proposed that other factor(s) induced by Dox was required for Indo to repress β-catenin expression. High level expression of peroxisome proliferator-activated receptor γ (PPAR-γ) and its co-receptor retinoid X receptor-α (RXR-α) was required for the interference with β-catenin function by NSAIDs [19]. Indeed, we recently found that PPAR-γ mRNA level was elevated 15-fold in response to 0.4 μg/ml Dox treatment, whereas 50 μM Indo had no such effect (data not shown). However, Hawcroft et al. demonstrated that the presence of functional PPAR-γ is not necessary for the antiproliferative activity associated with Indo in vitro [27]. Whether PPAR-γ or RXR-α was involved in the repression of β-catenin expression by Indo still needs further investigation.
In conclusion, our work showed that transient treatment with Dox initiated EMT and enhanced cancer cell in vitro migration ability. This process required the activation of β-catenin signaling. Repression of β-catenin by siRNA or Indo, a selective β-catenin inhibitor, effectively arrested Dox-induced EMT process. As few β-catenin inhibitors are available in clinical therapies, our findings hold implication for the use of NSAIDs to prevent the metastasis of gastric and other human cancers [28].
References
Chaffer CL, Weinberg RA. A perspective on cancer cell metastasis. Science. 2011;331:1559–64.
Hanahan D, Weinberg RA. The hallmarks of cancer. Cell. 2007;100:57–70.
Thiery JP. Epithelial-mesenchymal transitions in development and pathologies. Curr Opin Cell Biol. 2003;15:740–6.
Thiery JP, Acloque H, Huang RY, et al. Epithelial-mesenchymal transitions in development and disease. Cell. 2009;139:871–90.
Acloque H, Adams MS, Fishwick K, et al. Epithelial-mesenchymal transitions: the importance of changing cell state in development and disease. J Clin Invest. 2009;119:1438–49.
Pan JJ, Yang MH. The role of epithelial-mesenchymal transition in pancreatic cancer. J Gastrointest Oncol. 2011;2(3):151–6.
Xiao D, He J. Epithelial mesenchymal transition and lung cancer. J Thorac Dis. 2010;2(3):154–9.
Scheel C, Eaton EN, Li HJ. Paracrine and autocrine signals induce and maintain mesenchymal and stem cell states in the breast. Cell. 2011;145:926–40.
Kang Y, Massague J. Epithelial-mesenchymal transitions: Twist in development and metastasis. Cell. 2004;118:277–9.
Reya T, Clevers H. Wnt signalling in stem cells and cancer. Nature. 2005;434:843–50.
Clevers H. Wnt/β-catenin signaling in development and disease. Cell. 2006;127:469–80.
Willert K, Jones KA. Wnt signaling: is the party in the nucleus? Genes Dev. 2006;20:1394–404.
Li J, Zhou BP. Activation of β-catenin and Akt pathways by Twist are critical for the maintenance of EMT associated cancer stem cell-like characters. BMC Cancer. 2011;11:49–59.
Yang L, Lin C, Liu ZR. P68 RNA helicase mediates PDGF-induced epithelial mesenchymal transition by displacing Axin from beta-catenin. Cell. 2006;127:139–55.
Hanin L, Zaider M. Effects of surgery and chemotherapy on metastatic progression of prostate cancer: evidence from the natural history of the disease reconstructed through mathematical modeling. Cancers. 2011;3:3632–60.
Li QQ, Xu JD, Wang WJ, et al. Twist1-mediated adriamycin-induced epithelial-mesenchymal transition relates to multidrug resistance and invasive potential in breast cancer cells. Clin Cancer Res. 2009;15:2657–65.
Bandyopadhyay A, Wang L, Agyin J, et al. Doxorubicin in combination with a small TGFbeta inhibitor: a potential novel therapy for metastatic breast cancer in mouse models. PLoS One. 2010;5:e10365.
Huang Q, Gumireddy K, Schrier M, et al. The microRNAs miR-373 and miR-520c promote tumour invasion and metastasis. Nat Cell Biol. 2008;10:202–10.
Lu D, Cottam HB, Corr M, et al. Repression of β-catenin function in malignant cells by nonsteroidal anti-inflammatory drugs. PNAS. 2005;102:18567–71.
Hawcroft G, D'Amico M, Albanese C, et al. Indomethacin induces differential expression of β-catenin, γ-catenin and T-cell factor target genes in human colorectal cancer cells. Carcinogenesis. 2002;23:107–14.
Yamauchi K, Yang M, Hayashi K, et al. Induction of cancer metastasis by cyclophosphamide pretreatment of host mice: an opposite effect of chemotherapy. Cancer Res. 2008;68:516–20.
Mani SA, Guo W, Liao MJ, et al. The epithelial-mesenchymal transition generates cells with properties of stem cells. Cell. 2008;133:704–15.
Yang J, Weinberg RA. Epithelial-mesenchymal transition: at the crossroads of development and tumor metastasis. Dev Cell. 2008;14:818–29.
Huls G, Koornstra JJ, Kleibeuker JH. Non-steroidal anti-inflammatory drugs and molecular carcinogenesis of colorectal carcinomas. Lancet. 2003;362:230–2.
Sandler RS, Halabi S, Baron JA, et al. A randomized trial of aspirin to prevent colorectal adenomas in patients with previous colorectal cancer. N Engl J Med. 2003;348:883–90.
Baron JA, Cole BF, Sandler RS, et al. A randomized trial of aspirin to prevent colorectal adenomas. N Engl J Med. 2003;348:891–9.
Hawcroft G, Gardner SH, Hull MA. Activation of peroxisome proliferator-activated receptor does not explain the antiproliferative activity of the nonsteroidal anti-inflammatory drug indomethacin on human colorectal cancer cells. J Pharmacol Exp Ther. 2003;305:632–7.
Watanabe DX. Winning WNT: race to Wnt signaling inhibitors. PNAS. 2011;108:5929–30.
Acknowledgments
Rongfei Han and Jie Xiong equally contributed to this work. This study was supported by the National Natural Science Foundation of China (nos. 30971280, 30801336, and 81270607), Key Program of Natural Science Foundation of Hubei Province of China (no. 2011CDA044), and the Fundamental Research Fund for the Central Universities (no. 201130102020005).
Conflicts of interest
None.
Author information
Authors and Affiliations
Corresponding author
Rights and permissions
About this article
Cite this article
Han, R., Xiong, J., Xiao, R. et al. Activation of β-catenin signaling is critical for doxorubicin-induced epithelial–mesenchymal transition in BGC-823 gastric cancer cell line. Tumor Biol. 34, 277–284 (2013). https://doi.org/10.1007/s13277-012-0548-3
Received:
Accepted:
Published:
Issue Date:
DOI: https://doi.org/10.1007/s13277-012-0548-3