Abstract
Agave bagasse is a waste material generated during agave sap collection for “pulque” production. This residue is a potential feedstock for steroidal saponins and other phytochemicals. Ultrasonically assisted extraction (UAE) allows the use of green solvents such as water or ethanol for phytochemicals recovery. The influence of solvent composition and its effect on the intensity of the ultrasonic field during the UAE of agave bagasse saponins were evaluated. The best extraction conditions were a temperature of 60 °C (T) and a solvent-to-mass ratio of 20 (S/M), for conventional and UAE. In contrast to conventional extraction, where mixtures of ethanol-water were more efficient, water was the best extraction solvent in UAE. The amount of saponins obtained without ultrasound in 58% ethanol (22.48 ± 1.34 mg PE/g dw) was similar to the amount obtained by UAE in water (24.41 ± 0.84 mg PE/g dw). When only water was used, the ultrasound intensity was higher (271.40 ± 11.91 W/L) than in ethanol mixtures (144.81–202.30 W/L) due to cavitation intensity, demonstrated in a test on aluminum foil and SEM images of exhausted bagasse. Despite lower chemical affinity of water for saponins, its lower vapor pressure and viscosity, as well as higher surface tension, compared with ethanol, provided a greater cavitation intensity. Therefore, UAE using water is an excellent alternative to recover bioactive compounds from agave bagasse.
Similar content being viewed by others
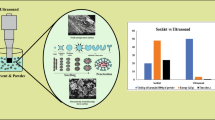
Explore related subjects
Discover the latest articles, news and stories from top researchers in related subjects.Avoid common mistakes on your manuscript.
Introduction
The extraction of bioactive compounds from plant tissues can be carried out via several methods, commonly involving the use of organic solvents. The most frequently used methods include solvent maceration and Soxhlet extraction, both requiring the use of organic solvents [2, 3]. However, these processes have the disadvantages of requiring a long extraction time, generating toxic emissions and organic wastes, a risk of flammability, and the possibility of leaving solvent residues in the extracts [2, 3].
Several technologies, such as supercritical fluid extraction (SFE), often using carbon dioxide in supercritical state (SC-CO2), have been proposed with the aim of avoiding or minimizing the use of organic solvents [2]. Unfortunately, SC-CO2 is not suitable for the dissolution and extraction of polar compounds, such as glycosides, that are tightly bound to the matrix [27]. Other alternatives include assisted extraction techniques, such as microwave-assisted extraction, enzyme-assisted extraction, and ultrasound assisted extraction (UAE).
During UAE, when ultrasound waves travel through the medium, the phenomenon of acoustic cavitation occurs due to the formation and collapse of bubbles in the liquid solvent [19]. The thermal and mechanical effects created by cavitation cause cell disruption, the breakdown of particles, and an improved mass transfer from the matrix to the solvent [30]. Among the advantages of UAE may be found enhanced mixing, ability to operate at lower temperatures, and the possibility of using green solvents, such as water or ethanol [19]. The efficiency of ultrasound in the extraction process depends on how the solvent’s physical properties, such as surface tension, vapor pressure, and viscosity, affect its capacity to absorb and transmit the vibrational energy into the medium; making solvent composition is decisive for this process [30]. UAE has been successful in enhancing the recovery of several bioproducts from vegetable tissues. When applied as a 10-min pretreatment at 400 W, the aqueous extraction of the betanin and isobetanin pigments from Opuntia stricta fruits increased from 22.8 to 50 mg/100 g [12]. UAE can also be carried out as a continuous ultrasonic irradiation process, enhancing the pectin extraction yield from Agave sisalana [17], the anthocyanin and phenolic recovery from mulberry pulp [7], antioxidants from mandarin and lime peels [32] or anthraquinones from the Morinda citrifolia root [9], among others.
Plant materials have been ancestrally considered as a valuable part of traditional medicine in diverse cultures, such as pre-Hispanic, Chinese, and Ayurvedic [1, 10]. Among these plants, the genus, Agave, is characterized by the presence of steroidal saponins with biological effects (antifungal, anti-inflammatory, anticarcinogenic, and others) [28, 31]. In addition, antioxidant compounds, including flavonoid glycosides, phenolic compounds, and homoisoflavonoids, have also been found in plants of this genus [16, 28]. In the Agave salmiana species, steroidal saponins with in vitro anticarcinogenic potential in colon and hepatic cancer cells have been identified in the fresh and concentrated sap [8, 26, 29], while compounds with antioxidant capacity, such as flavonoids and other phenolics, were reported in the leaf tissue [20,21,22]. Unfortunately, the conventional method used for the extraction of these saponins requires n-butanol or methanol [15, 20], which needs to be eliminated from the extract, generating toxic wastes and presenting the risk of leaving solvent residues in the final product.
Steroidal saponins from plants have been extracted by ultrasonically assisted methods, such as black garlic (Allium nigrum), the root Dioscorea panthaica, and the Asian medicinal plant, Paris polyphylla [5]. However, the main focus of these studies was the analysis and characterization of the extracted compounds. Up to date, there is no literature regarding the UAE of agave saponins. Moreover, the effect of solvent composition on the ultrasonic energy transmitted to the medium, the cavitation intensity, the damage to the tissue microstructure, and its effect on the extraction have not yet been reported. Therefore, the aim of this study was to determine the influence of solvent composition on obtaining extracts rich in saponins using ultrasound as affected by the ultrasonic intensity transmitted to the medium, evaluating different mixtures of water and ethanol.
Materials and Methods
Plant Material
Agave salmiana bagasse, a by-product of “aguamiel” (agave sap) production, was donated by the company AGMEL S.A. de C.V. (Monterrey, México). The bagasse sample was collected in the state of Coahuila, Mexico, in December 2016. It was sundried at an average temperature of 16 °C until its moisture content was 11%. The material was ground, sieved through a 1- to 3-mm mesh, and stored in darkness at room temperature until analysis.
Experimental Design and Statistical Analysis
Agave bagasse extraction was carried out according to a Box-Behnken experimental design (Table 1), where the effect of experimental factors, the temperature (T, °C), ethanol concentration (EC, %), and solvent-to-sample mass ratio (S/M), was evaluated on saponin concentration. The experiments were divided into two sets: extraction without ultrasound (NUS) and UAE. All the extractions were carried out using 10 g of agave bagasse, and the solvent-to-mass ratio was adjusted using the corresponding amount of solvent (100, 150, or 200 mL) according to the experimental design. The ethanol concentration was adjusted using 96% pharmaceutical degree ethanol (AppliChem GmbH, Darmstadt, Germany) and distilled water. One extraction replicate was carried out for each condition within the experimental design, and the center point was performed in triplicate.
Data from the Box-Behnken design was analyzed by ANOVA, and Pareto Chart to determine the significance of each factor and response surface methodology was used to observe the interactions graphically. The statistical model was obtained by the least squares fit methodology. Optimization was carried out by means of the desirability function, through the minimization of the covariance matrix of the model estimates. The data obtained from subsequent experiments to validate optimal conditions was analyzed by ANOVA. A significance level of α = 0.05 was applied in all analyses, and they were carried out using the software JMP 14 (2018).
Extraction Procedure and System Description
Extractions without ultrasound (NUS) were carried out in a borosilicate 600-mL jacketed extraction vessel (Cole-Parmer, Vernon Hills, IL, USA) using mechanical agitation (RZR 1, Heidolph Instruments GmbH & Co.KG, Schwabach, Germany) for 60 min. For UAE, a 400-W, 24-kHz ultrasonic processor (UP400S, Hielscher Ultrasonics GmbH, Teltow, Germany) was used. Amplitude was set at 70%, and the generator was coupled to a H22 sonotrode (Hielscher Ultrasonics GmbH, Teltow, Germany), 100 mm in length with a 22-mm-diameter tip. The sonotrode was submerged 10 mm into the solvent, and ultrasound was applied from 0 to 5 min, with subsequent mechanical agitation for 25 min. From 30 to 35 min, ultrasound was applied a second time and the extraction continued under mechanical agitation during a total extraction time of 60 min. The temperature within the extraction vessel was controlled by recirculating (Bunsen, Madrid, Spain) hot or cold water according to the desired set-point, using a PID controller (E5CK, Omron, Madrid, Spain) connected to a K-thermocouple sensor (T). The solvent temperature was adjusted to the desired set-point before adding 10 g of ground agave bagasse. At the end of the extraction, the samples were gravity filtered using 43—48 µm mesh paper filter (Filter-Lab 1300/80, Barcelona, Spain), the extract volume was measured, and the extract was concentrated to dryness under vacuum at 55 °C for saponin analysis.
Model Validation
After optimal conditions were obtained from the experimental design, validation was carried out in triplicate using the same extraction setup and experimental procedure. In the case of NUS, saponins were obtained at 60 °C, 58% ethanol, and S/M 20 under mechanical agitation for 60 min. For UAE, conditions were 60 °C, 0% ethanol (100% water), and S/M 20. During the 60 min extractions, ultrasound was applied for two periods of 5 min, as previously explained in “Extraction Procedure and System Description.”
Determination of Extraction Kinetics
Extraction kinetics experiments were performed in optimal conditions (“Model Validation”) to observe the effect of the extraction time on NUS and UAE. Extract aliquots (5 mL) were taken at 5, 10, 15, 20, 30, 45, 60, 90, and 120 min, and solvent was replenished. For UAE kinetics, ultrasound was applied for 5 min at the beginning of the extraction and for 5 more minutes after 30, 60, and 90 min. All the kinetics, NUS and UAE, were carried out for a total extraction time of 120 min. We carried out six independent kinetics experiments for each condition. On three replicates, sampling was done at 5, 15, 30, 60, and 120 min, and on the other three replicates, sampling was done at 10, 20, 45, and 90 min.
Kinetic data for each extraction was adjusted according to the Peleg equation (Eq. 1) [11]:
where c(t) is the concentration of saponins (mg PE/g dw), t is the extraction time (min), K1 is Peleg’s rate constant (min g dw/mg PE), and K2 is Peleg’s capacity constant (g dw/mg PE).
Peleg’s rate constant (K1) was used to determine the initial extraction rate (β0) following Eq. 2:
where β0 is the extraction rate at the beginning of the process (t = 0 min; mg PE/g dw min).
Peleg’s capacity constant (K2) was used to predict the amount of saponins (mg PE/g dw min) obtained at the plateau (cplateau) following Eq. 3:
Saponin Profile Characterization
The dry extracts from the experimental design (Table 1) and the model validation and kinetics experiments were re-suspended in 2 mL of HPLC grade aqueous methanol solution (50:50 v/v), centrifuged (5804r, Eppendorf AG, Hamburg, Germany) at 10,000×g to precipitate suspended matter and filtered through 0.25-µm PTFE membrane filters (Agilent Captiva Econofilter, Santa Clara, CA, USA). Saponins were identified by liquid chromatography coupled to a time of flight mass detector with electrospray source in positive mode (HPLC-MS-ESI-TOF, G1969A, Agilent Technologies, Santa Clara, CA, USA) [34]. The compounds were separated in a Zorbax Eclipse XDB-C18, 150 mm × 4.6 mm I.D, 5-μm column (Agilent Technologies, Santa Clara, CA, USA), using HPLC grade (A) water (Tedia, Fairfield, OH, USA) and (B) acetonitrile (Tedia, Fairfield, OH, USA) as mobile phase, both acidified with 0.1% (v/v) formic acid (CTR Scientific, Monterrey, Mexico).
The saponins were quantified by HPLC using an evaporative light scattering detector (HPLC-DAD-ELSD, Agilent Technologies, 1200 Series, Santa Clara, CA, USA) [34] and expressed as protodioscin equivalent milligram per gram of bagasse in dry weight (PE mg/g dw), using a protodioscin standard curve (Sigma-Aldrich, St. Louis, MO, USA) from 12.5 to 350 ppm. The reported values correspond to the total amount of the saponins found in each extract.
Characterization of the Acoustic Intensity in the Different Solvents
The acoustic energy supplied by the ultrasonic equipment to the solvents was assessed by means of the calorimetric method [25]. For each assay, 200 mL of the different solvents (0, 35, 70, and 96% ethanol) was placed in a thermally isolated vessel and heated to 60 °C. The sonotrode was submerged 10 mm into the solvent, and ultrasound was applied during 120 s. The water temperature increase was recorded using a K-type thermocouple placed in the vessel and connected to a data logger (HP Data Logger 34970 A, Hewlett-Packard Española, S. A., Madrid, Spain). The ultrasound power was calculated using Eq. 4:
where P represents the ultrasonic power (W), Cp is the heat capacity for each solvent obtained from literature (Table 2), and dT/dt is the increase in temperature over time. The volumetric power (W/L) was determined by dividing P by the volume of solvent used in the experiment (200 mL). Three replicates of each measurement were carried out.
To evaluate the cavitation, 200 mL of solvent was added to the extraction vessel and a piece of commercial aluminum foil was placed at the bottom of the vessel. The sonotrode was submerged into the liquid in the same way as the previous experiments (72.5 mm distance from the sonotrode tip to the aluminum foil), and ultrasound was applied for 1 min. Afterwards, the aluminum foil was collected. The foils were observed for cavitation damage (holes) through a stereoscope (SMZ1500, Nikon Instruments Inc., Melville, NY, USA) connected to a digital camera and pictures were taken. The procedure was carried out for 100% water and 96% ethanol.
Analysis of Bagasse Morphology After Extraction
To determine the physical effect of ultrasound on the extracted solid matrix, samples from agave bagasse were collected after the gravity filtration step and the remaining solvent was allowed to evaporate overnight at 25 °C. To observe contrasting solvent conditions for ultrasound cavitation, the central points (T = 45 °C, EC = 35% ethanol, S/M 15) and two axial points were chosen to analyze the solvent effect (T = 45 °C, EC = 0% ethanol, S/M 10 and T = 45 °C, EC = 70% ethanol, S/M 20), from both NUS and UAE experiments that were analyzed by scanning electron microscopy (SEM).
The samples were sputter coated with a layer of gold (5 nm) using a Quorum Q1505 rotary pump coater (Quorum Technologies Ltd, East Grinstead, UK). SEM was carried out using an EVO MA25 device (Zeiss, Oberkochen, Germany). The system was operated in high vacuum, with a 10-kV electron acceleration voltage and 8-mm work distance. Images were analyzed using the SmartSem 5.07 software (Zeiss, Oberkochen, Germany).
Results and Discussion
Effect of Process Parameters on Saponin Extraction
The total saponin content extracted from agave bagasse was between 3.53 and 20.51 mg PE/g dw, (Table 1) within the conditions of the experimental design. The multifactor ANOVA (Table 3), carried out on the experimental data, showed that the effect of all the factors (T, EC, S/F, and US) on total saponin extraction was significant (p < 0.05) and the lack of fit was not significant (p > 0.05).
Analyzing the effect of temperature (T), at 60 °C, 12.59 ± 0.73 mg PE/g dw of saponins were obtained, while at 30 °C, a significantly (p < 0.05) lower (30%) amount was achieved (8.71 ± 0.73 mg PE/g dw). No significant difference was observed regardless of whether 35 or 70% ethanol was used for saponin extraction, recovering 11.25 ± 0.54 or 12.15 ± 0.73 mg PE/g dw, respectively. By contrast, when using water, only 8.76 ± 0.72 mg PE/g dw were obtained, significantly (p < 0.05) lower when using 35 or 70% ethanol. With respect to solvent-to-mass ratio (S/M), ratios of 15 and 20 produced a similar result on saponin recovery, obtaining 11.13 ± 0.54 and 13.31 ± 0.72 mg PE/g dw, respectively. These values were significantly (p < 0.05) higher than for a ratio of 10, where only 7.71 ± 0.72 mg PE/g dw were obtained. Finally, when using ultrasound, a significantly (p < 0.05) higher amount of saponins was extracted (12.21 ± 0.58 mg PE/g dw) than when no ultrasound was applied (9.23 ± 0.58 mg PE/g dw of saponins).
Overall, the use of higher temperatures, ethanol concentrations and solvent-to-mass ratios, and the application of ultrasound led to an increase in saponin extraction from agave bagasse. The increase in saponin extraction and AOXC when using ultrasound could be due to acoustic cavitation since it accelerates solvent penetration into the tissue, generates tissue disruption, and enhances the mass transfer rate due to microjets and turbulence [33].
Optimization by Response Surface Methodology
To thoroughly analyze the effect of the process parameters on total saponin content, a response surface analysis was carried out in two sets of experiments: without ultrasound and using ultrasound-assisted extraction (UAE). When the block without ultrasound was analyzed, a model for saponin extraction was fitted considering the three principal factors (T, EC and S/M) and the quadratic effects of EC and S/M, as well as interaction (T × S/M). The remaining quadratic effects and factor interactions were not significant (p > 0.6) and therefore excluded from the model. The model was significant (p < 0.05; Fig. 1a), with a determination coefficient of 0.74. The parameter of ethanol concentration (EC) was the most important driving force behind the extraction, along with the S/M. Chemically, saponins consist of a steroid skeleton joined to a sugar moiety, which can be formed from one sugar unit to chains of six sugar units, making them soluble in alcohol/water mixtures [31]. The solvent-to-mass ratio is also determinant in a solid-liquid extraction, since the greater the S/F, the higher the concentration gradient between the solvent and the plant material, driving the mass transfer during extraction [5, 6]. These two factors, however, explained only 52.6% of the variance in the experimental data. Although the temperature effect was not significant (p = 0.08), when considered along with EC and S/M, 71.2% of the variance was explained by the model. Regarding the phenomena observed during extraction, higher temperatures generally result in a better diffusivity and, therefore, an improved extraction yield [4]. In every case, the parameters had a positive effect on extraction. The best experimental results were obtained in combinations involving the highest ethanol concentrations, solvent-to-mass ratios, and temperatures (Table 1; experiments 11, 14 and 15), extracting more than 12 mg PE/g dw.
Carrying out the model optimization using the desirability function, the conditions for the maximum saponin extraction without ultrasound were determined to be T = 60 °C, EC = 58% ethanol, and S/M = 20, with a desirability of 0.91. The model predicted a saponin concentration 95% confidence interval between 11.04 and 18.85 mg PE/g dw; experimentally, 22.48 ± 1.34 mg PE/g dw were obtained. Using the model equation to make estimations at 60 °C and a solvent-to-mass ratio of 20 but without ethanol in the solvent mixture, saponin recovery decreases to 10.56 mg PE/g dw.
For the UAE set of experiments, the fitted model did not consider the quadratic effects, since statistical significance was very low (p > 0.6) but included the effect of the three main factors (T, EC, and S/M) as well as the interactions between them. The model was significant (p < 0.05) and had a determination coefficient of 0.90. In this case, all three of the principal factors were considered significant (p < 0.05; Fig. 1b) and were able to explain 81.0% of the variance observed within the experimental data. Each of them had a positive effect on saponin extraction. The higher the S/M, T, and EC were, the larger the amount of extracted saponins. Within the experimental conditions evaluated in the design, the best results were obtained at T = 60 °C, EC = 35% ethanol, S/M = 20 (Table 1; exp 14), reaching 20.51 mg PE/g dw. None of the other evaluated conditions permitted a similar saponin recovery.
When optimization was carried out by means of the desirability function, the optimal conditions were T = 60 °C, EC = 0% ethanol, S/M = 20. The desirability was 0.90, and the predicted 95% confidence interval ranged from 15.50 to 23.52 mg PE/g dw. In this case, the experimental results were slightly higher, obtaining 24.41 ± 0.84 mg PE/G dw (Table 1). It is interesting to note that while the Pareto chart (Fig. 1b) indicated that a higher ethanol concentration yields a higher amount of saponins, the optimal level for EC was 0%. However, it has been documented that the best solvent for traditional extraction may not be optimal for UAE, due to the effectiveness of cavitation [6].
When optimization was carried out for the extraction of AOXC using ultrasound, the best conditions were T = 60 °C, EC = 0% ethanol, and S/M = 20. With these parameters, a 0.99 desirability was obtained, and the model predicted a 95% confidence interval for AOXC between 94.99 and 103.37 μmol TE/g. In the validation experiments, 96.79 μmol TE/g were obtained, confirming the validity of the model prediction. It was interesting to see that, in the case of UAE, the same optimal conditions were obtained for both AOXC and saponins.
When comparing these results to a two-step extraction using organic solvents, only 1.36 mg PE/g dw of total saponins was recovered [18]. Contrastingly, in this study, 24.41 ± 0.84 mg PE/g dw of saponins were extracted under optimized UAE. The method used by Menache-Carretero [18] involved the use of 80% methanol as an extraction solvent, which co-extracted a considerable amount of fiber, requiring a subsequent partition with a two-phase system composed of n-butanol, ethyl acetate, and water to obtain a saponin-rich extract. Alternatively, the extraction with supercritical CO2 and 70% ethanol as a co-solvent did not show very efficient results, only permitting the recovery of 0.07 mg PE/g dw when the system was enhanced with ultrasound at 450 bar, 60 °C, and using 10% of co-solvent [27]. In the present study, a 300 times higher saponin extraction was observed for UAE at 60 °C, using water at a ratio of 20, applying a less expensive technology, compared with supercritical fluid extraction.
Regarding other agave tissues, more saponins were recovered in this study by optimizing extraction conditions for both NUS and UAE. The maximum amount of saponins obtained from fresh or concentrated Agave salmiana sap using 50% aqueous n-butanol at 25 °C, a solvent-to-mass ratio of 20 (50 mL for 2.5 g) after 60 min of extraction was 50 lower than in this study (0.35–0.48 mg PE/g dw) [15, 26, 29]. In the case of the leaf tissue, the amount of saponins extracted using 80% methanol with S/F 10 was 2.8 times lower than in this work (2.1–8.5 mg PE/g dw) [20,21,22].
Saponin Extraction Kinetics
To determine the effect of the extraction time and assess a suitable time for the process, saponin kinetics were determined for NUS and UAE, carrying out the experiments in the optimal conditions for each process (Fig. 2). NUS extraction kinetics were evaluated at 60 °C, 58% ethanol, and S/F 20, and it was observed that 60 min are required to reach the plateau. By this time, 90% of the total amount of saponins was recovered (22.48 mg PE/g dw). More than 90 min were required to recover 92% of the maximum amount of saponins (23.06 mg PE/g dw).
The Peleg model kinetic parameters were adjusted for NUS (Table 4). Peleg’s rate constant (K1) for saponins was 0.2790 min × (g dw/mg PE), and Peleg’s capacity constant (K2) was 0.04303 g dw/mg PE. The model had a correlation coefficient of 0.959, with root mean square error (RMSE) of 1.186, indicating a good fit of the model to the experimental data. With the values of K2, the plateau amount of saponins under these conditions can be estimated at 24.81 mg PE/g dw of agave bagasse, which is close to the experimental value observed of 25.10 mg PE/g dw.
UAE kinetics were evaluated at 60 °C, 0% ethanol, and S/F 20. In this case, the plateau for saponins was reached after 60 min (Fig. 2; 24.41 mg PE/g dw of agave bagasse), observing an 85% recovery after 45 min of extraction (21.41 mg PE/g dw). Further extraction time than required to reach the plateau did not yield a significant increase in saponins. Therefore, under optimal conditions, 45 min could be an adequate time to obtain a saponin rich extract.
For UAE, the Peleg’s model kinetic parameters (Table 4) were a K1 of 0.2012 min*(g dw/mg PE) and K2 of 0.0407 g dw/mg PE. The model had a correlation coefficient of 0.8808 and a root mean square error (RMSE) of 1.879, indicating that the model was not as accurate as the one for NUS. With the values of K2, the plateau amount of saponins extracted under these conditions can be estimated at 24.57 mg PE/g dw of agave bagasse, close to the experimental values observed of 25.06 mg PE/g dw.
The Peleg’s rate constant (Table 4) indicates that the initial extraction rates for UAE (4.97 mg PE/g dw) were faster than for NUS (3.58 mg PE/g dw min). Therefore, both the experimental data and the model show that the process can be carried out faster with the optimized UAE, requiring less than 60 min to recover 90% of the saponins without the use of ethanol.
Saponin Characterization
Regarding extract composition, seven different steroidal saponins were identified in agave bagasse extracts, including four kammogenin glycosides and one manogenin glycoside (Fig. 3), characteristic of Agave salmiana sap and bagasse [26, 27, 29]. Compound 1 was identified as a kammogenin hexaglycoside with four hexose and 2 pentose residues in the sugar moiety (Fig. 3b) and was the second most abundant compound in agave bagasse extracts (Table 5). The pseudomolecular ion was m/z 1357.57 and corresponded to M + H+. The fragments for the pentaglycoside (m/z 1225.53), tetraglycosides (m/z 1093.49 and m/z 1063.49), triglycoside (m/z 931.44), diglycoside (m/z 769.41), monoglycoside (m/z 607.35), and the hydrated aglycone (m/z 467.27) were also observed in the mass spectrum of this compound. This compound has been previously identified in Agave salmiana bagasse [27].
Compound 2 was a kammogenin pentaglycoside with 3 hexose and 2 pentose residues in the glycosidic moiety (Fig. 3b), and the most abundant saponin was quantitated in this study (Table 5). The pseudomolecular ion m/z was shown as 1195.52 in the [M + H]+ form. Fragments of the tetraglycosides (m/z 1063.49 and 1033.49), triglycosides (m/z 931.44 and 901.44), diglycoside (m/z 769.41), monoglycoside (m/z 607.35), and the aglycone kammogenin (m/z 445.29) were observed. According to its molecular mass, this compound appears to be an isomer for magueyoside A, which was previously identified in A. salmiana sap [15, 26, 29]. However, the fragmentation pattern showing the tetraglycosides indicates that the sugars are likely arranged in a different order.
Compound 3 was identified as an hecogenin hexaglycoside, with four hexose and two pentose residues in the sugar moiety and a pseudomolecular ion m/z 1343.63 [M + H]+, but was under the quantitation limit (< 0.3 mg PE/g dw agave bagasse) The fragments for the pentaglycoside (m/z 1211.56), diglycoside (m/z 755.42), and monoglycoside (m/z 593.37) were observed within the mass spectrum, but not the aglycone. Though this particular compound, as far as we know, has not been reported in agave, the fragments that are observed are analogous to the ones from compound 1, and the monoglycoside ion is characteristic for hecogenin [15].
Compounds 4 and 5 coelute in one peak (Fig. 3b) and were identified as magueyoside A and magueyoside B (Fig. 3b), and the sum of both represented the lowest abundance in agave bagasse (Table 5). These two compounds have been among the most representative saponins identified in Agave salmiana sap and bagasse [8, 15, 26, 27, 29]. Magueyoside B, in particular, has presented potential antiproliferative activity on colon and hepatic cancer in vitro; therefore, there is interest in the recovery of this particular saponin [8, 26, 26].
Compounds 6 and 7 also coelute as a single peak and were previously identified in Agave salmiana, corresponding to the third place in abundance, with respect to other saponins (Table 5). Compounds 6 corresponds to a kammogenin tetraglycoside with two hexose and two pentose residues, characteristic in A. salmiana sap and bagasse, with the pseudomolecular m/z 1055.46 [M + Na]+. Compound 7 was identified as magueyoside C, a manogenin tetraglycoside with 3 hexose and two pentose residues, analogous to magueyoside B and also previously reported in agave sap and bagasse [15, 26, 27, 29].
Characterization of the Acoustic Intensity in the Different Solvents
When no ultrasound was applied, the best solvent for the extraction saponins was a mixture of ethanol in water (58%) due to the chemical affinity of the extracted molecules and the solvent. In the case of UAE, the best solvent was pure water since the ultrasonic intensity conveyed to the medium; therefore, its intensification effect was linked to the solvent composition. Hemiwol et al. (2006) reported how properties of the liquid, such as vapor pressure, viscosity, and surface tension, can have an influence on the efficiency of ultrasound on UAE [9], though the actual effect on the acoustic field was not measured by these authors.
Two tests were carried out to determine whether the ultrasound generated a stronger acoustic field on water than on ethanol mixtures. The calorimetric test (Fig. 4a) indicated that, at 70% amplitude, a power of 271.40 ± 11.91 W/L was obtained when pure water was used (0% ethanol), the highest observed in this test. As the ethanol concentration increased, there was a reduction in the power delivered into the medium, following a quadratic trend (RSq = 0.9831). The minimum power (144.81 ± 10.43 W/L) occurred when using a 96% aqueous solution, representing 53% of the power delivered when only water was used. With respect to the specific ethanol concentrations used in the extraction experiments, it was observed that with 35% ethanol, the power was reduced to 74% with respect to when using water, to 69% when using 58% ethanol, and to 63% with 70% ethanol (Fig. 4a). Moreover, the cavitation experiment demonstrated that when using 100% water, the microbubble explosion was strong enough to generate holes in the aluminum foil placed at the bottom of the water vessel subjected to ultrasound (Fig. 4b). By contrast, when carrying out the same experiment using 96% ethanol, cavitation did cause deformation of the aluminum foil, but it was not strong enough to break it (Fig. 4c).
The intensity of cavitation in UAE depends on the solvent chosen for the process, since it is affected by parameters, such as vapor pressure, surface tension, and viscosity [19]. In this regard, a lower vapor pressure and viscosity enhance cavitation [9]. When the vapor pressure is higher, more cavitation bubbles are formed in the liquid; however, the bubbles implode with greater force when the vapor pressure is lower, generating a greater cavitation effect [9, 14, 33]. With respect to viscosity, when it is high, more ultrasound intensity is required to overcome the cohesive forces between the liquid molecules and is lost as friction [9]. In the case of surface tension, when a liquid has a low surface tension, more cavitation bubbles are formed [9]. However, when the liquid has a higher surface tension, the force of the bubble collapse is stronger and creates a stronger cavitation effect. The addition of ethanol to the extraction solvent generates an increase in viscosity and vapor pressure, as well as a decrease in surface tension (Table 2), thereby mitigating the cavitation effect. This is observed in a decrease in the amount of power delivered, measured by means of the calorimetric method (Fig. 4a), as well as in a milder effect of the cavitation bubbles on the aluminum foil (Fig. 4 b and c). Accordingly, it can be concluded that, in spite of the fact that water has a lower chemical affinity for saponins and other phytochemicals than ethanol, its physical properties (viscosity, vapor pressure and surface tension) provide better conditions for cavitation and, thus, ultrasound intensification. This factor exerted more influence than the solvent polarity.
Effect of Ultrasound on Tissue Microstructure
The effect of disruptive mechanical damage was observed by using scanning electron microscopy to analyze the tissue after extraction (SEM; Fig. 5). When only water was used (0% ethanol), SEM images show that the physical structure of the bagasse tissue was scarcely damaged when no ultrasound was used (Fig. 5a). By contrast, after UAE using 0% ethanol, the agave tissue exhibited significant structural damage, observed as sections of broken tissue in the SEM image (Fig. 5b). Ultrasound was reported to generate damage to vegetable tissue by means of fragmentation and erosion, caused not only by cavitation but also by particle collision due to shockwaves [4]. Cavitation can create microjets of fluid that generate the erosion and destruction of the cell walls upon contact with the solid tissue, enhancing the extraction [14].
The influence of ethanol on the bagasse tissue was also observed by SEM. Increasing ethanol concentration to 35% (Fig. 5c) and 70% (Fig. 5e) generated damage to bagasse structure. Studies into the use of ethanol in the dehydration and rehydration of vegetable tissue have determined that ethanol increases the permeability of the cell walls, improving the mass transfer between the internal cell content and the medium [9, 24]. This effect appears more evident when using 70% ethanol (Fig. 5e) than when only 35% ethanol is used (Fig. 5c). However, when comparing the effect ultrasound has on the agave tissue during UAE using aqueous ethanol (Fig. 5d, f), it was observed that the disruptive effect was not as severe as when using only water as the extraction solvent. These results correlate well with the evaluation of both the volumetric power (Fig. 4a) and the differences observed in cavitation (Fig. 4b, c), which point to the fact that water is a better solvent for the UAE of saponins from agave bagasse.
SEM images of agave bagasse subjected to extraction at a 45 °C, 0% ethanol, solvent-to-mass ratio of 15; b UAE at 45 °C, 0% ethanol, solvent-to-mass ratio of 15; c 45 °C, 35% ethanol, solvent-to-mass ratio of 15; d UAE at 45 °C, 35% ethanol, solvent-to-mass ratio of 15; e 45 °C, 70% ethanol, solvent-to-mass ratio of 20; and f UAE at 45 °C, 70% ethanol, solvent-to-mass ratio of 20
Conclusions
In this study, saponins from Agave salmiana bagasse were successfully extracted with and without ultrasound at 60 °C and a solvent-to-mass ratio of 20. For extractions without ultrasound, the best solvent choice was a mixture of ethanol and water. However, when ultrasound was applied for extraction, saponins from agave bagasse were better extracted using only water as a solvent. This phenomenon was due to the more intense power density and cavitation in water than in ethanol mixtures due to its lower vapor pressure and viscosity and to its higher surface tension. The more intense cavitation in water leads to better tissue disintegration and mass transfer coefficients. Therefore, the feasibility of using water for the UAE of agave phytochemicals represents an interesting possibility for industrial applications, reducing the use of organic solvents and providing a greener alternative for the exploitation of agro-industrial waste. Furthermore, aqueous extracts can be further processed by technologies, such as spray drying or lyophilization, or incorporated as a liquid ingredient and used in a food product, without requiring solvent-removal steps.
References
Alonso-Castro AJ (2014) Use of medicinal fauna in Mexican traditional medicine. J Ethnopharmacol 152(1):53–70. https://doi.org/10.1016/j.jep.2014.01.005
Azmir J, Zaidul ISM, Rahman MM, Sharif KM, Mohamed A, Sahena F et al (2013) Techniques for extraction of bioactive compounds from plant materials: A review. J Food Eng 117(4):426–436. https://doi.org/10.1016/j.jfoodeng.2013.01.014
Azwanida N (2015) A review on the extraction methods use in medicinal plants, principle, strength and limitation. Medicinal & Aromatic Plants 04(03):3–8. https://doi.org/10.4172/2167-0412.1000196
Chemat F, Rombaut N, Sicaire AG, Meullemiestre A, Fabiano-Tixier AS, Abert-Vian M (2017) Ultrasound assisted extraction of food and natural products. Mechanisms, techniques, combinations, protocols and applications. A review Ultrasonics Sonochemistry 34:540–560. https://doi.org/10.1016/j.ultsonch.2016.06.035
Cheok CY, Salman HAK, Sulaiman R (2014) Extraction and quantification of saponins: A review. Food Res Int 59:16–40. https://doi.org/10.1016/j.foodres.2014.01.057
Esclapez MD, García-Pérez JV, Mulet A, Cárcel JA (2011) Ultrasound-assisted extraction of natural products. Food Engineering Reviews 3(2):108–120. https://doi.org/10.1007/s12393-011-9036-6
Espada-Bellido E, Ferreiro-González M, Carrera C, Palma M, Barroso CG, Barbero GF (2017) Optimization of the ultrasound-assisted extraction of anthocyanins and total phenolic compounds in mulberry (Morus nigra) pulp. Food Chem 219:23–32. https://doi.org/10.1016/j.foodchem.2016.09.122
Figueroa LM, Santos-Zea L, Escalante A, Gutiérrez-Uribe JA (2017) Mass spectrometry-based metabolomics of agave sap (Agave salmiana) after its inoculation with microorganisms isolated from agave sap concentrate selected to enhance anticancer activity. Sustainability, 9(11):2095. https://doi.org/10.3390/su9112095
Hemwimol S, Pavasant P, Shotipruk A (2006) Ultrasound-assisted extraction of anthraquinones from roots of Morinda citrifolia. Ultrason Sonochem 13(6):543–548. https://doi.org/10.1016/j.ultsonch.2005.09.009
Hosseinzadeh S, Jafarikukuhdan A, Hosseini A, Armand R (2015) The application of medicinal plants in traditional and modern medicine: A review of Thymus vulgaris. International Journal of Clinical Medicine 6:635–642. https://doi.org/10.1007/978-981-10-1926-5_79
Jokić, S., Velić, D., Bilić, M., Bucić-Kojić, A., Planinić, M., & Tomas, S. (2010). Modelling of solid-liquid extraction process of total polyphenols from soybeans. Czech Journal of Food Sciences, 28(3):206–212. https://doi.org/10.17221/200/2009-cjfs
Koubaa M, Barba FJ, Grimi N, Mhemdi H, Koubaa W, Boussetta N, Vorobiev E (2016) Recovery of colorants from red prickly pear peels and pulps enhanced by pulsed electric field and ultrasound. Innovative Food Science and Emerging Technologies 37:336–344. https://doi.org/10.1016/j.ifset.2016.04.015
Lapornik B, Prošek M, Wondra AG (2005) Comparison of extracts prepared from plant by-products using different solvents and extraction time. J Food Eng 71(2):214–222. https://doi.org/10.1016/j.jfoodeng.2004.10.036
Lavilla I, Bendicho C (2017) Fundamentals of ultrasound-assisted extraction. From Plants to Drug Development. Elsevier Inc., Water Extraction of Bioactive Compounds. https://doi.org/10.1016/B978-0-12-809380-1.00011-5
Leal-Díaz AM, Santos-Zea L, Martínez-Escobedo HC, Guajardo-Flores D, Gutiérrez-Uribe JA, Serna-Saldivar SO (2015) Effect of Agave americana and Agave salmiana ripeness on saponin content from aguamiel (agave sap). Journal of Agricultural and Food Chemistry 63(15):3924–3930. https://doi.org/10.1021/acs.jafc.5b00883
López-Romero JC, Ayala-Zavala JF, Peña-Ramos EA, Hernández J, González-Ríos H (2018) Antioxidant and antimicrobial activity of Agave angustifolia extract on overall quality and shelf life of pork patties stored under refrigeration. J Food Sci Technol 55(11):4413–4423
Maran JP, Priya B (2015) Ultrasound-assisted extraction of pectin from sisal waste. Carbohyd Polym 115:732–738. https://doi.org/10.1016/j.carbpol.2014.07.058
Menache-Carretero E (2018) Aislamiento e identificación de saponinas esteroidales de bagazo de agave con potencial apoptótico. Tecnológico de Monterrey, Monterrey, Mexico (Unpublished undergraduate thesis)
Panda D, Manickam S (2019) Cavitation technology—The future of greener extraction method: a review on the extraction of natural products and process intensification mechanism and perspectives. Applied Sciences 9(4):766. https://doi.org/10.3390/app9040766
Puente-Garza CA, Espinosa-Leal CA, García-Lara S (2018) Steroidal saponin and flavonol content and antioxidant activity during sporophyte development of maguey (Agave salmiana). Plant Foods Hum Nutr. https://doi.org/10.1007/s11130-018-0684-z
Puente-Garza CA, García-Lara S, Gutiérrez-Uribe JA (2017) Enhancement of saponins and flavonols by micropropagation of Agave salmiana. Ind Crops Prod 105(May):225–230. https://doi.org/10.1016/j.indcrop.2017.05.014
Puente-Garza CA, Meza-Miranda C, Ochoa-Martínez D, García-Lara S (2017) Effect of in vitro drought stress on phenolic acids, flavonols, saponins, and antioxidant activity in Agave salmiana. Plant Physiol Biochem 115:400–407. https://doi.org/10.1016/j.plaphy.2017.04.012
Pulido R, Bravo L, Saura-Calixto F (2000) Antioxidant activity of dietary polyphenols as determined by a modified ferric reducing/antioxidant power assay. Journal of Agricultural and Food Chemistry 48(8):3396–3402. https://doi.org/10.1021/jf9913458
Rojas ML, Augusto PED (2018) Ethanol pre-treatment improves vegetable drying and rehydration: Kinetics, mechanisms and impact on viscoelastic properties. J Food Eng 233:17–27. https://doi.org/10.1016/j.jfoodeng.2018.03.028
Santos-Zea L, Antunes-Ricardo M, Gutierrez-Uribe JA, García-Pérez JV, Benedito J (2018) Effect of ultrasound transducer design on the acoustically-assisted supercritical fluid extraction of antioxidants from oregano. Ultrason Sonochem 47:47–56. https://doi.org/10.1016/j.ultsonch.2018.04.019
Santos-Zea L, Fajardo-Ramírez OR, Romo-López I, Gutiérrez-Uribe JA (2016) Fast centrifugal partition chromatography fractionation of concentrated agave (Agave salmiana) sap to obtain saponins with apoptotic effect on colon cancer cells. Plant Foods Hum Nutr 71(1):57–63. https://doi.org/10.1007/s11130-015-0525-2
Santos-Zea, L., Gutiérrez-Uribe, J. A., & Benedito, J. (2019). Effect of ultrasound intensification on the supercritical fluid extraction of phytochemicals from Agave salmiana bagasse. Journal of Supercritical Fluids, 144:98-107. https://doi.org/10.1016/j.supflu.2018.10.013
Santos-Zea, L., Leal-Diaz, A., Cortes-Ceballos, E., & Gutierrez- Uribe, J. (2012). Agave (Agave spp.) and its traditional products as a source of bioactive compounds. Current Bioactive Compounds, 8(3):218–231. https://doi.org/10.2174/157340712802762410
Santos-Zea L, Rosas-Pérez AM, Leal-Díaz AM, Gutiérrez-Uribe JA (2016) Variability in saponin content, cancer antiproliferative activity and physicochemical properties of concentrated agave sap. J Food Sci 81(8):H2069–H2075. https://doi.org/10.1111/1750-3841.13376
Shirsath SR, Sonawane SH, Gogate PR (2012) Intensification of extraction of natural products using ultrasonic irradiations-A review of current status. Chem Eng Process 53:10–23. https://doi.org/10.1016/j.cep.2012.01.003
Sidana J, Singh B, Sharma OP (2016) Saponins of Agave: chemistry and bioactivity. Phytochemistry 130:22–46. https://doi.org/10.1016/j.phytochem.2016.06.010
Singanusong, R., Nipornram, S., Tochampa, W., Rattanatraiwong, P. (2015). Low power ultrasound-assisted extraction of phenolic compounds from mandarin (Citrus reticulata Blanco cv. Sainampueng) and lime (Citrus aurantifolia) peels and the antioxidant. Food Analytical Methods, 8(5):1112–1123. https://doi.org/10.1007/s12161-014-9992-6
Tiwari BK (2015) Ultrasound: A clean, green extraction technology. TrAC - Trends in Analytical Chemistry 71:100–109. https://doi.org/10.1016/j.trac.2015.04.013
Urbina CJF, Casas A, Martínez-Díaz Y, Santos-Zea L, Gutiérrez-Uribe JA (2018) Domestication and saponins contents in a gradient of management intensity of agaves: Agave cupreata, A. inaequidens and A. hookeri in central Mexico. Genetic Res Crop Evol 65(4):1133–1146. https://doi.org/10.1007/s10722-017-0601-6
Vazquez G, Alvarez E, Navaza JM (1995) Surface ttension of alcohol + water from 20 to 50 °C. J Cheml Engr Data 40:611–614. https://doi.org/10.1021/je00019a016
Acknowledgments
We thank the ASPA group technicians, Nieves Pérez-Muelas Picón and Ramón Peña, for the technical support, as well as Albino Vargas from AGMEL S.A de C.V for donating agave bagasse for this project.
Funding
The authors received financial support from CONACYT CVU 270166 through the program “Apoyo para Estancias Posdoctorales en el Extranjero Vinculadas a la Consolidación de Grupos de Investigación y Fortalecimiento del Posgrado Nacional,” Mexico; NutriOmics Chair from Tecnológico de Monterrey, Mexico; and Nutrigenomics Research Chair from Fundación FEMSA, Mexico.
Author information
Authors and Affiliations
Corresponding author
Ethics declarations
Conflict of Interest
The authors declare that there is no conflict of interest.
Additional information
Publisher’s Note
Springer Nature remains neutral with regard to jurisdictional claims in published maps and institutional affiliations.
Rights and permissions
About this article
Cite this article
Santos-Zea, L., Gutierrez-Uribe, J.A. & Benedito, J. Effect of Solvent Composition on Ultrasound-Generated Intensity and Its Influence on the Ultrasonically Assisted Extraction of Bioactives from Agave Bagasse (Agave salmiana). Food Eng Rev 13, 713–725 (2021). https://doi.org/10.1007/s12393-020-09260-x
Received:
Accepted:
Published:
Issue Date:
DOI: https://doi.org/10.1007/s12393-020-09260-x