Abstract
Two polyubiquitin genes, bul409 and bul427, were isolated from a Solanum bulbocastanum BAC library. The bul409 and bul427 genes encode hexameric and heptameric polyproteins, respectively. bul427 exhibits a number of features suggestive of a pseudogene: (1) The last ubiquitin monomer of bul427 is interrupted by a frame shift mutation. (2) The coding sequence is flanked 3′ by mitochondrial and chloroplast sequences and 5′ by a protein kinase pseudogene. However, characterization of cDNAs amplified using bul427-based primers demonstrated that bul427 is transcriptionally active. Chimeric transgenes encoding β-glucuronidase (GUS) translationally fused to the first ubiquitin-coding units of bul409, a truncated version 409s, and bul427 were constructed and introduced into potato. In transgenic potato lines both S. bulbocastanum promoters were weakly transcribed in tubers and efficiently transcribed in leaves. In leaves, bul409s was wound-induced, while bul427 was not. In tubers both promoters were wound-induced. In unwounded leaves and tubers, the steady state mRNA levels from both bul promoters were lower than the steady state mRNA levels from the Cauliflower Mosaic Virus 35S promoter. However, in the leaves and tubers of many of the transgenic lines the GUS activity was significantly higher in the bul lines compared to the 35S lines. The apparent inconsistency of higher enzymatic activity correlated with lower steady state levels of mRNA demonstrates the enhanced protein expression observed with ubiquitin fusion proteins.
Resumen
Dos genes de poliubiquitina, bul409 y bul427, fueron aislados de una librería BAC de Solanum bulbocastanum. Los genes bul409 y bul427 codifican poliproteínas hexámera y heptámera, respectivamente. El bul427 exhibe varios rasgos que sugieren un seudo gen: (1) El último monómero de bul427 es interrumpido por una mutación con desplazamiento de la pauta de lectura. (2) La secuencia codificante es flanqueada en 3′ por secuencias mitocondriales y de cloroplastos y en 5′ por un seudogen de proteína quinasa. Sin embargo, la caracterización de cDNAs amplificadas usando iniciadores basados en bul427 demostró que bul427 es transcripcionalmente activo. Se construyeron e introdujeron en papa transgenes quiméricos que codifican β-glucuronidasa (GUS) translacionalmente fusionados a las primeras unidades que codifican ubiquitina de bul409, una versión truncada de 409s y bul427. En las líneas de papa transgénica de S. bulbocastanum, los promotores fueron débilmente transcritos en los tubérculos y eficientemente transcritos en hojas. En hojas el bul409s fue inducido por heridas, mientras que bul427 no fue. En tubérculos, ambos promotores fueron inducidos por heridas. En hojas y tubérculos sin herir, los niveles mRNA en estado de equilibrio para ambos promotores bul fueron más bajos que los niveles de mRNA en estado de equilibrio del promotor 35S del virus del Mosaico de la Coliflor. Sin embargo, en hojas y tubérculos de muchas líneas transgénicas, la actividad de GUS fue significativamente más alta en las líneas bul en comparación con las 35S. La aparente inconsistencia de una actividad enzimática mayor, correlacionada con bajos niveles de mRNA en estado de equilibrio demuestra el incremento de la expresión de proteína observada con proteínas fusión ubiquitina.
Similar content being viewed by others
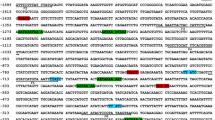
Avoid common mistakes on your manuscript.
Introduction
Ubiquitin is a small (76 amino acids), highly conserved protein that serves as recognition signal for protein degradation via the 26S proteasome pathway (Smalle and Vierstra 2004). The ubiquitin gene family contains two types of structures, polyubiquitin and ubiquitin extension protein genes. Polyubiquitin genes contain several tandem repeats of the ubiquitin-coding unit while the extension protein genes contain a single ubiquitin-coding unit fused in frame to the coding region for ribosomal proteins (Ozkaynak et al. 1987). Both types of genes are transcribed as gene fusions and translated as polyproteins that undergo rapid enzymatic hydrolysis to give free ubiquitin or ubiquitin and ribosomal proteins. To date, active polyubiquitin genes isolated from eukaryotes contain conserved architectural features. While these genes contain an intron between the 5′ untranslated region and the ATG start codon of the first ubiquitin monomer, in no cases are the polyprotein coding sequences interrupted by additional introns.
Promoter elements derived from polyubiquitin genes from a number of plant sources have been characterized. Previously described elements include those from Arabidopsis (Burke et al. 1988), maize (Christensen and Quail 1996), potato (Garbarino et al. 1995), tobacco (Plesse et al. 2001), rice (Wang et al. 2000), Gladiolus (Joung and Kamo 2006) and sunflower (Binet et al. 1989; Binet et al. 1991). These control elements have been employed in the expression of a wide variety of transgenes in monocots and dicots (Atkinson et al. 2004; Chen et al. 2004; Kang et al. 2006; Panahi et al. 2004; Ramesh et al. 2004; Rommens et al. 2004; Schultheiss et al. 2005; Tiimonen et al. 2005; Yang et al. 2007).
Of particular utility are transgenes in which coding regions are translationally fused to the first ubiquitin monomer (Garbarino et al. 1995; Hondred et al. 1999; Sivamani and Qu 2006). Not only are these translational fusions highly expressed (Sivamani and Qu 2006), they are efficiently processed by ubiquitin-specific proteases releasing the transgene product. In addition, this type of construct does not interfere with appropriate cellular protein targeting (Hondred et al. 1999).
Two new polyubiquitin genes from the wild potato species, Solanum bulbocastanum, are reported here. The first, bul427, is related to the potato ubi7 gene (Garbarino et al. 1995). The second, bul409, has similarity to a tomato polyubiquitin gene (Rollfinke et al. 1998). Control elements from both genes have potential applications in expressing transgenes in potato.
Materials and Methods
Plant Material
Solanum bulbocastanum and potato cv. Lenape (Akeley et al. 1968) were obtained from the Inter-Regional Potato Introduction Station (NRSP-6), Sturgeon Bay, Wisconsin. Plants were maintained in tissue culture or grown in a glasshouse in Albany, CA.
Isolation and Characterization of Polyubiquitin Genes
A S. bulbocastanum Bacterial Artificial Chromosome (BAC) library (Song et al. 2000) was probed with random primed (GE Healthcare) coding sequence from the potato polyubiquitin cDNA ubi9 (Garbarino et al. 1992). To identify unique polyubiquitin genes, DNA prepared (Qiagen) from positive clones was digested (BamHI, HinDIII and EcoR1), separated by agarose gel electrophoresis, blotted to charged nylon membranes and hybridized with the polyubiquitin probe described above. Hybridizing bands were subcloned into the plasmid BluescriptII SK(+) (Stratagene) for further characterization. Subcloned restriction fragments were subjected to DNA sequence analysis.
Pustell matrix analysis (Pustell and Kafatos 1982) was used to compare DNA sequences (MacVector8.0). Matrix alignments presented in the figures were downloaded from MacVector. Similar sequences in the available database were identified using the BLAST Network Service of the National Center for Biotechnology Information (Altschul et al. 1990).
cDNA Isolation
Total RNA was prepared from wounded S. bulbocastanum tubers as previously described (Verwoerd et al. 1989). Polyadenylated RNA was then isolated using magnetic beads (Promega Z5300 Polytract Isolation System), subjected to reverse transcriptase PCR (BD Biosciences Marathon cDNA Amplification Kit) for first stand synthesis, and amplification using bul427-specific primers (forward ATCCCCAAATCATCATCC and reverse AAAACAACAGAACATAACACATTACAAGGTCTCT). The 1.8 kb amplification products (Fisher Scientific Taq DNA Polymerase) were cloned into the pCR2.1 vector using the TA Cloning Kit (Invitrogen) and subjected to DNA sequence analysis.
Transgene Construction
Three chimeric genes were constructed. The first (bul409-GUS) contains the first 4.2 kb of the bul409 genomic clone indicated in Fig. 1 including the full-length promoter, intron and first ubiquitin monomer translationally fused to the GUS marker gene. Attempts to generate a full length promoter by PCR failed, potentially due to repeated domains within the intron. This construct was initiated by generating a 1.8 kb PCR product from the bul409 genomic subclone employing 5′ (CCGGATCCAATATTACTTAAATATCATGAAATAAACTA) and 3′ (CCGGATCCACCACCACGTAGACGG) primers as indicated in Fig. 1. Both primers contain 5′ BamHI sites, the location of the BamHI site on the 3′ primer allows construction of GUS translational fusions identical to those employed previously (Garbarino and Belknap 1994; Garbarino et al. 1995). The bul409-GUS transgene was constructed by fusing the 3′ end of bul409 (3.3 kb HindIII/XbaI, Fig. 1) to the 0.9 kb XbaI/BamHI from the above PCR product (containing 5′-UTR, intron, ubiquitin monomer) and the GUS-NOS (Agrobacterium nopaline synthase polyadenylation signal) sequence employed previously (Garbarino and Belknap 1994; Garbarino et al. 1995) in BluescriptII SK(+). The resulting transgene with a 3.4 kb promoter, 536 bp intron and ubiquitin monomer fused to GUS-NOS was then mobilized into the binary plant transformation vector pBINPlus/ARS (McCue et al. 2006).
Structures of S. bulbocastanum polyubiquitin genes bul409 and bul427. Sequences encoding ubiquitin monomers (black) and S-receptor protein kinase (gray) proteins are designated with large arrows with lines/arrows above indicating their associated transcripts indicated above. Shaded boxes indicate positions of 5′-UTR domains and introns indicated by hatched boxes. Small arrows indicate the locations of primer pairs for PCR amplification of promoters (1–2, 3–4) or reverse transcriptase PCR amplifications of cDNAs (5–6). Domains a–e indicate locations of flanking recombination events described in text: a transposon-related sequence; b and c repeats of unknown origin; d chloroplast genome sequences; e mitochondrial genome sequences
In the second transgene (bul409s-GUS, Fig. 4) 2.6 kb at the 5′ end of the bul409 promoter was deleted by fusing unique HinDIII and ScaI sites (Fig. 1) in the bul409-GUS binary plasmid, resulting in a 771 bp promoter and intron/ubiquitin sequences as in bul409-GUS.
The third transgene (bul427-GUS) was constructed by first amplifying the a 3 kb promoter fragment from the bul427 BAC clone using 5′ (GCTTGGTCTTACTTCATCGTC) and 3′ (CCGGATCCTCCACCACGTAGACGAAGG) primers and cloning the product into plasmid pCR2.1. The bul427 promoter product (1,278 bp promoter, 1555 bp intron and ubiquitin monomer) was used to construct a GUS translational fusion product with NOS terminator as described above (Fig. 4). The transgene was then mobilized into the binary plant transformation vector pBINPlus/ARS.
For relative promoter strength determination, the Cauliflower Mosaic Virus (CaMV) 35S-GUS-NOS transgene from pBI121 (Jefferson et al. 1986) was employed.
Transgenes were mobilized into potato cv. Lenape via Agrobacterium-mediated transformation (Snyder and Belknap 1993). Potato leaf and tuber tissues were wounded as described (Garbarino and Belknap 1994; Garbarino et al. 1995).
RNA Blots
Total RNA was prepared from control and wounded leaves and tubers as described previously (Garbarino et al. 1995). RNA was fractionated by agarose gel electrophoresis, and transferred to a nylon membrane and hybridized with a random primed (GE Healthcare) double stranded GUS probe.
GUS Assays
Leaf tissues were taken from 15-week-old plants from the greenhouse. Tuber tissue was obtained from freshly harvested mature plants. Individual transgenic lines were sampled in triplicate, and assayed for GUS activity (μkat mg protein−1) as described previously (Garbarino et al. 1995; Jefferson et al. 1986). Standard deviations were determined using Excel (Microsoft) and pairwise multiple comparison of means (Holm–Sidak method) in one-way ANOVA using SigmaStat (Jandel).
Results and Discussion
Structure of bul409 and bul427 Endogenous Genes
The coding sequence of a highly expressed potato polyubiquitin cDNA, ubi9 (Garbarino et al. 1992) was used to probe a BAC library containing genomic S. bulbocastanum DNA inserts. Hybridizing BACs were identified and characterized by restriction enzyme analysis. Two BACs displaying distinct ubiquitin-hybridizing restriction band profiles and patterns indicating a single ubiquitin-hybridizing locus were selected for further characterization. Both BACs contained approximately 100 kb inserts (Song et al. 2000) and polyubiquitin-hybridizing restriction fragments from each were subcloned and subjected to sequence analysis. The structures of the two genes are shown in Fig. 1.
The bul409 gene appears as a standard hexameric polyubiquitin gene. The 5′-UTR, coding sequence and 3′-UTR of this gene have considerable identity (>95%) to a tomato cDNA (BT012698) and to the potato ubi7 polyubiquitin cDNA (Garbarino et al. 1992). The bul409 and ubi7 promoter regions also share high (~80%) identity (Garbarino et al. 1995). In addition, the bul409 promoter region shown in Fig. 1 contains two repetitive domains found in Solanum BACs. Repeated domain “a” (Fig. 1) represents an apparent terminal inverted repeat flanking transposon found in S. demissum accession AC149301 (see related rice transposon; Fujino et al. 2005). The “b” repeated domain in Fig. 1 is of unknown origin and is found in a single S. demissum BAC accession (AC149287).
In contrast to bul409, the heptaubiquitin bul427 gene (Fig. 1) has a number of features suggesting it represents a pseudogene. This gene has significant similarity to the tomato heptaubiquitin gene LEPUR locus (accession X73156) (Rollfinke et al. 1998), While the bul427 promoter domain shares less than 80% identity with the similar LEPUR domain, these two genes share 96% identity in the coding sequence. The most striking feature of bul427 is the presence of a two bp deletion in the seventh ubiquitin monomer, resulting in a frame shift and truncated protein (Fig. 2). Several other features were consistent with bul427 representing a pseudogene. First, similarity to the LEPUR gene ends abruptly following the LEPUR polyadenylation site (Fig. 2) at an unusual sequence (the extent of this C-rich domain is unique in the available database) consistent with a rearrangement event. Second, the bul427 has a cluster of recombination events within 900 bp of the transcription start site (Fig. 1): “c”, an unknown element found in a variety of Solanum loci (GenBank accessions AC149266, AC149291, and AC144791); domain “d” is 92% identical to sequences 3′ the to chloroplast 16S rRNA (GenBank Accession AM087200); domain “e” has 80% identity to a sequence 5′ to the mitochondrial apocytochrome b gene (GenBank Accession AF095724). Finally, an apparent S-receptor protein kinase pseudogene is located in extremely close proximity (Fig. 1), 600 bp 3′ to the bul427 3′ UTR (89% identity to tomato EST GenBank Accession A1896901).
bul427-related cDNAs
In order to determine if bul427 is expressed, cDNAs related to the gene were amplified by reverse transcriptase PCR using primers indicated in Fig. 1. Two classes of transcripts were amplified; those with complete heptaubiquitin open reading frames and those with the 2bp deletion. Alignment of the 3′ ends of cDNAs from both classes to the bul427 genomic sequence is shown in Fig. 3. These data are consistent with expression of both bul427 and closely related polyubiquitin genes that are not interrupted by a deletion.
Alignment of bul427 genomic sequence flanking the frame shift mutation with two S. bulbocastanum polyubiquitin cDNAs. Dashes indicate relative deletions. Translations of the bul427 genomic DNA and the 427.4 cDNA are indicated. At 4526 on bul427 genomic sequence there is a mismatched base relative to cDNA 427.4 indicated with lower case letter. The binding site of the 3′ primer used to amplify the cDNAs is underlined
Transgene Expression Profiles
Three chimeric genes were constructed to evaluate the expression profiles of the bul409 and bul427 promoters. The transgenes included the introns and the first ubiquitin monomer translationally fused to the marker gene GUS (Jefferson et al. 1987) for optimized expression (Hondred et al. 1999; Sivamani and Qu 2006). The first transgene, bul409-GUS, contained the 4.2 kb of the bul409 genomic clone (Fig. 1) including the full length promoter, intron and first ubiquitin monomer. While this promoter directed efficient wound-induced expression in transgenic leaves and tubers (data not shown), its excessive size limits its applicability. For this reason, a second transgene, bul409s-GUS, (Fig. 4) employing 1.6 kb of the bul409 gene (771 bp promoter, intron and first ubiquitin monomer) was constructed. The third transgene (bul427-GUS) was constructed by amplifying a 3 kb promoter/intron/ubiquitin fragment from the bul427 BAC (Fig. 4).
Structures of promoter-GUS fusion transgenes. Ubiquitin coding sequences, introns, 5′-UTRs and recombination sites are as in Fig. 1. The GUS coding sequence translationally fused to the ubiquitin monomer and the NOS (Agrobacterium nopaline synthase) polyadenylation signal are indicated
In unwounded transgenic leaves, the bul409s promoter directs expression of GUS mRNA at levels similar to the CaMV35S promoter (Kay et al. 1987) (Fig. 5) and expression is increased by wounding. In contrast, levels of expression from the bul409s promoter are lower in unwounded tubers and significantly induced by injury (Fig. 6), similar to the ubi7 profile (Garbarino et al. 1995). The GUS mRNA levels in leaves of plants expressing the bul427-GUS transgene were significantly lower than observed with the CaMV35S promoter (Fig. 5). While expression of bul427-GUS in leaves was not wound-induced (Fig. 5), expression was strongly induced by wounding in tubers (Fig. 6).
Wound-regulated GUS mRNA levels in transgenic potato leaves. Total RNA was prepared from leaves, separated by agarose gel electrophoresis and stained with ethidium bromide (lower panel). RNA was transferred to a nylon membrane and hybridized to a probe for GUS. Time post injury is indicated and plant lines characterized include bul409s-GUS #14 (a high level expressing clone), bul427-GUS #1 and CaMV35S-GUS #8
Wound-regulated GUS mRNA expression in transgenic potato tubers. Total RNA was prepared from tubers, separated by agarose gel electrophoresis and stained with ethidium bromide (lower panel). RNA was transferred to a nylon membrane and hybridized to a probe for GUS. Time post injury is indicated and plant lines characterized include bul409s-GUS #14 (a high level expressing clone), bul427-GUS #1 and CaMV35S-GUS #8
GUS Expression
GUS assays were performed on three individual leaves or tubers for a series of transgenic isolates and the data is arranged by descending values for GUS activity in the leaves (Fig. 7). Each set of plant lines were grown, harvested and assayed with two independent CaMV35S-GUS lines for comparison (with the exception of bul409s-GUS#14 that was included in the bul427 set). As expected (Hondred et al. 1999; Sivamani and Qu 2006), GUS activity in transgenic plants expressing ubiquitin translational fusions was significantly higher than that of the CaMV35S transgenes when compared to the steady state GUS mRNA levels (Figs. 5, 6 and 7). The activity of bul427-GUS #1 in leaves was not significantly higher, but resulted from a much lower steady state mRNA level (Fig. 5). In bul409s-GUS leaves, the activity of the four highest lines (3, 12, 17 and 14) was significantly greater than the rest (P ≤ 0.001). The activity of the 4 lowest lines (9, 4, 27 and 24) was not significantly different than both of the CaMV35S-GUS lines. In bul409s-GUS tubers, the activity of the five highest lines (12, 17, 14, 13 and 5) was significantly different than the CaMV35S-GUS lines. In bul427-GUS leaves, the activity of only the highest line (22) was significantly different than both CaMV35S-GUS lines. In bul427-GUS tubers, the activity in all but the two lowest lines (29 and 2) was significantly higher than the CaMV35S-GUS lines. In both leaves and tubers, mean levels of GUS activity controlled by both promoters were comparable to, or up to 30-fold greater than observed with the CaMV35S promoter.
Conclusions
The bul427 gene contains structural features common to pseudogenes (Balakirev and Ayala 2003) including a frame shift mutation and numerous potential recombination events proximal to the coding sequence. However, these recombination events have not resulted in the inactivation of gene transcription (Figs. 3 and 7) and the frame shift mutation does not prevent the synthesis of active protein from the mRNA due to the unique duplicate polyprotein nature of polyubiquitin coding sequences. Rather than gene inactivation, the presence of the frame shift mutation in this case suggests a novel mechanism for fine-tuning the levels of accumulated gene product in cells.
The promoter employed in the construction of a transgene largely determines the magnitude and condition/tissue-specificity of its expression. In addition, plant promoter elements capable of directing levels of transgene expression suitable for commercial applications have generally proven difficult to isolate. The promoter elements derived from both the bul409 and bul427 polyubiquitin genes described here are capable of directing expression of transgenic protein at levels significantly greater than observed with the CaMV35S promoter (Fig. 7). These elements represent useful alternatives to currently employed promoter sequences, with specific utility in construction of transgenic potatoes containing only native Solanaceous DNA (Rommens et al. 2004).
Abbreviations
- GUS:
-
E. coli β-glucuronidase
- BAC:
-
bacterial artificial chromosome
- UTR:
-
untranslated region
References
Akeley, R.V., W.R. Mills, C.E. Cunningham, and J. Watts. 1968. Lenape: a new potato variety high in solids and chipping quality. American Potato Journal 45: 142–151.
Altschul, S.F., W. Gish, W. Miller, E.W. Myers, and D.J. Lipman. 1990. Basic local alignment search tool. Journal of Molecular Biology 215: 403–410.
Atkinson, H.J., S. Grimwood, K. Johnston, and J. Green. 2004. Prototype demonstration of transgenic resistance to the nematode Radopholus similis conferred on banana by a cystatin. Transgenic Research 13: 135–142.
Balakirev, E.S., and F.J. Ayala. 2003. Pseudogenes: are they “junk” or functional DNA? Annual Review of Genetics 37: 123–151.
Binet, M.N., A. Steinmetz, and L.H. Tessier. 1989. The primary structure of sunflower (Helianthus annuus) ubiquitin. Nucleic Acids Research 17: 2119.
Binet, M.N., J.H. Weil, and L.H. Tessier. 1991. Structure and expression of sunflower ubiquitin genes. Plant Molecular Biology 17: 395–407.
Burke, T.J., J. Callis, and R.D. Vierstra. 1988. Characterization of a polyubiquitin gene from Arabidopsis thaliana. Molecular Gen Genetics 213: 435–443.
Chen, T.L., Y.L. Lin, Y.L. Lee, N.S. Yang, and M.T. Chan. 2004. Expression of bioactive human interferon-gamma in transgenic rice cell suspension cultures. Transgenic Research 13: 499–510.
Christensen, A.H., and P.H. Quail. 1996. Ubiquitin promoter-based vectors for high-level expression of selectable and/or screenable marker genes in monocotyledonous plants. Transgenic Research 5: 213–218.
Fujino, K., H. Sekiguchi, and T. Kiguchi. 2005. Identification of an active transposon in intact rice plants. Molecular Genetics & Genomics 273: 150–157.
Garbarino, J.E., and W.R. Belknap. 1994. Isolation of a ubiquitin-ribosomal protein gene (ubi3) from potato and expression of its promoter in transgenic plants. Plant Molecular Biology 24: 119–127.
Garbarino, J.E., D.R. Rockhold, and W.R. Belknap. 1992. Expression of stress-responsive ubiquitin genes in potato tubers. Plant Molecular Biology 20: 235–244.
Garbarino, J.E., T. Oosumi, and W.R. Belknap. 1995. Isolation of a polyubiquitin promoter and its expression in transgenic potato plants. Plant Physiology 109: 1371–1378.
Hondred, D., J.M. Walker, D.E. Mathews, and R.D. Vierstra. 1999. Use of ubiquitin fusions to augment protein expression in transgenic plants. Plant Physiology 119: 713–724.
Jefferson, R.A., S.M. Burgess, and D. Hirsh. 1986. b-Glucuronidase from Escherichia coli as a gene-fusion marker. Proceedings of the National Academy of Sciences of the United States of America 83: 8447–8451.
Jefferson, R.A., T.A. Kavanagh, and M.W. Bevan. 1987. GUS Fusions: beta-glucuronidase as a sensitive and versatile gene fusion marker in higher plants. EMBO Journal 6: 3901–3907.
Joung, Y.H., and K. Kamo. 2006. Expression of a polyubiquitin promoter isolated from Gladiolus. Plant Cell Reports 25: 1081–1088.
Kang, T.J., B.G. Kim, J.Y. Yang, and M.S. Yang. 2006. Expression of a synthetic cholera toxin B subunit in tobacco using ubiquitin promoter and bar gene as a selectable marker. Molecular Biotechnology 32: 93–100.
Kay, R., A. Chan, M. Daly, and J. McPherson. 1987. Duplication of CaMV 35S promoter sequences creates a strong enhancer for plant genes. Science 236: 1299–1302.
McCue, K.F., P.V. Allen, L.V. Shepherd, A. Blake, J. Whitworth, M.M. Maccree, D.R. Rockhold, D. Stewart, H.V. Davies, and W.R. Belknap. 2006. The primary in vivo steroidal alkaloid glucosyltransferase from potato. Phytochemistry 67: 1590–1597.
Ozkaynak, E., D. Finley, M.J. Solomon, and A. Varshavsky. 1987. The yeast ubiquitin genes: a family of natural gene fusions. EMBO Journal 6: 1429–1439.
Panahi, M., Z. Alli, X. Cheng, L. Belbaraka, J. Belgoudi, R. Sardana, J. Phipps, and I. Altosaar. 2004. Recombinant protein expression plasmids optimized for industrial E. coli fermentation and plant systems produce biologically active human insulin-like growth factor-1 in transgenic rice and tobacco plants. Transgenic Research 13: 245–259.
Plesse, B., M.C. Criqui, A. Durr, Y. Parmentier, J. Fleck, and P. Genschik. 2001. Effects of the polyubiquitin gene Ubi. U4 leader intron and first ubiquitin monomer on reporter gene expression in Nicotiana tabacum. Plant Molecular Biology 45: 655–667.
Pustell, J., and F.C. Kafatos. 1982. A high speed, high capacity homology matrix: zooming through SV40 and polyoma. Nucleic Acids Research 10: 4765–4782.
Ramesh, S.A., S. Choimes, and D.P. Schachtman. 2004. Over-expression of an Arabidopsis zinc transporter in Hordeum vulgare increases short-term zinc uptake after zinc deprivation and seed zinc content. Plant Molecular Biology 54: 373–385.
Rollfinke, I.K., M.V. Silber, and U.M. Pfitzner. 1998. Characterization and expression of a heptaubiquitin gene from tomato. Gene 211: 267–276.
Rommens, C.M., J.M. Humara, J. Ye, H. Yan, C. Richael, L. Zhang, R. Perry, and K. Swords. 2004. Crop improvement through modification of the plant’s own genome. Plant Physiology 135: 421–431.
Schultheiss, H., G. Hensel, J. Imani, S. Broeders, U. Sonnewald, K.H. Kogel, J. Kumlehn, and R. Huckelhoven. 2005. Ectopic expression of constitutively activated RACB in barley enhances susceptibility to powdery mildew and abiotic stress. Plant Physiology 139: 353–362.
Sivamani, E., and R. Qu. 2006. Expression enhancement of a rice polyubiquitin gene promoter. Plant Molecular Biology 60: 225–239.
Smalle, J., and R.D. Vierstra. 2004. The ubiquitin 26S proteasome proteolytic pathway. Annual Review of Plant Biology 55: 555–590.
Snyder, G.W., and W.R. Belknap. 1993. A modified method for routine Agrobacterium-mediated transformation of in vitro grown potato microtubers. Plant Cell Reports 12: 324–327.
Song, J., F. Dong, and J. Jiang. 2000. Construction of a bacterial artificial chromosome (BAC) library for potato molecular cytogenetics research. Genome 43: 199–204.
Tiimonen, H., T. Aronen, T. Laakso, P. Saranpaa, V. Chiang, T. Ylioja, H. Roininen, and H. Haggman. 2005. Does lignin modification affect feeding preference or growth performance of insect herbivores in transgenic silver birch (Betula pendula Roth)? Planta 222: 699–708.
Verwoerd, T.C., B.M.M. Dekker, and A. Hoekema. 1989. A small-scale procedure for the rapid isolation of plant RNAs. Nucleic Acids Research 17: 2362.
Wang, J., J. Jiang, and J.H. Oard. 2000. Structure, expression and promoter activity of two polyubiquitin genes from rice (Oryza sativa L.). Plant Science 156: 201–211.
Yang, Z.Q., Q.Q. Liu, Z.M. Pan, H.X. Yu, and X.A. Jiao. 2007. Expression of the fusion glycoprotein of Newcastle disease virus in transgenic rice and its immunogenicity in mice. Vaccine 25: 591–598.
Acknowledgements
The authors acknowledge funding by the Agricultural Research Service National Programs (Project Number 5325-21420-001-00).
Author information
Authors and Affiliations
Corresponding author
Additional information
The mention of a trademark or proprietary product does not constitute a guarantee or warranty of the product by the US Department of Agriculture and does not imply its approval to the exclusion of other products that may be suitable.
Rights and permissions
About this article
Cite this article
Rockhold, D.R., Chang, S., Taylor, N. et al. Structure of Two Solanum bulbocastanum Polyubiquitin Genes and Expression of Their Promoters in Transgenic Potatoes. Am. J. Pot Res 85, 219–226 (2008). https://doi.org/10.1007/s12230-008-9015-5
Published:
Issue Date:
DOI: https://doi.org/10.1007/s12230-008-9015-5