Abstract
Ab initio and DFT methods have been employed to study the hydrogen bonding ability of formamide, urea, urea monoxide, thioformamide, thiourea and thiourea monoxide with one water molecule and the homodimers of the selected molecules. The stabilization energies associated with themonohydrated adducts and homodimers’ formation were evaluated at B3LYP/6-311++G** and MP2/6-311++G** levels. The energies were corrected for zero-point vibrational energies and basis set superposition error using counterpoise method. Atoms in molecules study has been carried out in order to characterize the hydrogen bonds through the changes in electron density and laplacian of electron density. A natural energy decomposition and natural bond orbital analysis was performed to understand the nature of hydrogen bonding.

Sixteen hydrogen bonded adducts of formamide, urea and urea monoxide with one water molecule and their homodimers have been optimized at B3LYP/6-311++G** and MP2/6-311++G** levels. Monohydrated Adducts and homodimers formation with the corresponding thio-analogs were also studied for comparative purpose. Atoms in molecules study has been carried out in order to characterize the hydrogen bonds. A natural energy decomposition and natural bond orbital analysis were performed to understand the nature of hydrogen bonding.
Similar content being viewed by others
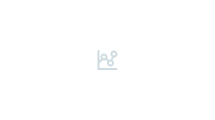
Avoid common mistakes on your manuscript.
1 Introduction
Intermolecular interactions like van der Waals or hydrogen bonding have drawn attention of many scientific groups due to their importance in physical, chemical and biological fields.[1–4]Formamide-water complex provides the simplest model for the hydration of proteins. Due to the simplicity of this model, it has been subjected to a large number of theoretical and experimental studies.[5–17]Formamide-water complex also plays an essential role in the properties of various materials such as synthetic polymers, biomolecules, molecular solids and fluids.[18–21]Urea and thiourea are widely used in the production of some pharmaceuticals (sulfothiazoles, barbiturates) and as an additive to some plastic materials.[22] Zhang et al. used radial distribution functions, statistics of hydrogen bonding networks and the viscosities to study the interactions and structures of urea-water system by an all-atom molecular dynamics simulation. They concluded that urea molecule shows the tendency to self-aggregate with increasing mole fraction of urea.[23]Many theoretical and experimental investigations performed on this urea-water system.[24–35]The stabilities of thiourea-water complexes were investigated theoretically as well.[36]
Thiourea and its oxides were extensively used in various industrial productions such as in photosensitive materials, medicine, spices and the recycle of precious metals.[37–41]The Jacobsen group made the most remarkable advances in the domain of urea and thiourea catalysts.[42] Thiourea and thiourea oxides are also valuable for fundamental scientific researches, especially in the study of non-linear reaction dynamics.[43] The studies on the kinetics and mechanisms of thiourea oxidation have attracted a great deal of attention.[44,45]These investigations have led to a general conclusion that the oxidation of thiourea goes through S-oxygenation to form sulfenyl, sulfinic and sulfonic acids and finally sulfate ions. The R-naphthylthiourea and phenylthiourea are pulmonary toxins to rats. Thiourea causes liver and thyroid tumors in rats.[46,47]Theoretical study on interactions between thiourea monoxide and water has been carried out to understand its stability in water.[48]
A variety of experimental and theoretical methods have been used to study the hydrogen bonding of homodimers. Formamide homodimers is important as a simple model for establishing the characteristics of N-H⋯O=C interaction present in a wide variety of biological systems.[49–51]These interactions have been studied by both theoretical and experimental methods.[52–60]For comparisons, the analysis of similar homodimers in case of thioformamide have also been studied.[60] Several groups have studied urea homodimer interactions theoretically.[61–64]The formation of urea homodimers and higher aggregates was confirmed from osmotic pressure measurements[65] and some evidence of urea homodimer pairing in solution emerged at higher concentrations emerged from X-ray study.[66] Present study systematically investigates the hydrogen bonding interactions that plays a significant role in stabilizing the monohydrated adducts of formamide, urea, urea monoxide, thioformamide, thiourea and thiourea monoxide with the aid of AIM, NEDA and NBO analysis. In this work, we have also conducted ab initio and DFT studies of the interaction in formamide, urea, urea monoxide, thioformamide, thiourea and thiourea monoxide homodimers.
2 Computational Details
All the calculations carried out with the Gaussian 09 package.[67] The theoretical methods employed were Density Functional Theory (DFT) and ab initio molecular orbital method.[68,69]The geometries of isolated formamide (F), urea (U), urea monoxide (UO), thioformamide (TF), thiourea (TU) and thiourea monoxide (TUS) and their corresponding 1:1 water adducts have been fully optimized at B3LYP/6-311 ++G** [L1] and MP2/6-311 ++G** [L2] levels (geometrical parameters are given in supplementary information TS1–TS22). The optimized orientations of the adducts of the molecule with water obtained at MP2/6-311 ++G** [L2] level are shown in figure 1. The homodimers of selected molecules were also optimized using above-mentioned theoretical methods. The geometrical parameters of optimized homodimers are reported in supplementary information TS23–TS38. The stabilization energy (ΔE) calculated as the difference of the total energy of the monohydrated adducts or homodimers and the sum of the isolated monomers in their minima configurations. The zero-point vibrational energy (ZPE) with basis set superposition error (BSSE) corrected stabilization energy (ΔE Corr) for all of the monohydrated adducts and homodimers were calculated at B3LYP/6-311 ++G**[L1] and MP2/6-311 ++G** [L2] levels. The counterpoise method proposed by Boys and Bernardi used to estimate the BSSE.[70]
The scaling factor of 0.96 and 0.95 was used for ZPE at B3LYP/6-311 ++G**[L1] and MP2/6-311 ++G** [L2] levels respectively.[71] The atoms in molecules (AIM) calculations were performed using the AIM2000 program.[72,73]The natural energy decomposition analysis (NEDA)[74–76] calculations were performed with the NBO 5.9[77,78]program linked to the GAMESS[79] package. A natural bond orbital (NBO) analysis were carried out using the NBO package included in the Gaussian 09 suite of programs.[80,81] The geometrical parameters and ΔE Corr values obtained at MP2/6-311 ++G** [L2] level are considered for discussion unless and otherwise mentioned.
3 Results and Discussion
3.1 AIM analysis
The analysis of topology of electron density using AIM has been used extensively to locate the hydrogen bonds. The values of electron density (ρ) at bond critical point (BCP) and its laplacian (\(\nabla _{\rho }^{2}\)) are important quantities to characterize hydrogen bond’s strength and its nature. The value of topological properties at BCPs characterizing the hydrogen bonds in monohydrate adducts and homodimers are reported in supplementary information table TS39–TS40. The ρ values at BCP in monohydrate adducts for molecules containing oxygen are in the range 0.014–0.042 au and the similar range for the monohydrate adducts of thio-analogs is 0.008–0.035 au. Relatively lower range of ρ values point towards the comparatively weak nature of hydrogen interactions in latter adducts. The \(\nabla _{\rho }^{2}\) indicating whether the electron density is locally concentrated (\(\nabla _{\rho }^{2} < 0\)) or depleted (\(\nabla _{\rho }^{2} > 0\)) for all the hydrogen bonded monohydrate adducts shown in table TS39 range from 0.052–0.160 au and from 0.020–0.104 au for oxo- and thio-adducts, respectively. The values for \(\nabla _{\rho }^{2}\) are clearly positive, as expected for the hydrogen bond. For homodimers of molecules containing oxygen ρ values at BCPs falls in the range of 0.011–0.032 au, whereas homodimers of thio-analogs falls in the range of 0.010–0.028 au and the values of ∇2 ρ at the BCPs are positive and well within the range suggested for hydrogen bonds (table TS40). The ρ and \(\nabla _{\rho }^{2}\) at BCP are smaller for the H⋯S bonds than that for H⋯O bonds in monohydrated adducts and homodimers. These values of ρ and \(\nabla _{\rho }^{2} \) fall within the range specified for the existence of the hydrogen bond in terms of ρ (0.002–0.040 au) and its \(\nabla _{\rho }^{2} \) (0.024–0.139 au) as suggested by Koch and Popelier with a few exceptions.[82,83]
3.2 Important structural changes and stabilization energies
Full geometrical optimizations of formamide, urea, urea monoxide, thioformamide, thiourea and thiourea monoxide and their adducts with single water have been carried out at B3LYP/6-311 ++G** [L1] and MP2/6-311 ++G** [L2] theoretical levels. The hydrogen bond distances and angles at the bridging hydrogen along with the stabilization energies are recorded in table 1. It was observed that the stabilization energies evaluated at MP2/6-311 ++G** [L2] level fall in the range of 2.73–9.29 kcal/mol and are consistently lower than the values obtained at B3LYP/6-311 ++G** [L1] which fall in the range of 3.64–10.54 kcal/mol theoretical level. Three different conformations FW 1 , FW 2 and FW 3 for the formamide-water adduct have been optimized and are shown in figure 1. FW 1 is the most stabilized adduct with oxygen of formamide as the hydrogen bond acceptor and N-H as hydrogen bond donor to water. It is perceived that the adduct FW 1 has two hydrogen bonds with lengths (O1⋯H8) and (O7⋯H6) of 1.947 Å and 2.060 Å and angles of 145.30 ∘ and 136.40 ∘ respectively with ΔE Corr of 6.34 kcal/mol. The difference in hydrogen bond distances from the respective sum of van der Waals radii and the ρ values at the BCP of two hydrogen bonds suggest both the hydrogen bonds play significant role in stabilizing the adduct. Experimental results reported by Langley et al.,[84] include the hydrogen bond distance and angles in the formamide-water adduct in FW 1 conformation as (O⋯H-O = 1.902 Å, ∠O-H-O = 151.1 ∘, O⋯H-N = 2.002 Å, ∠O-H-N = 141.5 ∘) which matches with the values obtained at MP2/6-311 ++G** [L2] levels with small variations. The other two adducts of formamide with water FW 2 and FW 3 are stabilized through single hydrogen bond as reflected by the geometrical parameters in table 1 and AIM analysis (table TS39) and thereby, their stabilization energies are relatively lower. The hydrogen bond distances in FW 2 and FW 3 are O7⋯H5 and O1⋯H7 are 1.995 Å and 1.900 Å and angles of 177.61 ∘ and 156.63 ∘, respectively with difference of 0.13 kcal/mol in ΔE Corr. The geometrical parameters for the hydrogen bond have an agreement with the experimentally reported values for similar adducts FW 2 (O⋯H-O = 1.981 Å, ∠O-H-O = 173.9 ∘) and FW 3 (O⋯H-O = 1.907 Å, ∠O-H-O = 156.1 ∘).[84]
The particulars of three adducts of thioformamide with water listed in table 1 also suggest that the most stabilized structure TFW 1 out of three is analogous to the most stable formamide-water adduct. The adduct TFW 1 is stabilized by two hydrogen bonds; S1⋯H8 (2.394 Å) and O7⋯H6 (1.940 Å) with ΔE Corr of 5.23 kcal/mol. The S and N-H of thioformamide act as hydrogen bond acceptor and hydrogen bond donor towards water molecule respectively and the ΔE Corr is 1.11 kcal/mol lower in comparison to that of FW 1 . The O7⋯H5 hydrogen bonded adduct TFW 2 , has hydrogen bond distance of 1.961 Å and angle of 176.57 ∘. Interestingly both TFW 2 and FW 2 involve single hydrogen bond N-H⋯O water interactions but the ΔE Corr of stabilization energy of TFW 2 is relatively higher (0.31 kcal/mol) supported by shorter hydrogen bond distance. During the MP2 and B3LYP calculations with the same basis set, the maximum stability difference among three conformation of formamide and thioformamide water adduct is for FW 1 and TFW 1 is 0.87 and 1.58 kcal/mol respectively.
Two conformations have been optimized for the adduct between urea and water in 1:1 ratio. Both UW 1 and UW 2 are stabilized by the two hydrogen bonds each. However, UW 1 with oxygen and N-H of urea as hydrogen bond acceptor and donor respectively towards water is 2 kcal/mol more stable relative to UW 2 wherein two N-H bonds of two amino groups act as hydrogen bond donor to oxygen of water. The two hydrogen bond distances in UW 1 are O1⋯H9 and O10⋯H7 are 1.906 Å and 2.060 Å with angles 149.94 ∘ and 142.70 ∘ respectively. For UW 2 adduct, oxygen of water acts as hydrogen bond acceptor to N4-H8 and N3-H6 of urea with the hydrogen bond distances O9⋯H8 (2.240 Å) and O9⋯H6 (2.200Å) and angles of 142.00 ∘ and 145.78 ∘. The TUW 1 being analogous to UW 1 has ΔE Corr of 5.67 kcal/mol while TUW 2 has ΔE Corr of 5.35 kcal/mol. The maximum difference for ΔE Corr for both selected theoretical methods for UW 1 and TUW 1 is 0.83 and 1.61 kcal/mol respectively.
In case of urea monoxide, the attempt to optimize the adduct with hydrogen bonding to O1 reveals adduct UOW 1 , wherein there is intramolecular N5-H8⋯O1 hydrogen bond along with O1⋯H10-O11 intermolecular hydrogen bond which exhibits the ΔE Corr of 8.59 kcal/mol. The hydrogen bond distances in the two hydrogen bonds are 1.730 Å and 1.930 Å respectively with angles of 158.01 ∘ and 111.98 ∘ respectively. The order of stability for most stable adducts of formamide, urea and urea monoxide is UOW 1 >UW 1 >FW 1 . The thio-analog TUSW 1 reflects six-membered cyclic structure with the presence of two intermolecular O1⋯H10 and O11⋯H8 hydrogen bonds with bond distances 1.710 Å and 1.839 Å. Both the bonds are relatively stronger, as suggested by geometrical parameters and ΔE Corr. The TUSW 1 adduct is 0.70 kcal/mol more stabilized than UOW 1 .
The O2 and H6-N4 bond of urea monoxide acts as hydrogen acceptor, and hydrogen bond donor respectively toward water in UOW 2 with hydrogen bond distances of 2.200 Å and 1.960 Å and angles of 122.95 ∘ and 151.40 ∘ respectively. The N4-H6⋯O11 hydrogen bond in TUSW 2 has a bond distance of 1.970 Å with an angle of 167.15 ∘ and ΔE Corr of 4.28 kcal/mol. Though the hydrogen bond distance for S2⋯H10 is longer than the sum of van der Waals radii of the two participating atoms, the presence of hydrogen bond is indicated by the BCP (table TS39). Three hydrogen bond interactions are located in the adducts UOW 3 and TUSW 3 with the bifurcated hydrogen bonding N-H⋯O⋯H-N interaction in which the water acts as hydrogen bond acceptor and urea monoxide or thiourea monoxide acts as hydrogen bond donor and one intramolecular hydrogen bond. The UOW 3 adduct with bond distance (O1⋯H8) 1.789 Å, (O10⋯H7) 2.154 Å and (O10⋯H9) 2.180 Å and angles of 116.49 ∘, 145.71 ∘ and 142.18 ∘ respectively has ΔE Corr of 6.50 kcal/mol. TUSW 3 O1 ⋅⋅ H8, O10⋯H7 and O10⋯H9 are 2.031 Å, 2.185 Å and 2.220 Å with angles of 117.40 ∘, 142.40 ∘ and 137.70 ∘, respectively. The adduct UOW 3 is 0.92 kcal/mol more stabilized than TUSW 3 . The order of stabilization for urea monoxide-water adducts is UOW 1 >UOW 2 >UOW 3 whereas for thiourea monoxide-water adduct is TUSW 1 >TUSW 3 >TUSW 2 . In the adducts of urea monoxide and their thio-analogs, the B3LYP calculations show a tendency to give a larger value for the ΔE Corr compared to the MP2 values with difference falls in range 0.10–3.5 kcal/mol.
For comparing the hydrogen bond strength and hydrogen bonding ability of the molecules with themselves, the homodimers of the molecules have also been studied. The optimization of homodimers of the selected molecules have been carried out at B3LYP/6-311 ++G** [L1] and MP2/6-311 ++G** [L2] theoretical levels. The presence of hydrogen bonds in the homodimers is confirmed through location of BCP with AIM studies (table TS40). The results depict that the stabilization energies for optimized homodimers calculated at MP2/6-311 ++G** [L2] method are higher than those obtained using B3LYP/6-311 ++G** [L1] method. The optimization of homodimers of formamide revealed the presence of five minima. Figure 2 shows the minima for formamide homodimer and table 2 summarizes their structural parameters important for hydrogen bonding. The most stable DF 1 , which correspond to planar cyclic structure involving two hydrogen bonds N-H⋯O=C with hydrogen bond distance 1.890 Å and 1.880 Å and nearly same hydrogen bond angle 172.8 ∘ with ΔE Corr 12.34 kcal/mol. The planar DF 2 comprise of hydrogen bonds N-H⋯O=C and C-H⋯O=C with bonding distance of 2.316 Å and 1.924 Å and angles of 141.23 ∘ and 167.20 ∘ respectively result in relatively lower ΔE Corr value of 8.54 kcal/mol while the homodimers DF 3 and DF 4 are non-planar. The presence of single hydrogen bond in DF 4 and the two hydrogen bonds in DF 3 show larger deviation from linearity and the stabilization associated with these homodimers are comparatively lower. The planar homodimer DF 5 with ΔE Corr of 5.01 kcal/mol is stabilized by O1⋯H9 and H6⋯O7 where the hydrogen bond distances and angles are (2.389 Å and 2.387 Å) and (138.68 ∘ and 138.75 ∘) respectively. The stability order for formamide dimer is DF 1 >DF 2 >DF 3 >DF 4 >DF 5 .
Figure 2 also depicts the structure corresponding to the minima on the potential surface of thioformamide dimers and table 2 lists their structural parameters important for hydrogen bonding. The structures are similar to those of formamide homodimer and follow the same stability sequence. Again, the most stable structure occurs in a conformation DTF 1 where the two molecules form two equivalent N-H⋯S=C hydrogen bonds that results in ΔE Corr of 10.50 kcal/mol with each bond distance 2.382 Å and angle 171.0 ∘ nearly same. DTF 2 forms two hydrogen bonds N-H⋯S=C and C-H⋯S=C with ΔE Corr of 8.35 kcal/mol defined by the hydrogen bond distance H4⋯S7 of 2.400 Å and angle 159.66 ∘ in the former bond. The position of S1 and H9 does not fall within their sum of van der Waals radii with angle of 106.35 ∘, but the presence of the BCP (table TS40) reflects presence of electrostatic interactions between the two atoms. The homodimer DTF 3 and DTF 4 are stabilized with ΔE Corr of 6.25 and 5.63 kcal/mol respectively. The hydrogen bond distances in DTF 3 , H5 ⋅⋅S7 and N3⋯H12 are 2.492 Å and 2.319 Å with angles of 148.06 ∘ and 146.54 ∘ respectively. Whereas DTF 4 is stabilized with single hydrogen bond with bond distance 2.449 Å and angle 151.24 ∘. DTF 5 and DF 5 have symmetrically hydrogen bonded cyclic structure involving S⋯H-C in the former and O⋯H-C hydrogen bonds in the latter but the former dimer is 0.23 kcal/mol more stabilized. The formamide homodimers are more stabilized than thioformamide with two exceptions (DF 3 , DTF 3 ) and ((DF 5 , DTF 5 ). The homodimers of formamide and thioformamide are more stable than their monohydrated adducts respectively. The most stable DF 1 is 6.0 kcal/mol more stabilized as compared to FW 1 adduct whereas formation of DTF 1 is 5.27 kcal/mol more strengthened in contrast to TFW 1 . In the homodimers of formamide and their thio-analogs, the B3LYP calculations often produce a lower value for the ΔE Corr on comparing to the MP2 values.
Two conformations for the energy minimum structure have been obtained in case of urea homodimer, and these structures are reported in figure 2. These structures adorn two hydrogen bonds each. In DU 1 , one of the N-H of each monomer unit acts as hydrogen bond donor towards the carbonyl oxygen as hydrogen bond acceptor. The distances and angles of each hydrogen bonds O1⋯H15 and H5⋯O9 are 1.864 Å and angles 176.22 ∘ nearly same. The DU 2 dimer involves two N-H⋯O=C and N-H⋯N-C hydrogen bonds with distances 1.994 Å and 2.129 Å and angles 150.97 ∘ and 155.24 ∘ respectively. The differences in ΔE Corr of homodimers DU 1 and DU 2 is 2.06 kcal/mol with the stability order DU 1 >DU 2 . The homodimers of thiourea labeled as DTU 1 and DTU 2 that have conformation orientation similar to DU 1 and DU 2 respectively are also placed in figure 2. The DTU 1 has two equivalent hydrogen bonds (N-H⋯S) with hydrogen bond distance and angle of 2.365 Å and 169.26 ∘ respectively nearly same. However, the DTU 1 is 0.59 kcal/mol more stabilized than the DU 1 . The DU 1 is 4.72 kcal/mol more stabilized as compared to UW 1 whereas DTU 1 is 5.81kcal/mol more stabilized as compared to TUW 1 adduct.
The DUO 1 with single N-H⋯O bond distance and angle of 1.889 Å and 169.56 ∘ stabilized by ΔE Corr of 11.05 kcal/mol. Similar conformation of homodimer DTUS 1 is obtained for thiourea monoxide with ΔE Corr of 10.25 kcal/mol with hydrogen bond distance of 1.933 Å and angle of 150.05 ∘. The stability order is as follows DUO 1 >DTUS 1 . It is found that stability difference for homodimer of urea monoxide and thiourea monoxide is about 2.55–2.75 kcal/mol for two selected methods. The DUO 1 is 2.46 kcal/mol more stabilized as compared to most stable UOW 1 adduct while other adducts, UOW 2 and UOW 3 are 4.38–4.55 kcal/mol less stabilized. The DTUS 1 is 0.96 kcal/mol more stabilized as compared to most stable TUSW 1 adduct while other adducts, TUSW 2 and TUSW 3 are 4.67–5.97 kcal/mol less stabilized.
3.3 NEDA analysis
To better understand the nature and relative stability arising from components contributing to the intermolecular attraction in these monohydrated adducts, individual energy components {static polarization (ES), induced polarization (PL), self energy correction at each polarizing centre (SE)}, collectively referred as electrical components (EL) were obtained using NEDA procedures, where EL = ES + PL + SE. The EL component describes both static and induced interactions between monomer charge densities, generally compared favourably with estimates from classical point multipole/polarizability approximations. The charge transfer (CT) component describes delocalizing, donor-acceptor interactions. CORE accounts for the quantum mechanical effects (Pauli repulsions and exchange) of filled orbital (σ- σ) interactions that arise from the required antisymmetry of the wave function for the interacting monomers.[85] The evaluated components at B3LYP/6-311 ++G** [L1] theoretical level are listed in table 3.
As can be seen from table 3, the ES components of most of the adducts are higher than CT component with the exception of four monohydrated adducts TFW 1 , TUW 1 , UOW 1 and TUSW 1 . The adduct TUSW 1 shows a difference of 5.57 kcal/mol between ES and CT while in other three cases the difference is less than 1.22 kcal/mol. The highest ES component is observed for TUSW 1 amongst all the adducts under study. The adduct FW 1 , the most stable of formamide-water adducts has highest ES component amongst the three, while in case of its thio-analogs TFW 1 , though the ES is relatively highest amongst the three conformation, CT component is larger than ES. Results in table reflect that in addition to attractive interactions, EL and CT add significantly to stability and CORE component. This plays an important role in overall stabilization energy. The CT component is more favourable in TFW 1 , but high CORE component makes it overall less stabilized in comparison to FW 1 . The ES component for FW 1 is 27.3% of total attractive interactions. In spite of low polarity of C-S bond in TFW 1 , in comparison to C-O bond in FW 1 , the comparable ES component in the two cases indicates favourable placement of other dipoles. The lowest ES component is indicated for the adduct FW 2 . Though both FW 2 and TFW 2 have N3-H5⋯O7 hydrogen bond, the hydrogen bond angles and the atomic charges on the hydrogen bond donor and acceptor in the two cases also show negligible difference, the higher ES associated with TFW 2 relative to FW 2 results from closer proximity of hydrogen bond donor and acceptor in case of TFW 2 . It is interesting to note that in spite of low magnitude of atomic charge on S, the EL component in case of thio-analogs is comparable to that observed for oxo-carbonyl compounds with the exception of two adducts.
The EL and CT component explains higher stability of UW 1 and TUW 1 as compared to FW 1 and TFW 1 respectively. In fact, in case of UOW 1 and TUSW 1 pair, the ES component is higher in the latter and the huge difference can be traced to the presence of highly electronegative oxygen in thiourea monoxide. In case of TUSW 1 , S does not act as hydrogen bond acceptor but favours in the electron delocalization through polarization of S-O bond as well comparatively larger CT component. In spite of the higher polarizability of sulfur relative to oxygen, the PL component is higher in oxygen adducts (FW 1 , FW 3 , UW 1 , UOW 2 and UOW 3 ) and the high electronegative nature of oxygen enhances EL component in these adducts relative to the adducts of sulfur. The order of stability for most stable adducts of formamide, urea and urea monoxide is UOW 1 >UW 1 >FW 1 is supported by CT and EL from NEDA analysis.
3.4 Atomic charge analysis
The atomic charges are reflective of the electrostatic component. The values of atomic charges of hydrogen bond acceptor and hydrogen bond donor are analyzed from NBO obtained at B3LYP/6-311 ++G** [L1] theoretical level. The atomic charges for all the sixteen monohydrated adduct with water are reported in tables TS41–TS42. Atomic charge analysis indicates that the charge on S1 is comparatively much smaller in magnitude than on O1 in the adducts of thioformamide and formamide respectively. Hence, the contribution of electrostatic interactions in adducts involving sulfur of thioformamide as hydrogen bond acceptor is expected to be smaller in comparison to oxygen of formamide. In monohydrated adducts, the oxygen of water is more electron dense than the oxygen of formamide but polarity of O-H of water favours the carbonyl oxygen to act as hydrogen bond acceptor.
The decrease in electron density on hydrogen atom of water involved in hydrogen bonding has been accepted as one of the criteria for hydrogen bond and clearly shows that the hydrogen nuclei are deshielded upon hydrogen bond formation. The analysis of atomic charges on atoms involved in hydrogen bond formation in table TS41–TS42 reflects that the hydrogen atoms of the hydrogen bond donors have charge in the range 0.203–0.509 au. In addition, the charge density on the atom acting as hydrogen bond acceptor is increased. The NEDA study indicated that ES components of thio-analogs of formamide, urea and urea monoxide have comparative value with few exceptions.
Nearly all the atoms in the urea and thiourea undergo variation in atomic charge density upon adduct formation with water in UW 1 and TUW 1 orientations. Relatively high variation in atomic charge on atoms H5, H6 and H8 is observed. The most stable adduct of urea monoxide with water UOW 1 , reflects large atomic charge variations on O1, C3 and both the N atoms. The addition of water molecule to thiourea monoxide increases charge on C atom and decreases that on S atom, which indicates that with the addition of water molecule, the polarity of C-S bond becomes larger. These results are supported by the molecular electrostatic potential (MEP maps) calculated using Molekel 4.3 version on molecules and their adducts with water under study at B3LYP/6-311 ++G** [L1] theoretical level shown in figure S1. The red region indicates the most negative potential Vmin while blue region indicates the positive potential Vmax. As can be seen from the figure, sulfur and oxygen involved in adducts under study exhibits negative electrostatic potential and hydrogen exhibits positive electrostatic potential. The electrostatic potential on oxygen is 62.75 kcal/mol while for sulfur is 53.33 kcal/mol reflects higher stability of adducts of formamide with water relative to thioformamide adducts.
The atomic charges for all the sixteen homodimers are reported in tables TS41–TS42. Atomic charge analysis indicates that the charge on S1 is relatively −0.427 to −0.515 au lower in magnitude than on O1 in homodimers of thioformamide and formamide respectively. This shows large electrostatic contribution to the total energy in formamide homodimers under study, which dominates over all other stabilizing interactions. However, in case of formamide and thioformamide homodimers, the small difference in stabilization energies is indicated in the results. Polarizations of all the bonds are increased in DF 1 and DTF 1 . However, variations in DF 1 are larger at the hydrogen bond acceptor and hydrogen bond donor group. Atomic charge variations in DU 1 also suggest change in polarization of bonds on dimer formation. However, high atomic charge values caused by the presence of the two –NH2 group, the electrostatic interactions are anticipated to be higher in DU 1 relative to DF 1 . The thio-analogs of urea, also reflects change in bond polarities upon dimer formation. However, the variations are relatively smaller in comparison to DU 1 formation.
3.5 NBO analysis
The second order interactions energies (E (2)) of relevant orbital interactions associated with hydrogen bond donor and acceptor from NBO analysis obtained at B3LYP/6-311 ++G** [L1] theoretical level are reflective of the charge transfer between the monomeric units in monohydrated adducts and are recorded in table 4. The occupancies of the acceptor orbitals are indicative of extent of charge transfer and are also included in the table 4. As can be seen in case of FW 1 , the orbital interactions nO1→σ*H8-O7 and nOw→σ*N-H stabilize the adduct with E (2) values of 6.91 and 3.58 kcal/mol. The E (2) values also suggest that former interactions are larger in comparison to latter. Hence, strength of O1⋯H8-O7 is relatively stronger, which is also reflected by the ρ value of the BCP and the hydrogen bond length. The hydrogen bonded FW 2 and FW 3 have nO7→σ*N3-H5 and nO1→σ*O8−H7with E (2) values 6.65 and 7.43 kcal/mol. The E (2) values for both the hydrogen bonds in TFW 1 are higher in comparison to the respective values present in FW 1 which suggests CT component to be relatively higher which indeed is reflected in NEDA analysis as well.
The CT in case of UW 1 is indicated to be higher in relation to that in FW 1 by the E (2) values. The NEDA also suggests similar results where EL and CT components favour UW 1 . The orbital interactions nO1→σ*H10-O11 with E (2) value of 17.85 kcal/mol strengthen the O1⋯H10 bond in UOW 1 while nO1→σ*H10-O11 and nO11→σ*H8-N5 orbital interactions with E (2) values of 10.21 and 15.77 kcal/mol strengthen the TUSW 1 adduct. The orbital interactions nO11→σ*H6-N4 in TUSW 2 , nO2→σ*H10-O11 and nO10→σ*H6-N4 in UOW 2 have E (2) values of 8.83, 1.40 and 8.02 kcal/mol respectively suggesting higher stabilization of UOW 2 adduct. The E (2) values in the adducts UOW 2 , UOW 3 , TUSW 2 , and TUSW 3 indicate that CT components are relatively lower than the respective values for UOW 1 and TUSW 1 . The E (2) values indicate that the covalent character of the hydrogen bonds in urea monoxide-water adducts follow the same order UOW 1 >UOW 2 >UOW 3 as that of stabilization energies. The order of stability for adducts UOW 1 >UW 1 >FW 1 is supported by CT.
Important second order stabilization energies for the orbital interactions strengthening the formation of homodimers at B3LYP/6-311 ++G** [L1] level are reported in table 5. In the most stable homodimer of formamide, DF 1 that have two N-H⋯O=C as hydrogen bonds, E (2) values for the orbital interactions nO1→σ*H12-N10 and nO7→σ*H4-N3 is 12.02 kcal/mol each which are higher in comparison to the E (2) values in FW 1 . Thus the larger stability of DF 1 can be assigned to better CT between the monomeric units. The E (2) values associated with orbital interactions among N-H⋯S=C in most stable DTF 1 is 11.63 kcal/mol nearly each which explains its higher stability in comparison to TFW 1 . The E (2) values for the orbital interactions in DF 2 are nO1→σ∗H9-C8 (3.56 kcal/mol) and nO7→σ∗H4-N3 (11.88 kcal/mol) indicating higher contribution of H4⋯O7 hydrogen bond as well supported by geometrical parameters for the hydrogen bond reported in table 2. In DTF 2 , the small E (2) values for nS1→σ*H9-C8 (4.98 kcal/mol) supporting the weak hydrogen bond S1⋯H9 also supported by geometrical parameters. However, the second hydrogen bond has nS7→σ*H4-N3 orbital interaction that gives rise to E (2) value of 11.12 kcal/mol. The stability of DF 3 is more supported by orbital interaction H5⋯O7 as suggested by E (2) value of nO7→σ∗H5-N3 (7.75 kcal/mol) and favoured by short hydrogen bond distance and more linearity as compared to N3⋯H12 hydrogen bond which possesses small E (2) value of nN3→σ*H12-N10 (1.72 kcal/mol). The E (2)values for nS7→σ*H5-N3 orbital interactions (9.70 kcal/mol) strengthen the S7⋯H5-N3 hydrogen bond in DTF 3 in comparison to small E (2) stabilization for N3⋯H12.
The strongly bound homodimers DU 1 and DTU 1 are supported by strong E (2) values for the two hydrogen bonds and can be seen in table 5. In DU 1, CT contribution arising from nO1→ σ*H15-N11 and nO9→σ*H5-N3 orbital interactions with E (2) values of 13.33 and 13.43 kcal/mol respectively. Similarly for nS1→σ*H15-N11 and nS9→σ*H5-N3 orbital interactions in DTU 1 , the E (2) values are 17.79 and 17.75 kcal/mol respectively indicating higher stability of DTU 1 in comparison to the DU 1 . Relatively weaker strengthening of hydrogen bond from the CT interactions occur in monohydrated adducts of urea and thiourea. As can be seen from table 5 in DUO 1 , the E (2) value of orbital interactions nO1→σ*H17-N14 for the hydrogen bond O1⋯H17 is 18.39 kcal/mol suggesting its high stability as compared to DTUS 1 with E (2) value of 9.05 kcal/mol. From the analysis of E (2) values, it is reflected that homodimer DUO 1 is 2.46 kcal/mol more stable as compared to the most stable monohydrated adduct UOW 1 also supported by geometrical parameters in tables 1 and 2 whereas for other conformation of monohydrate adducts is 4.38–4.55 kcal/mol less stable. The NBO analysis clearly states that better CT occurs between the monomers in homodimers, in comparison to the adducts with water. Important E (2) values for the orbital interactions that are explaining conjugation in adducts with water relative to monomer evaluated at B3LYP/6-311 ++G** [L1] level are reported in table TS46. It is reflected that conjugation in adducts is being enhanced on adduct formation suggesting their high stability. Similar enhancement in conjugation has been observed upon homodimeric formation reported in supporting information table TS47. The increment in E (2) values for conjugation is higher for homodimers relative to monohydrated adducts.
4 Conclusions
The hydrogen bonding ability of formamide, urea, urea monoxide and their thio-analogs have been analyzed by studying their adducts with water and the homodimers. The stabilization energies for the adduct between formamide, urea and urea monoxide with single water, range between 3.67–8.59 kcal/mol. While similar adducts with thio-analogs have the stabilization energy in the range 2.73–4.29 kcal/mol. Relatively stronger hydrogen bonds result in homodimers as reflected by range of stabilization energy 5.01–12.54 kcal/mol for formamide, urea and urea monoxide and 5.63–11.48 kcal/mol for thio-analogs. The most stable adduct of formamide and urea with water has two hydrogen bonds with carbonyl oxygen and N-H as hydrogen bond acceptor and donor respectively towards water, the most stable homodimer also adorn two hydrogen bonds with similar sites as hydrogen bond donor and hydrogen bond acceptor in each monomeric unit. But the difference in stability of the two can be assigned to better CT in the homodimers assisted by resonance interactions in the monomeric units as reflected by NBO analysis.
In case of thioformamide and thiourea, it is thiocarbonyl sulfur and N-H as hydrogen bond acceptor and donor respectively. Thus, when both the sites (thiocarbonyl sulfur and N-H) are simultaneously involved in hydrogen bonding, it results in most stable conformation observed from the study of hydrated molecules and homodimers. The CT in the most stable conformation of homodimer is high as compared to monohydrated adducts. However in case of urea monoxide and thiourea monoxide, the most stable adducts with water involve additional oxygen and N-H as hydrogen bond acceptor and donor, respectively. In this case, stability of the latter is higher in comparison to former, which has been rationalized in terms of two hydrogen bonds involving resonance in the six-membered cyclic structure, due to high charge density of oxygen arising from the presence of sulfur. Whereas, in their homodimer, only single hydrogen bond with additional oxygen of one monomer and N-H of other monomer. Oxo-analog of homodimer is more stabilized relative to its thio-analog.
References
Jeffrey G A 1997 In An Introduction to Hydrogen Bonding (USA: Oxford University Press)
Desiraju G R and Stenier T 1999 In The Weak Hydrogen Bond (Oxford: Oxford University Press)
Bandhopadhyay I, Lee H M and Kim K S 2005 J. Phys. Chem. A. 109 1720
Sobczyk L, Grabowski S and Krygowski T M 2005 Chem. Rev. 105 3513
Hinton J F and Harpool R D 1997 J. Am. Chem. Soc. 99 349
Jaeisen P G and Stevens W J 1986 J. Chem. Phys. 84 3271
Engdahl A and Nelander B 1993 J. Chem. Phys. 99 4894
Sim F and St-Amant A 1992 J. Am. Chem. Soc. 114 439
Wang X C, Facelli J C and Simons J 1993 Int. J. Quantum. Chem. 45 123
Liu T, Li H, Huang M, Duan Y and Wang Z 2008 J. Phys. Chem. A. 112 5436
Urban J J, Tillman B G and Cronin W A 2006 J. Phys. Chem. A. 110 11120
Pliego J R Jr 2004 Chem. Phy. 306 273
Cordeiro M A M, Santana W P, Cusinato R and Cordeiro J M M 2006 J. Mol. Struct. (THEOCHEM) 759 159
Sakai D, Mastuda Y, Hachiya M, Mori M, Fujii A and Mikami N 2008 J. Phys. Chem. A. 112 6840
Del Bene J E, Alkorta I and Elguero J 2008 J. Phys. Chem. A. 112 6338
Taha A N and True N S 2000 J. Phys. Chem. A. 104 2985
Angelina E L and Peruchena N M 2011 J. Phys. Chem. A. 115 4701
Espinosa E and Molins E 2000 J. Chem. Phys. 113 5686
Sunita S S, Rohini N K, Kulkarni M G, Nagaraju M and Sastry G N 2006 J. Am. Chem. Soc. 128 7752
Sunita S S, Rohini N K, Kulkarni M G, Nagaraju M and Sastry G N 2007 Macromolecules 40 1824
Nagaraju M and Sastry G N 2010 Int. J. Quantum. Chem. 110 1994
Nenitescu K D 1962 In Organicheskaya Khimiya (Organic Chemistry). Izdatestvo Innostrannoy Literatury, Academician Kabachnick, MI (ed.) (Moscow: Publishing of foreign literature) Vol. 1 p 815
Zhang R, Zhao G and Wu W 2009 Chin. J. Chem. Phys. 22 511
Lee K, Benson D R, and Kuczera K 2000 Biochemistry 39 13737
Gao J, Pavelites J J and Habibollazadeh D 1996 J. Phys. Chem. A. 100 2689
Vishnyakov A, Lyubartsev A P and Laaksonen A 2001 J. Phys. Chem. A. 105 1702
Finer E G, Franks F and Tait M J 1972 J. Am. Chem. Soc. 94 4424
Hoccart X and Turrel G J 1993 J. Chem. Phys. 99 8498
Keuleers R, Rousseau B, Alsenoy C V and Desseyn H O 1999 J. Phys. Chem. A. 103 462
Ramondo F, Bencienni L, Caminiti R, Pieretti A and Gontrani L 2007 Phys. Chem. Chem. Phys. 9 2206
Burton R C, Ferrari E S, Davey R J, Hopwood J, Qualey M J, Finney J L and Bowron D T 2008 Cryst. Growth Des. 8 1559
Siu D and Koga Y 2005 J. Phys. Chem. B. 109 16886
Lee M and van der Vegt N F A 2006 J. Am. Chem. Soc. 128 4948
Fong C, Wells D, Krodkiewska I, Hartley P G and Drummond C J 2006 Chem. Mater. 18 594
Koga Y, Miyazaki Y, Nagano Y and Inaba A 2008 J. Phys. Chem. B. 112 11341
Weiqn Z, Wen Y and Lihua Qiu 2005 J. Mol. Struct. (THEOCHEM) 730 133
Vazquez L, Salvarezza R C and Arvia A 1997 J. Phys. Rev. Lett. 79 709
Kim K, Lin Y T and Mosher H S 1988 Tetrahedron. Lett. 29 3183
Maryanoff C A, Stanzione R C, Plampin J N and Mills J E 1986 J. Org. Chem. 51 1882
Mantri P, Duffy D E and Kettner C A 1996 J. Org. Chem. 61 5690
Dempcy R O, Browne K A and Bruice T C 1995 J. Am. Chem. Soc. 117 6140
Sigman M S and Jacobsen E N 1998 J. Am. Chem. Soc. 120 4901
Whitesides G M and Ismagilov R F 1999 Science 284 89
Chigwada T R and Simoyi R H 2005 J. Phys. Chem. A. 109 1094
Gao Q Y, Liu B, Li L H and Wang J C 2007 J. Phys. Chem A. 111 872
Miller A E, Bischoff J J and Pae K 1988 Chem. Res. Toxicol. 1 169
Wiequn Z, Wen Y and L Qiu 2005 J. Mol. Struct. (THEOCHEM) 133
Peng K, Yang W and Zhou W 2009 Int. J. Quantum Chem. 109 811
Dill K A 1990 Biochemistry 29 7133
Jeffrey G A and Saenger W 1991 In Hydrogen Bonding in Biological Structures (Berlin: Springer-Verlag)
Stickle D F, Presta L G, Dill K A and Rose G D 1992 J. Mol. Biol. 226 1143
Mardyukov A, Sanchez-Garcia E, Rodziewicz P, Doltsinis N L and Sander W 2007 J. Phys. Chem. A. 111 10552
Frey J A and Leutwyler S 2006 J. Phys. Chem. A. 110 12512
Grabowski S J, Sokalski W A and Leszczynski J 2006 J. Phys. Chem. A. 110 4772
Papmokos G V and Demetropoulos I N 2004 J. Phys. Chem. A. 108 7291
Tsuchida E 2004 J. Chem. Phys 121 4740
Bende A and Suhai S 2005 Int. J. Quantum Chem. 103 841
Varga R, Garza J, Friesner R A, Stern H, Hay B P and Dixon D A 2001 J. Phys. Chem. A. 105 4963
Desfrancois C, Peiquet V, Carles S, Schermann J P and Andamowicz L 1998 Chem. Phys. 239 475
Cabaleiro-Lago E M and Otero J R 2002 J. Chem. Phys. 117 1621
Belosludov R V, Li Z and Kawazoe Y 1999 Mol. Eng. 8 105
Masunov A and Dannerberg J J 199 J. Phys. Chem. A. 103 178
Wallqvist A and Karlström G 1989 Chem. Scr. A. 29 1989
Tanaka H, Touhara H and Nakanishi K 1985 J. Chem. Phys. 82 5184
Jakli G and van Hook W W 1981 J. Phys. Chem. 85 3480
Adams R, Balyuzi H M and Burge R E 1977 J. Appl. Crystallogr. 10 256
Frisch M J, Trucks G W, Schlegel H B, Scuseria G E, Robb M A, Cheeseman J R, Scalmani G, Barone V, Mennucci B, Petersson G A, Nakatsuji H, Caricato M, Li X, Hratchian H P, Izmaylov A F, Bloino J, Zheng G, Sonnenberg J L, Hada M, Ehara M, Toyota K, Fukuda R, Hasegawa J, Ishida M, Nakajima T, Honda Y, Kitao O, Nakai H, Vreven T, Montgomery J A, Peralta J E, Ogliaro F, Bearpark M, Heyd J J, Brothers E, Kudin K N, Staroverov V N, Kobayashi R, Normand J, Raghavachari K, Rendel A, Burant J C, Iyengar S S, Tomasi J, Cossi M, Rega N, Millam J M, Klene M, Knox J E, Cross J B, Bakken V, Adamo C, Jaramillo C, Gomperts R, Stratmann R E, Yazyev O, Austin A J, Cammi R, Pomelli C, Ochterski J W, Martin R L, Morokuma K, Zakrzewski V G, Voth G A, Salvador P, Dannenberg J J, Dapprich S, Daniels A D, Farkas O, Foresman J B, Ortiz J V, Cioslowski J and Fox D J 2009 Exploring Chemistry with Electronic Structure Methods; Gaussian Inc.: Wallingford, CT
Hehre W J, Radoom L, Schleyer P V R and Pople J A 1986 In Ab Initio Molecular Orbital Theory (New York: Wiley)
Foresman J B and Frisch E 1996 In Exploring Chemistry with Electronic Structure Methods: A Guide to using Gaussian. (Pittsburg: Gaussian Inc.)
Boys S F and Bernardi F 1970 Mol. Phys. 19 553
Merrick J P, Moran D and Radom L 2007 J. Phys. Chem. A. 11683
Bader R F W 1990 In Atoms in Molecules: A Quantum Theory (Oxford: Oxford University Press)
Biegler-König F and Schönbohm J 2002 AIM 2000 version 2.0, Germany
Glendening E D and Streitwieser A 1994 J. Chem. Phys. 100 2900
Glendening E D 1996 J. Am. Chem. Soc. 118 2473
Schenter G K and Glendening E D 1996 J. Phys. Chem. 100 17152
Glendening E D, Badenhoop J K, Reed A E, Carpenter J E, Bohmann J A, Morales C M and Weinhold F 2001 Theoretical Chemistry Institute, University of Wisconsin, Madison
Weinhold F and Landis C R 2001 Chem. Edu. Res. Pract. 2 91
Schmidt M W, Baldridge K K, Boatz J, Elbert S T, Gordon M S, Jensen J H, Koseki S, Matsunaga N, Nguyen K A, Su S J, Windus T L, Dupuis M and Montgomery Jr. J A 1993 J. Comput. Chem. 141347
Foster J P and Weinhold F 1980 J. Am. Chem. Soc. 102 7211
Reed A E and Weinhold F 1983 J. Chem. Phys. 78 4066
Koch U and Popelier P L A 1995 J. Phys. Chem. 99 9747
Popelier P L A 2000 In Atoms in Molecules: An Introduction (London: Pearson Education)
Langley C H and Allinger N L 2003 J. Phys. Chem. A. 107 5208
Glendening E D 2005 J. Phys. Chem. A. 109 11936
Acknowledgement
The authors are highly thankful to University Grants Commission (UGC) for financial assistance.
Author information
Authors and Affiliations
Corresponding author
Additional information
Supplementary Information
The optimized geometrical parameters for the adducts and corresponding monomeric units using the B3LYP and MP2 method are accessible through the supporting information tables TS1–TS22. The optimized geometrical parameters for the dimers are reported in tables TS23–TS38. The values of topological properties at BCPs characterizing the hydrogen bonds in monohydrate adduct and homodimers are reported in tables TS39–S40. The atomic charges have been evaluated using NBO analysis for all the thirty-two monohydrated adducts and homodimers reported in tables TS41–TS45. Important second order stabilization energies E(2) (kcal/mol) for the orbital interactions strengthening the formation of adduct with water and their homodimers reported in TS46–TS47. Molecular electrostatic potential (MEP) maps of the molecules and their adducts with water under investigation along with the Vmax and Vmin values from blue to red regions respectively are reported in figure S1. Supplementary information is available at www.ias.ac.in/chemsci.
Electronic supplementary material
Below is the link to the electronic supplementary material.
Rights and permissions
About this article
Cite this article
KAUR, D., KHANNA, S. Hydrogen bonding of formamide, urea, urea monoxide and their thio-analogs with water and homodimers. J Chem Sci 126, 1815–1829 (2014). https://doi.org/10.1007/s12039-014-0725-6
Received:
Revised:
Accepted:
Published:
Issue Date:
DOI: https://doi.org/10.1007/s12039-014-0725-6