Abstract
In response to traumatic brain injury, there is local and transient accumulation of 2-AG at the site of injury, peaking at 4 h and sustained up to at least 24 h. Neuroprotection exerted by exogenous 2-AG suggests that the formation of 2-AG may serve as a molecular regulator of pathophysiological events, attenuating the brain damage. Inhibition of this protective effect by SR-141716A, a CB1 cannabinoid receptor antagonist, and the lack of effect of 2-AG in CB1 knockout mice suggest that 2-AG and the CB1 receptor may be important in the pathophysiology of traumatic brain injury. 2-AG exerts its neuroprotective effect after traumatic brain injury, at least in part, by inhibition of NF-κB transactivation. 2-AG also inhibits, at an early stage (2–4 h), the expression of the main proinflammatory cytokines, TNF-α, IL-6, and IL-1β, and is accompanied by reduction of BBB permeability. Moreover, the CB1, CB2, and TRVP1 receptors are expressed on microvascular endothelial cells, and their activation by 2-AG counteracts endothelin (ET-1)-induced cerebral microvascular responses (namely, Ca2+ mobilization and cytoskeleton rearrangement). This suggests that the functional interaction between 2-AG and ET-1 may provide a potential alternative pathway for abrogating ET-1-inducible vasoconstriction after brain injury and play a role in the neuroprotective effects exerted by 2-AG, as a potent vasodilator.
Similar content being viewed by others
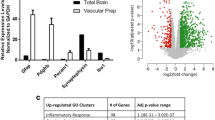
Avoid common mistakes on your manuscript.
Introduction
The mammalian body has a highly developed immune system, whose main role is to guard against protein attack and reduce the damage caused by it. A wide variety of immune responses occur to pathogen infections that use diverse strategies in their invasions. It is inconceivable that through evolution, analogous biological protective systems have not been developed against nonprotein attacks. Are there mechanisms through which our body lowers the damage caused by various types of trauma? The answer, of course, is affirmative, but details of the biological systems involved in neuroprotection are much less understood than those of the immune reactions.
We believe that the endocannabinoid system is a component of mammalian neuroprotective mechanisms. In this short overview, we shall review our work on the role of the endocannabinoid system on the sequelae of traumatic brain injury [1–3]. We assume that many of the results we have on trauma and endocannabinoids are relevant to other brain pathologies, such as cerebral ischemia or neurodegenerative disorders.
Traumatic brain injury is a major cause of mortality and morbidity in the young age (<40) group. Understanding posttrauma events is important as it may ultimately lead to the development of novel therapies. At present, there are no effective drugs to treat brain injury, although it is well established that the injury triggers both the accumulation of harmful mediators that lead to secondary damage and to the initiation of neuroprotective processes.
When we began our research in the field of brain trauma and cannabinoids, several groups had already shown that the emerging endocannabinoid system was involved in neuroprotection. It was known that N-acyl ethanolamines are formed in canine heart after ischemia, and about a decade later, it was reported that cannabinoid receptor agonists protect cultured rat hippocampal neurons from neurotoxicity, and several groups reported enhanced levels of anandamide post mortem or after injury [for reviews see 4–6]. Nagayama et al. [7] found that a synthetic cannabinoid agonist protected rat brain against ischemia and van der Stelt et al. [6] reported that the plant cannabinoid Δ9-tetrahydrocannabinol (THC) protects rat brain against oubain-induced excitotoxicity. Additional early work in this field is cited in [4–6].
Over the last decade, considerable progress has been made in understanding the role of endocannabinoids in preventing or reducing the effects of progressive neurodegenerative, diseases such as Parkinson’s disease, Huntington’s disease, multiple sclerosis, amyotropic lateral sclerosis, and Alzheimer’s disease, as well as ischemia and trauma; but, we still need a general theory encompassing the entire field.
Head Injury: Effects of 2-AG on Edema and Neurological Damage
We first looked into the changes of 2-arachidonoyl glycerol (2-AG) levels in mouse brain after closed head injury (CHI) [1]. We had previously reported that 2-AG suppresses the formation of reactive oxygen species (ROS) and TNF-α by murine macrophages in vitro after stimulation with lipopolysaccharide (LPS). Lowering of TNF-α levels was also seen in serum of LPS-treated mice on administration of 2-AG [8]. We assumed that if 2-AG levels were enhanced after CHI, we would note attenuation of brain damage, when it was administered as a drug, as both TNF- α and ROS contribute to the pathophysiology of brain trauma [1]. Mice were subjected to CHI using a weight-drop device or to sham surgery. They were decapitated at various time intervals (between 15 min and 24 h), and the brains were immediately frozen in liquid nitrogen to prevent post mortem formation of 2-AG. The levels of 2-AG were determined in the traumatized hemispheres and compared with those in controls. The levels of 2-AG increased after brain trauma, reaching a tenfold peak after 4 h, declining thereafter. After 24 h, the levels were still sixfold higher than in the controls [1].
Were the enhanced 2-AG levels just a result of some biochemical process gone astray due to the trauma, or were they a neuroprotective response? To test this, we synthesized and administered 2-AG at various doses, 15 min before or 1 h after CHI. We first evaluated the functional status of the mice, 1 and 24 h after the trauma using a scoring system, named neurological severity score (NSS), based on motor and behavioral functions. The difference between the score after 1 h and after 24 h after injury (ΔNSS) indicates the extent of recovery, with the higher number demonstrating a greater improvement. We found that 5 mg/kg 2-AG led to a significant functional recovery. Moreover, it also reduced edema formation (accumulation of water in the tissue), which reaches its maximal level 24 h after injury [1]. In our experimental paradigm, the water content in the untreated mice is about 81%, and in noninjured controls, it is about 78%. 2-AG doses as low as 0.1 mg/kg led to a significant reduction in water content (about 50%). Surprisingly, higher doses did not give better results. The NSS after 1 h was similar in the treated and control groups. However, the mice treated with 2-AG recovered faster within the first 24 h. All doses of 2-AG showed a trend towards better recovery (greater NSS), but it reached significance only at 5 mg kg−1. We found that 2-AG is less potent against the motor deficits than in reducing edema, as evidenced from the different effective doses needed to achieve protection. As brain edema and neurological deficits represent different manifestations of brain damage, this observation is not surprising [1].
Neuronal cell death is one of the manifestations of brain injury and is mainly evidenced by an infarct around the site of injury. Mice treated with 2-AG (5 mg kg−1) showed significantly reduced infarct volume compared with that in vehicle-treated mice, while no infarct was observed in sham-operated mice. The effect of 2-AG on hippocampal cell death, which occurs specifically in the CA3 subfield was also examined. The ipsilateral hippocampus of vehicle- and 2-AG-treated mice showed ischemic and dystrophic neurons, with large or small patchy acellular regions, in the CA3 subfield. No neuronal pathology could be detected in the contralateral CA3 region, while neuronal loss was apparent in the injured side compared with the contralateral side. This loss was significantly larger in the vehicle-treated animals. The number of viable neurons counted in the CA3 area in the injured hemisphere was significantly lower in the vehicle-treated mice than in the 2-AG-treated mice [1].
The protective effect of 2-AG is mediated, at least in part, by the CB1 cannabinoid receptor. Administration of SR-141716A (20 mg kg−1), an antagonist specific to the CB1 receptor, together with 2-AG after CHI led to a dose dependent worsening of the ΔNSS and the brain water content compared to mice that had received 2-AG alone. Mice treated with 2-AG and antagonist did not differ from the CHI control mice. The doses of the antagonist used were relatively high, but the antagonist alone did not worsen the outcome of traumatized mice not treated with 2-AG.
To confirm endocannabinoid involvement in brain trauma damage, the spontaneous and 2-AG-mediated neurobehavioral recovery in CB1 receptor knockout (CB1−/−) mice were compared with those observed in wild-type (WT) mice [2]. Zimmer et al. have reported that naive \( {\text{CB}}^{{ - \mathord{\left/ {\vphantom { - - }} \right. \kern-\nulldelimiterspace} - }}_{1} \) mice display some motor deficits, unrelated to the trauma [9]. As expected, after brain injury, the spontaneous recovery of \( {\text{CB}}^{{ - \mathord{\left/ {\vphantom { - - }} \right. \kern-\nulldelimiterspace} - }}_{1} \) mice was extremely slow compared with the WT mice, suggesting that the endogenous cannabinoids play a role in the spontaneous recovery after CHI. In another experiment, NSS was evaluated at 3 and 7 days, and the same pattern was found, namely, NSS of the CB1−/− mice remained below 1 during the whole period, while that of the WT slowly, but consistently, increased. These findings agree with those of Parmentier-Batteur et al., who showed increased severity of stroke in \( {\text{CB}}^{{ - \mathord{\left/ {\vphantom { - - }} \right. \kern-\nulldelimiterspace} - }}_{1} \) mice [10]. To further confirm the role of the CB1 receptor in 2-AG neuroprotection, WT and \( {\text{CB}}^{{ - \mathord{\left/ {\vphantom { - - }} \right. \kern-\nulldelimiterspace} - }}_{1} \) mice were treated with exogenous 2-AG. As expected, no beneficial effects, either on neurobehavior or on edema formation, were noted in the \( {\text{CB}}^{{ - \mathord{\left/ {\vphantom { - - }} \right. \kern-\nulldelimiterspace} - }}_{1} \) mice, in contrast to a significant effect on the WT.
It seems unreasonable to assume that the endocannabinoid system alone mediates the neuroprotective reaction of the injured brain. Accordingly, additional endogenous systems should be evaluated. Likewise, additional non-CB1-mediated actions of 2-AG cannot be excluded [1].
Endogenous active compounds are frequently accompanied by ‘inactive’ congeners. This is true also for the endocannabinoids [11]. 2-AG is accompanied in mouse brains by an ‘entourage’ of congeners, such as 2-palmitoyl-glycerol and 2-linoleoyl-glycerol, which alone have no activity at the cannabinoid receptors. However, these glycerol esters facilitate the binding of 2-AG to the CB1 and CB2 receptors, probably through increase in the availability of 2-AG at the receptors by inhibiting its hydrolysis and uptake. To test the effect of the ‘entourage’ after CHI, mice were injected with a combination of 2-AG (1 mg kg−1), 2-palmitoyl-glycerol (5 mg kg−1), and 2-linoleoyl-glycerol (10 mg kg−1) 1 h after CHI. Although no effect was observed when these compounds were injected separately, the combination of the three acyl-glycerol esters led to a significant improvement in functional recovery at 24 h. When the combination was administered again 24 and 48 h after CHI, the improved clinical recovery was sustained up to 7 days after the trauma [1]. These results may be of general importance. Active compounds, either endogenous constituents or synthetic drugs, are usually administered alone. Is it conceivable that in some cases, in particular, with endogenous constituents, adding specific ‘inactive’ compounds, which enhance or modify their activity, may be of therapeutic importance.
These results were the first to demonstrate temporal and local changes in brain levels of the endogenous cannabinoid 2-AG, after traumatic brain injury and to record its neuroprotective effects. We had previously reported using the same model of CHI that the arachidonic acid metabolic cascade is activated after trauma [12]. This event may be related to 2-AG release, as 2-AG can be synthesized and released from neuronal and non-neuronal cells on demand by a non-synaptic release mechanism. Endocannabinoids are taken up by cells by either using a specific transporter or by passive diffusion and are hydrolyzed by fatty acid amide hydrolase (FAAH), which acts on anandamide and an esterase which acts on 2-AG [13]. It seems reasonable to expect that drugs blocking the hydrolysis of 2-AG may be of considerable therapeutic value.
Several cannabinoids that bind to CB1 have been assayed for neuroprotection both in vitro and in vivo, mostly using ischemia and excitotoxicity models. These publications have been reviewed extensively [4–6, 14, 15]. We would like to point out however that while brain damage due to ischemia or excitotoxicity is related to the damage observed on brain trauma, they are not identical. Thus, Leker et al. [16] have shown that the highly potent CB1 agonist HU-210 reduces ischemic damage by inducing hypothermia. In contrast, no reduction of body temperature was found when 2-AG was administered after CHI (0.1 mg kg−1, a dose that abolished edema). Only minor reduction (1.6–1.9°C) of short duration (<2 h), which is presumably too small to exert the observed protection, was noted after administration of 5 mg kg−1 AG [1]. However, such minor hypothermia may possibly contribute to the observed neuroprotection.
What are the molecular mechanisms involved in neuroprotection after brain trauma? We assume that one possible mechanism by which 2-AG may protect the injured brain is related to its ability to inhibit glutamate release [1]. Acting as a retrograde activator of presynaptic CB1 receptors, which are Gi-coupled, such a mechanism could well be involved in neuroprotection.
In addition, via its effect on cerebral vasculature, 2-AG was also shown to abolish the deleterious effect of endothelin-1 (ET-1) [17]. ET-1 is the most potent vasoconstrictor known. It reduces cerebral blood flow and is important in the pathophysiology of brain trauma and ischemia. Indeed, we have demonstrated that 2-AG counteracts ET-1-induced cerebral capillary and microvascular endothelial responses (Ca2+ mobilization and cytoskeletal rearrangement) [18]. 2-AG may thus affect brain damage by lowering cerebral vasoconstriction. It should be pointed out that endocannabinoid-like brain constituents, which are not CB1 agonists, such as arachidonoyl serine, have also been found to cause vasodilation [19]. Their relevance to neuroprotection has not yet been reported.
Head Injury: Effects of 2-AG on Inflammatory Pathways and Oxidation
Cannabinoids have been found to be very effective in different models of inflammation. Already in the 1970s, Sofia et al. [20] showed robust anti-inflammatory effects of crude marijuana extract, of the active marijuana constituent THC, and of the marijuana nonpsychoactive constituents CBD and cannabinol (CBN), in paw edema inflammation model in rats. Some of these effects were later shown to be CB1 or CB2 receptor mediated [21]. Both cannabinol and 2-AG were shown to inhibit IL-2 expression in activated thymocytes through inhibition of NF-κB [22]. The detailed molecular mechanisms of all these beneficial effects remain unclear.
Inflammatory response is one of the early neurochemical events after CHI, and the upregulation of TNF-α and IL1β within the first few hours after injury is known to be deleterious. Treatment with 2-AG after CHI indeed significantly abolished this response [3]. As TNF-α is associated with the activation of nuclear factor-κB (NF-κB), a key regulator of inflammatory response (see below), and in view of the effect of 2-AG on NF-κB in thymocytes [22], we looked into the possibility that transcription factor is affected by cannabinoids in brain trauma [2].
NF-κB is composed of homo- and heterodimers including p65 and p50. The p65 subunit contains a translocation domain in its C-terminal end. Inactive NF-κB is retained in the cytosol, where its activity is tightly regulated by members of the IκB family. Activation of the IκB kinase by different proinflammatory signals (e.g., endotoxin, TNF-α, IL-1β, oxidative stress) leads to phosphorylation, ubiquitination, and degradation of IκB. NF-κB, thus released, translocates into the nucleus and activates various genes [23, 24]. CHI causes robust transactivation of NF-κB. Inhibition of this activation by melatonin is indeed known to be associated with improved outcome [25].
As mentioned above, proinflammatory cytokines play a crucial role in traumatic brain injury. TNF-α is released early (within 1 to 4 h) after CHI [26], and acts on specific receptors. On binding, the cytosolic portions of the TNF receptors recruit multiple intracellular adapter proteins that activate NF-κB, starting with hydrolysis of the inhibitory protein IκB that allows the p65/p50 complex to translocate to the nucleus and to regulate the expression of various proinflammatory cytokines [23, 24].
We found, as expected, that treatment of mice after CHI with 2-AG completely abolished the robust activation of NF-κB [2]. We also explored the effect of 2-AG on NF-κB transactivation after CHI in \( {\text{CB}}^{{ - \mathord{\left/ {\vphantom { - - }} \right. \kern-\nulldelimiterspace} - }}_{1} \) mice [2]. First, we noted that CHI-induced activation of NF-κB in the \( {\text{CB}}^{{ - \mathord{\left/ {\vphantom { - - }} \right. \kern-\nulldelimiterspace} - }}_{1} \) mice was three- to fourfold higher than in the respective noninjured (sham) \( {\text{CB}}^{{ - \mathord{\left/ {\vphantom { - - }} \right. \kern-\nulldelimiterspace} - }}_{1} \) mice, similar to that observed in the WT. This suggests that endogenous 2-AG does not affect the inflammatory signaling. Then, the spontaneous and 2-AG-mediated activation of NF-κB in these mice were compared with that observed in wild-type (WT) mice. WT and \( {\text{CB}}^{{ - \mathord{\left/ {\vphantom { - - }} \right. \kern-\nulldelimiterspace} - }}_{1} \) mice differed dramatically, as the latter did not respond at all to 2-AG treatment, while as mentioned above, 2-AG completely abolished the robust activation of NF-κB in the WT [2]. Thus, the endocannabinoids join the large group of compounds that inhibit NF-κB at one or multiple activation steps of the signaling pathway. These agents include proteasome inhibitors, glucocorticoids (such as dexamethasone), nonsteroidal anti-inflammatory drugs, and anti-inflammatory cytokines [27].
In addition to their anti-inflammatory activities, cannabinoids act also as antioxidants. The cannabis component, cannabidiol (CBD), is a potent antioxidative agent [28], and the nonpsychotropic synthetic cannabinoid, dexanabinol (HU-211), has also antioxidative properties [29]. As mentioned above, 2-AG was shown to suppress formation of ROS by murine macrophages in vitro after stimulation with lipopolysaccharide [8], and McCarron et al. [30] have reported on the antioxidant properties of 2-AG.
Oxidative stress is one of the major components in the pathophysiology of traumatic brain injury [31], and ample evidence suggests that ROS also regulate signal transduction pathways such as the NF-κB [32]. Thus, taken together, the anti-inflammatory and antioxidant properties of 2-AG may either add or synergize to enhance its activity as a neuroprotective agent. The effects of 2-AG and other antioxidants found in the brain on the blood–brain barrier are discussed in the following section.
Head Injury: Effect of 2-AG on the Blood–Brain Barrier
One of the early harmful events of TBI is the disruption of the blood–brain barrier (BBB), allowing infiltration of peripheral macrophages and leukocytes into cerebral tissue and aggravation of the inflammatory response. Reactive microglia produce proinflammatory cytokines such as TNF-α and IL-6, which in turn, together with NO, contribute towards the impairment of the BBB function [33].
TNF-α-converting enzyme (TACE) is a membrane protein that belongs to the ADAM (a disintegrin and a metalloprotease) family. It cleaves various membrane proteins, including the transmembrane precursor form of TNF-α. Hence, indirectly, it contributes to the impairment of the BBB. Little is known about the physiological role of TACE; however, some neurotoxic and neuroprotective functions have been reported [34].
Matrix metalloproteinases (MMPs) play a major role in physiological extracellular matrix turnover in pathological processes. Increases in MMP activity have been reported in brain disorders such as multiple sclerosis, Alzheimer’s disease, and after head trauma [35]. Downregulation of certain metalloproteinases may exert neuroprotection [36]. Matrix metalloproteinase-9 (MMP-9) participates in the disruption of the BBB during hemorrhagic transformation and exacerbates brain injury after cerebral ischemia.
Reactive oxygen species (ROS) have also been shown to play a role in altering BBB permeability and formation of brain edema induced by trauma. Antioxidants (e.g., nitroxides) have been reported to protect the BBB [37], and the findings of McCarron et al. [30] provide evidence of 2-AG antioxidant activity, and thus, to its possible effects on the BBB.
CHI has been shown to cause major transient disruption of BBB integrity, which peaks at 4 h post-injury [3]. Therefore, a study was designed to investigate the effects of 2-AG on BBB integrity after CHI and on the expression profile of the proinflammatory cytokines and the enzymes TACE and matrix metalloproteinase (MMP-9) over the initial 2–4 h after the injury, the time preceding the maximal disruption of the BBB [3]. 2-AG was administered immediately after CHI, and the BBB permeability 4 h after the trauma was determined. In the left, injured hemisphere 2-AG robustly reduced BBB disruption, as evidenced by 40% decrease in dye levels extracted from the brain. In the right hemisphere, not affected by CHI, no changes in dye levels were noted. The significant reduction of the BBB permeability after treatment with 2-AG may explain its effect on edema, seen at 24 h [1, 2], and on functional recovery. These findings also suggest that the mechanism by which 2-AG exerts its effect on the BBB may involve inhibition of the early (<4 h) inflammatory response.
The examination of the levels of the enzyme TACE after CHI showed that while TACE expression increased between 2 and 4 h after CHI, 2-AG had no effect on its levels. MMP-9 levels were similar at 2 and 4 h, and no effect of 2-AG on this enzyme was noted. Unexpectedly, these results indicate that the inhibition of cytokine expression and BBB protection are not associated with inhibition of these proteolytic enzymes [3].
In view of the effects of some cytokines on brain injury and the BBB (see above), the gene expression of proinflammatory cytokines was examined by using real-time-polymerase chain reaction (RT-PCR), and their amount was expressed relative to β-actin [3]. TNF-α mRNA was not found in noninjured sham mice, while a robust increase was found 2 and 4 h after injury. 2-AG significantly inhibits TNF-α expression at 2, but not at 4 h injury. The effect of 2-AG on TNF-α expression was confirmed and quantified by quantitative RT-PCR, showing an inhibitory effect of 2-AG on this cytokine 2 h post-CHI, but not at 4 h after injury.
Again, using RT-PCR, it was found that IL-6 mRNA levels after injury increased between 2 and 4 h. 2-AG treatment led to a decrease in IL-6 levels at 4 h, but not at 2 h. These observations underline again the complexity and time dependence of the changes taking place after CHI.
Three classes of water-soluble antioxidants are found in the brain, having oxidation potentials of 330–360 mV (e.g., ascorbic acid, uric acid), 650–700 mV (e.g., carnosine, melatonin, NADH) and 1,050–1,100 mV (e.g., lipoic acid) [38]. It was found that 4 h after CHI, the class of weak antioxidants, having the highest oxidation potential (1,050–1,100 mV), is significantly enhanced after CHI. 2-AG further significantly increases the levels of these compounds, thus, improving the tissue’s reducing capacity. The augmentation of these levels, which is noted at 4 h after CHI, may also contribute to the neuroprotection exerted by 2-AG. Indeed, previous work has shown that high levels of ROS-neutralizing molecules allow better coping in tissues with oxidative stress and enhancement of BBB protection [39]. We have proposed that ROS and TNF-α act synergistically at the early post CHI period, leading, among other effects, to BBB disruption [40]. Thus, taken together, inhibition of cytokines, along with higher levels of low molecular weight anti-oxidants (LMWA), protects the BBB from the early effects of CHI.
Head Injury: Some Random Thoughts
We have presented evidence that the endocannabinoid system lowers brain damage through a variety of mechanisms. But the picture is far from complete. Cannabinoids produce a variety of neurobehavioral effects, and a major focus of cannabinoid research has been the substantiation of the assumption that the pharmacological actions of cannabinoids are receptor-mediated. Our current findings fit into this body of evidence regarding the importance of the endocannabinoid system, acting via the CB1 receptor, in brain protection under physiological and pathological conditions. Several other groups, likewise, have discussed the role of the endocannabinoid system as a general endogenous protection system [4–6].
However, the pharmacological picture is complicated by numerous factors. For example, there seems to be species differences. While we found 2-AG production to be enhanced in adult mice after CHI [1], Hansen et al. found anandamide, and not 2-AG enhancement, in rat pups brains after trauma [41]. But, in view of the different procedures in these trauma experiments, it is at present difficult to state with certainty that species differences actually exist.
While we found that the 2-AG effects on brain trauma are CB1 dependent, Sancho et al. have reported that anandamide inhibits nuclear factor-kappaB activation through a cannabinoid receptor-independent pathway [42]. Again, the experimental conditions were completely different. Numerous other cannabinoid reactions are CB1/CB2 receptor independent (see for example, [43]). Are we missing something?
Until recently, it was assumed that CB2 receptors are not present in the brain. Over the last few years, numerous groups have shown that these receptors exist in the brain (mostly in glial cells), and their formation is enhanced after neurological diseases [44, 45]. Are CB2 receptors and agonists involved in protection against brain trauma? Is it possible that CB1 agonists are involved in the initial stages of brain trauma and CB2 agonists only later?
As mentioned above, some cytokines are involved in the damage produced by brain trauma. Molina-Holdago et al. [46] have shown that an endogenous IL-1 receptor antagonist mediates anti-inflammatory and neuroprotective actions of cannabinoids in neurons and glia. Are such effects relevant to the effects described above?
Brain ischemia and numerous neurological diseases are affected by cannabinoids (for reviews see [47–49]). Are the above-described results therapeutically relevant to such conditions? We believe that they are indeed relevant, but experimental clinical proof is still in the far away horizon. To the best of our knowledge, neither anandamide nor 2-AG have ever been administered to humans. This is in sharp contrast to insulin or the corticoids, for example, which were administered to patients shortly after their discovery in the 1920s and 1930s and became major therapeutic drugs. Again, are we missing something?
Conclusion
In conclusion, our studies shed additional light on the neuroprotective effects of the endocannabinoid 2-AG and on the mechanisms by which it exerts these effects, within the early period after traumatic brain injury. In view of the complexity of brain anatomy and physiology, it is inconceivable that 2-AG and the endocannabinoid system are the only protective tools available to the mammalian body to serve as protective agents. Additional, already known or unknown, systems are certainly involved. However, our knowledge in this area is limited.
The timing of the initiation of protective processes is presumably an important factor too. As mentioned above, CB2 receptors are known to be produced in the brain in numerous neurological diseases [44, 45]. Their role is presumably protective. Their formation indicates that while CB1 receptor-mediated processes are possibly mainly involved in the initial stages of protection, CB2 receptor-mediated processes are initiated at later stages.
We believe that brain trauma research aimed at the discovery of new protective compounds or novel processes and their timing and interactions may lead to important new findings of both basic and therapeutic value.
References
Panikashvili D, Simeonidou C, Ben-Shabat S, Hanus L, Breuer A, Mechoulam R, Shohami E (2001) An endogenous cannabinoid (2-AG) is neuroprotective after brain injury. Nature 413:527–531
Panikashvili D, Mechoulam R, Beni SM, Alexandrovitch A, Shohami E (2005) CB1 cannabinoid receptors are involved in neuroprotection via NF-kB inhibition. J Cerebral Blood Flow Metabol 25:477–484
Panikashvili D, Shein NA, Mechoulam R, Trembovler V, Kohen R, Alexandrovitch A, Shohami E (2006) The endocannabinoid 2-AG protects the blood brain barrier after closed head injury and inhibits mRNA expression of proinflammatory cytokines. Neurobiol Disease 22:257–264
Mechoulam R, Panikashvili D, Shohami E (2002) Cannabinoids and brain injury: therapeutic implications. Trends Mol Med 8:58–61
Hansen HS, Moesgaard B, Petersen G, Hansen HH (2002) Putative neuroprotective actions of N-acyl-ethanolamines. Pharmacol Therap 95:119–126
van der Stelt M, Veldhuis WB, Maccarrone M, Bar PR, Nicolay K, Veldink GA, Di Marzo V, Vliegenthart JF (2002) Acute neuronal injury, excitotoxicity, and the endocannabinoid system. Mol Neurobiol 26:317–346
Nagayama T, Sinor AD, Simon RP, Chen J, Graham SH, Jin KL, Greenberg DA (1999) Cannabinoids and neuroprotection in global and focal cerebral ischemia and in neuronal cultures. J Neurosci 19:2987–2995
Gallily R, Breuer A, Mechoulam R (2000) 2-Arachidonylglycerol, an endogenous cannabinoid, inhibits tumor necrosis factor-production in murine macrophages, and in mice. Eur J Pharmacol 406:R5–R7
Zimmer A, Zimmer AM, Hohmann AG, Herkenham M, Bonner TI (1999) Increased mortality, hypoactivity and hypoalgesia in cannabinoid CB1 receptor knockout mice. Proc Natl Acad Sci USA 96:5780–5785
Parmentier-Batteur S, Jin K, Mao Xo Xie L, Greenberg DA (2002) Increased severity of stroke in CB1 cannabinoid receptor knock-out mice. J Neurosci 22:9771–9775
Ben-Shabat S, Fride E, Sheskin T, Tamiri T, Rhee MH, Vogel Z, Bisogno T, De Petrocellis L, Di Marzo V, Mechoulam R (1998) An entourage effect: inactive endogenous fatty acid glycerol esters enhance 2-arachidonoyl–glycerol cannabinoid activity. Eur J Pharmacol 353:23–31
Shohami E, Shapira Y, Yadid G, Reisfeld N, Yedgar S (1989) Brain phospholipase A2 is activated after experimental closed head injury in rats. J Neurochem 53:1541–1546
Di Marzo V, Matias I (2006) Endocannabinoid synthesis and degradation, and their regulation in the framework of energy balance. J Endocrinol Invest 29(3 Suppl):15–26
Marsicano G, Lutz B (2006) Neuromodulatory functions of the endocannabinoid system. J Endocrinol Invest 29:27–46
Fernández-Ruiz J, González S, Romero J, Ramos JA (2005) Cannabinoids in neurodegeneration and neuroprotection. In Mechoulam R (ed) Cannabinoids as Therapeutics. Birkhaüser Verlag, Switzerland, pp 79–109
Leker RR, Gai N, Mechoulam R, Ovadia H (2003) Drug-induced hypothermia reduces ischemic damage. Effect the cannabinoid HU-210. Stroke 34:2000–2006
Chen Y, McCarron RM, Ohara Y, Bembry J, Azzam N, Lenz FA, Shohami E, Mechoulam R, Spatz M (2000) Human brain capillary endothelium: 2-arachidonoglycerol (endocannabinoid) interacts with endothelin-1. Circ Res 87:323–327
McCarron RM, Chen Y, Tomori T, Strasser A, Mechoulam R, Shohami E, Spatz M (2006) Endothelial-mediated regulation of cerebral microcirculation. J Physiol Pharmacol 57(suppl 11):133–144
Milman G, Maor Y, Abu-Lafi S, Horowitz M, Gallily R, Batkai S, Mo FM, Offertaler L, Pacher P, Kunos G, Mechoulam R (2006) N-Arachidonoyl l-serine, a novel endocannabinoid-like brain constituent with vasodilatory properties. Proc Natl Acad Sci USA 103:2428–2433
Sofia RD, Nalepa SD, Vassar HB, Knobloch LC (1974) Comparative anti-phlogistic activity of delta 9-tetrahydrocannabinol, hydrocortisone and aspirin in various rat paw edema models. Life Sci 15:251–260
Hanus L, Breuer A, Tchilibon S, Shiloah S, Goldenberg D, Horowitz M, Pertwee RG, Ross RA, Mechoulam R, Fride E (1999) HU-308: a specific agonist for CB(2), a peripheral cannabinoid receptor. Proc Natl Acad Sci USA 96:14228−14233
Herring AC, Kaminski NE (1999) Cannabinol-mediated inhibition of nuclear factor-kappaB, cAMP response element-binding protein, and interleukin-2 secretion by activated thymocytes. J Pharmacol Exp Ther 291:1156–1163
Karin M, Ben-Neriah Y (2000) Phosphorylation meets ubiquitination: the control of NF-[kappa]B activity. Annu Rev Immunol 18:621−663
Malek S, Chen Y, Huxford T, Ghosh G (2001) IκBβ but not IκBα functions as a classical cytoplasmic inhibitor of NF-κB dimers by masking both NF-κB nuclear localization sequences in resting cells. J Biol Chem 276:45225–45235
Beni-Adani L, Gozes I, Cohen Y, Assaf Y, Steingart RA, Brenneman DE, Eizenberg O, Trembolver V, Shohami E (2001) Reduced mortality and improved recovery after treatment with a femtomolar-acting peptide in a mouse model of closed head injury. J Pharmacol Exp Ther 296:57–63
Shohami E, Gallily R, Mechoulam R, Bass R, Ben-Hur T (1997) Cytokine production in the brain following closed head injury: dexanabinol (HU-211) is a novel TNF inhibitor and an effective neuroprotectant. J Neuroimmunol 72:169–177
Zingarelli B, Sheehan M, Wong HR (2003) Nuclear factor-κB as a therapeutic target in critical care medicine. Crit Care Med 31:S105–S111
Hampson AJ, Grimaldi M, Axelrod J, Wink D (1998) Cannabidiol and (−)Δ9-tetrahydrocannabinol are neuroprotective antioxidants. Proc Natl Acad Sci USA 95:8268–8273
Shohami E, Mechoulam R (2000) Dexanabinol (HU-211): a nonpsychotropic cannabinoid with neuroprotective properties. Drug Dev Res 50:211–215
McCarron RM, Shohami E, Panikashvili D, Chen Y, Golech S, Strasser A, Mechoulam R, Spatz M (2003) Antioxidant properties of the vasoactive endocannabinoid, 2-arachidonoyl glycerol (2-AG). Acta Neurochir Suppl 86:271–275
Lewen A, Matz P, Chan PH (2000) Free radical pathways in CNS injury. J Neurotrauma 17:871–890
Vollgraf U, Wegner M, Richter-Landsberg C (1999) Activation of AP-1 and nuclear factor-kappa B transcription factors is involved in hydrogen peroxide-induced apoptotic cell death of oligodendrocytes. J Neurochem 73:2501–2509
Walter L, Stella N (2004) Cannabinoids and neuroinflammation. Br J Pharmacol 141:775–785
Moro MA, Hurtado O, Cardenas A, Romera C, Madrigal JL, Fernandez-Tome P, Leza JC, Lorenzo P, Lizasoain I (2003) Expression and function of tumour necrosis factor-alpha-converting enzyme in the central nervous system. Neurosignals 12:53–58
von Gertten C, Holmin S, Mathiesen T, Nordqvist AC (2003) Increases in matrix metalloproteinase-9 and tissue inhibitor of matrix metalloproteinase-1 mRNA after cerebral contusion and depolarization. J Neurosci Res 73:803–810
Mori T, Wang X, Aoki T, Lo EH (2002) Downregulation of matrix metalloproteinase-9 and attenuation of edema via inhibition of ERK mitogen activated protein kinase in traumatic brain injury. J Neurotrauma 19:1411–1419
Beit-Yannai E, Zhang R, Trembovler V, Samuni A, Shohami E (1996) Cerebroprotective effect of stable nitroxide radicals in closed head injury in the rat. Brain Res 717:22–28
Shohami E, Beit-Yannai E, Horowitz M, Kohen R (1997) Oxidative stress in closed-head injury: brain antioxidant capacity as an indicator of functional outcome. J Cereb Blood Flow Metab 17:1007–1019
Beit-Yannai E, Kohen R, Horowitz M, Trembovler V, Shohami E (1997) Changes in biological reducing activity in rat brain following closed head injury: a cyclic voltammetry study in normal and acclimated rats. J Cereb Blood Flow Metab 17:273–279
Trembovler V, Beit-Yannai E, Younis F, Gallily R, Horowitz M, Shohami E (1999) Antioxidants attenuate acute toxicity of tumor necrosis factor-alpha induced by brain injury in rat. J Interferon Cytokine Res 19:791–795
Hansen HH, Schmid PC, Bittigau P, Lastres-Becker I, Berrendero F, Manzanares J, Ikonomidou C, Schmid HH, Fernandez-Ruiz JJ, Hansen HS (2001) Anandamide, but not 2-arachidonoylglycerol, accumulates during in vivo neurodegeneration. J Neurochem 78:1415–1427
Sancho R, Calzado MA, Di Marzo V, Appendino G, Munoz E (2003) Anandamide inhibits nuclear factor-kappaB activation through a cannabinoid receptor-independent pathway. Mol Pharmacol 63:429–438
Chen Y, Buck J (2000) Cannabinoids protect cells from oxidative cell death: a receptor-independent mechanism. J Pharmacol Exp Ther 293:807–812
Fernández-Ruiz J, Romero J, Velasco G, Tolón RM, Ramos JA, Guzmán M (2007) Cannabinoid CB2 receptor: a new target for controlling neural cell survival? Trends Pharmacol Sci 28:39–45
Ashton JC, Glass M (2007) The cannabinoid CB2 receptor as a target for inflammation-dependent neurodegeneration. Curr Neuropharmacol 5:73–80
Molina-Holgado F, Pinteaux E, Moore JD, Molina-Holgado E, Guaza C, Gibson RM, Rothwell NJ (2003) Endogenous interleukin-1 receptor antagonist mediates anti-inflammatory and neuroprotective actions of cannabinoids in neurons and glia. J Neurosci 23:6470–6474
Pacher P, Batkai S, Kunos G (2006) The endocannabinoid system as an emerging target of pharmacotherapy. Pharmacol Rev 58:389–462
Mackie K (2006) Cannabinoid receptors as therapeutic targets. Annu Rev Pharmacol Toxicol 46:101–122
Piomelli D (2005) The endocannabinoid system: a drug discovery perspective. Curr Opin Investig Drugs 6:622–679
Acknowledgements
We thank the US National Institute on Drug Abuse, the US-Israel Binational Foundation, and the Miriam and Sheldon Adelson Program in Neural Repair and Rehabilitation for generous support.
Author information
Authors and Affiliations
Corresponding author
Rights and permissions
About this article
Cite this article
Mechoulam, R., Shohami, E. Endocannabinoids and Traumatic Brain Injury. Mol Neurobiol 36, 68–74 (2007). https://doi.org/10.1007/s12035-007-8008-6
Received:
Accepted:
Published:
Issue Date:
DOI: https://doi.org/10.1007/s12035-007-8008-6