Abstract
Lead (Pb)-induced reproductive toxicity is a well-characterized adverse effect associated with this heavy metal. It has been found that Pb exposure is associated with altered spermatogenesis, increased testicular degeneration, and pathological sperm alterations. On the other hand, it has been reported that Pb-induced reproductive toxicity is associated with increased reactive oxygen species (ROS) formation and diminished antioxidant capacity in the reproductive system. Hence, administration of antioxidants as protective agents might be of value against Pb-induced reproductive toxicity. This study was designed to investigate whether carnosine (CAR) and histidine (HIS) supplementation would mitigate the Pb-induced reproductive toxicity in male rats. Animals received Pb (20 mg/kg/day, oral, 14 consecutive days) alone or in combination with CAR (250 and 500 mg/kg/day, oral, 14 consecutive days) or HIS (250 and 500 mg/kg/day, oral, 14 consecutive days). Pb toxicity was evident in the reproductive system by a significant increase in tissue markers of oxidative stress along with severe histopathological changes, seminal tubule damage, tubular desquamation, low spermatogenesis index, poor sperm parameters, and impaired sperm mitochondrial function. It was found that CAR and HIS supplementation blunted the Pb-induced oxidative stress and mitochondrial dysfunction in the rat reproductive system. Thereby, antioxidative and mitochondria-protective properties serve as primary mechanisms for CAR and HIS against Pb-induced reproductive toxicity.
Similar content being viewed by others
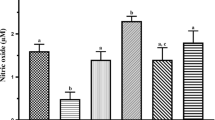
Avoid common mistakes on your manuscript.
Introduction
There has been serious concern about the deterioration of human and animal male reproductive health in association with exposure to environmental, industrial, and occupational chemicals, as well as several pharmaceuticals [1,2,3]. Among an array of toxic chemicals, heavy metals are environmental toxicants which pose male reproductive toxicity and infertility in wildlife, experimental animals, and humans [1, 2]. Lead (Pb) is a toxic pollutant extensively distributed in the environmental and biological systems. As a result of industrial development, the environmental levels of Pb have raised more than 1000 times, over the past 30 years [4]. It has been reported that Pb exposure, even at very low doses, is implicated in the impairment of a wide range of body function in laboratory animals and humans [5, 6]. Several systems including the heart, kidneys, liver, central and peripheral nervous systems, endocrine system, reproductive system, and hematological attributes are affected by Pb exposure [7,8,9,10,11,12,13,14].
Previous investigations have shown that Pb exposure induces deleterious effects on sperm motility, caused premature acrosome reaction, reduced zona-intact oocyte penetrating capability, decreased plasma testosterone, and impaired spermatogenesis [15,16,17,18,19]. The role of oxidative stress and its associated complications have been well described in Pb-induced reproductive toxicity [15,16,17,18,19]. A defect in testicular enzymatic antioxidants, such as superoxide dismutase, as well as an increase in sperm reactive oxygen species (ROS) generation, has been documented in Pb-exposed animals [15,16,17,18,19]. Several reproductive dysfunctions including histological evidence of testicular damage inhibited spermatogenesis, and a reduction in sperm quality and quantity have also been shown in animals exposed to Pb [15,16,17,18,19]. Hence, antioxidants may have the potential as protective agents against Pb-induced reproductive toxicity. Protective effects of several chemicals such as vitamin C, vitamin E, β-carotene, zinc, selenium, and polyphenol compounds have been investigated against Pb-induced reproductive toxicity [6, 17,18,19,20]. Sperm mitochondria also seem to be a potential target of Pb toxicity [21,22,23]. As proper mitochondrial function and enough level of energy guarantee proper sperm motility and function, mitochondrial injury leads to reduced sperm parameters and reproductive failure [24, 25]. Hence, in the current study, the involvement of sperm mitochondrial function in Pb-exposed rats was also evaluated as potential mechanisms of protection provided by carnosine and histidine.
Carnosine (CAR), a dipeptide composed of L-histidine (HIS) and β-alanine, is a potential endogenous antioxidant found mainly in skeletal and cardiac muscle, brain, and other organs in humans [26,27,28]. Numerous vital functions are reported for CAR [29,30,31,32]. Due to antioxidant and membrane-stabilizing activity of CAR under physiological conditions, it has been established that CAR prevents lipid peroxidation and oxidative damage to proteins [33]. This dipeptide also inactivates highly reactive aldehydes produced from degenerated biomembrane lipids [34]. Furthermore, the beneficial effect of CAR in the reproductive system has been widely investigated [35, 36]. Hence, in the current study, we aimed to assess the potential protective effect of CAR in Pb-induced reproductive toxicity and its potential mechanism(s) of protective properties.
It has been shown that some amino acids protect several types of animal cells against hypothermia and have ameliorative effects on sperm parameters in cryopreservative extenders [37]. There is a good body of literature explaining the protective function of amino acids in cryoprotection [38,39,40,41,42,43]. Among them, it has been reported that HIS cause a decrease in polyunsaturated fatty acid dilapidation of spermatozoa under peroxidation stress in humans [44]. The protective effects of this amino acid in other experimental models have also been evaluated [45,46,47]. Hence, another aim of the current study was to determine the protective effect of HIS against Pb-induced reproductive toxicity.
Materials and Methods
Chemicals
2′,7′ Dichlorofluorescein diacetate (DCFH-DA), 3-(N-morpholino) propane sulfonic acid (MOPS), 3-[4,5dimethylthiazol-2-yl]-2,5-diphenyltetrazolium bromide (MTT), 4,2-Hydroxyethyl,1-piperazineethanesulfonic acid (HEPES), bovine serum albumin (BSA), lead (Pb) acetate, dimethyl sulfoxide (DMSO), thiobarbituric acid (TBA), dithiobis-2nitrobenzoic acid (DTNB), glutathione (GSH), malondialdehyde (MDA), eosine, Coomassie brilliant blue, negrosine, ethylene glycol-bis (2-aminoethylether)-N,N,N′,N′-tetraacetic acid (EGTA), sucrose, KCl, NaCl, dithiothreitol (DTT), Na2HPO4, MgCl2, Rhodamine 123 (Rh 123), and ethylenediaminetetraacetic acid (EDTA) were purchased from Sigma Chemical Co. (St. Louis, MO, USA). Trichloroacetic acid (TCA) and hydroxymethyl aminomethane hydrochloride (Tris–HCl) were purchased from Merck (Darmstadt, Germany). The salts for preparing buffer solutions were of the analytical grade and obtained from Merck (Darmstadt, Germany).
Animals and Treatments
Mature male Sprague-Dawley (SD) rats (n = 48; 300–320 g) were purchased from Experimental and Comparative Medicine Research Center of Shiraz University of Medicine, Shiraz, Iran. Animals were maintained under controlled conditions (12:12 h, photoschedule; 18–22 °C; appropriate ventilation, ≈ 40% humidity). Animals had free access to tap water and commercial rodent pellets (Behparvar®, Tehran, Iran). All animal procedures were performed in compliance with the regulations and guidelines of the local ethics committee at Shiraz University of Medical Sciences, Shiraz, Iran (95-01-36-11290). Animals were allotted to six groups (n = 8 in each group). The treatments were as follows: (a) control (vehicle-treated), (b) Pb-acetate (20 mg/kg/day, gavage), (c) Pb-acetate + carnosine (250 mg/kg/day, gavage), (d) Pb-acetate + carnosine (500 mg/kg/day, gavage), (e) Pb-acetate + histidine (250 mg/kg/day, gavage), (f) Pb-acetate + histidine (500 mg/kg/day, gavage). Pb-acetate, carnosine, and histidine were administered daily for 14 consecutive days. On day 15, the animals were anesthetized, and samples collected. HIS and CAR were administered at least 6 h after Pb-acetate.
Sample Collection
Animals were anesthetized (Thiopental 70 mg/kg, i.p.) and the epididymides and testes were excised and weighed. The left testes were kept in 10% formalin for histopathological evaluations. Total antioxidant capacity, lipid peroxidation, reactive oxygen species (ROS) production, protein carbonylation, and glutathione contents were determined in the right testes. Sperm was collected from the left cauda epididymis.
Testis Weight Index
Testicular weight index (WI) was determined as WI = [wet weight of organ (g) / body weight (g)] × 100.
Sperm Quality Evaluation
Epididymal sperm count and sperm progressive motility were determined as previously reported [48]. Briefly, epididymal sperm was extracted by mincing the caudal epididymis in warm phosphate-buffered saline (PBS; 35 °C; pH = 7.4). Sperm progressive motility was recorded by putting a drop of sperm suspension on a glass slide covered with a coverslip and observing the sperm under a Zeiss (Jena, Germany) compound light microscope (400 × magnification) equipped with hot-stage (35 °C). The hypo-osmotic swelling (HOS) test was performed by mixing 10 μL of sperm suspension with 50 μL of hypo-osmotic solution (50-mOsm NaCl) for 10 min at 37 °C by evaluating the sperm percentage with a swollen “bubble” around the curled flagellum by counting 200 cells on each slide, using light microscopy (× 1000 magnification) [49, 50]. Sperm concentration was determined by placing 10 μL of diluted epididymal fluid on a Neubauer hemocytometer using a light microscope (× 200 magnification). Sperm abnormality and viability were monitored in duplicate (200 sperm per sample) after eosin-nigrosin staining [51]. Spermatozoa with protoplasmic droplets, ab-axial tail, malformed heads, double tails, coiled tails, bent tails, without tail and head, were recorded as abnormal under a phase-contrast microscope (Olympus BX41; Olympus Optical Co. Ltd., Japan) [52, 53].
Sperm Mitochondrial Dehydrogenase Activity (MTT Assay)
The 3-(4, 5-dimethylthiazol-2-yl)-2, 5-diphenyltetrazolium bromide (MTT) assay was used for colorimetric measurement of mitochondrial dehydrogenase activity in sperm [52, 53]. For this purpose, sperm samples (1 mg protein/mL) were incubated with 40 μL MTT solution (5 mg/mL) at 37 °C for 30 min. The reaction products (purple formazan crystals) were dissolved in dimethyl sulfoxide (DMSO; 1 mL), and the optical density (OD) of the dissolved formazan was determined at λ = 570 nm using an EPOCH plate reader (BioTek Instruments, Highland Park, USA) [54, 55].
Sperm Mitochondrial Membrane Potential
Mitochondrial uptake of the cationic fluorescent dye, rhodamine-123, was applied for the determination of sperm mitochondrial membrane potential [55, 56]. Briefly, sperm samples (1 mg protein/mL) were incubated with 10 μM of rhodamine-123 for 30 min at 35 °C under dark conditions. After centrifugation (10,000×g, 4 °C), the fluorescence intensity of supernatant was measured (λ excitation = 485 nm and λ emission = 525 nm) using a FLUOstar Omega® multifunctional microplate reader (BMG LABTECH, Germany) [55, 56].
Testicular and Sperm Reactive Oxygen Species
Testicular and sperm ROS levels were measured using the fluorescent probe dichlorofluorescein diacetate (DCFH-DA) [56, 57]. Briefly, DCFH-DA was added (10 μM final concentration) to sperm and homogenized testicular samples (1 mg protein/ml). Samples were incubated for 30 min at 35 °C in the dark. Afterward, the DCF fluorescence intensity was recorded using a FLUOstar Omega® multifunctional microplate reader (BMG LABTECH, Germany) (λ excitation = 485 nm and λ emission = 525 nm) [52, 58].
Testicular and Sperm Lipid Peroxidation
Thiobarbituric acid reactive substances (TBARS), as an index of lipid peroxidation, were measured in the testis. For this purpose, 500 mg of testis tissue homogenate (10% w/v in KCl, 1.15% w/v) or samples of 106 sperm/ml was added to a reaction mixture consisting of 1 mL thiobarbituric acid (0.375%, w/v) and 3 mL phosphoric acid (1% w/v, pH = 2) [53, 59]. The mixture was incubated at 100 °C for 45 min. Afterward, 2 mL of n-butanol was added and vigorously mixed. Finally, specimens were centrifuged (10,000×g for 5 min) and absorbance of the developed color in n-butanol phase was measured at λ = 532 nm using an Ultrospec 2000®UV spectrophotometer (Scinteck Instruments, USA) [60].
Testicular and Sperm Glutathione Content
Testicular and sperm glutathione (GSH) levels were determined spectrophotometrically using 5, 50-dithiobis-2-nitrobenzoic acid (DTNB) as the indicator [53, 61]. Briefly, testis tissue (200 mg) or sperm (106 cells/ml) samples were homogenized (Heidolph, Germany) in 8 mL of ice-cooled EDTA solution (20 mM). Then, 5 mL of the homogenate was mixed with 4 mL distilled water and 1 mL trichloroacetic acid (50% w/v). The mixture was gently shaken and centrifuged (10,000×g, 10 min, 4 °C). Then, 2 mL of the supernatant was mixed with 4 mL Tris buffer (pH = 8.9) and 100 μL Ellman’s reagent (DTNB, 10 mM in methanol) [62, 63]. The intensity of the developed yellow color was measured at λ = 412 nm using an Ultrospec 2000®UV spectrophotometer Pharmacia Biotech® UV spectrophotometer (Scinteck Instruments, United States).
Ferric-Reducing Antioxidant Power
The ferric-reducing antioxidant power (FRAP) of testicular tissue and sperm samples was measured in each experimental group. The FRAP assay evaluates changes in the absorbance at λ = 593 nm due to the formation of a blue-colored Fe2+-tripyridyltriazine from the colorless oxidized Fe3+ form by the action of electron-donating antioxidants [53, 64]. Briefly, the working FRAP mixture was freshly prepared by mixing 10 parts of 300 mmol/L acetate buffer (pH = 3.6) with 1 part of 10 mmol/L TPTZ (2, 4, 6-tripyridyl-s-triazine) in 40 mmol/L hydrochloric acid and with 1 part of 20 mmol/L ferric chloride. Testicular or sperm samples was homogenized in Tris–HCl buffer (0.25 M; pH = 7.4; 4 °C), containing 0.2 M sucrose and 5 mM dithiothreitol (DTT) [65]. Afterward, 1.5 mL FRAP reagent and 150 μL deionized water were added to 50 μL testicular tissue homogenate and incubated at 37 °C for 5 min. The intensity of the resultant blue color was measured at λ = 593 nm using an Ultrospec2000® spectrophotometer (Scinteck Instruments, United States) [66]. The protein content of the sample was measured, using the Bradford method, for standardization of data [67].
Testicular Histopathology
For histopathological evaluations, testicular specimens were fixed in buffered formalin solution (0.4% NaH2PO4, 0.64% Na2HPO4, and 10% formaldehyde in distilled water; pH = 7.4). The samples were rinsed with running water, dehydrated in graded alcohol, cleared in xylene, and embedded in paraffin; paraffin-embedded samples were cut into 5-μm sections with a rotary microtome and stained with hematoxylin-eosin stain. Tissue histopathological alterations were evaluated by a pathologist in a blind manner using a light microscope (Olympus BX41; Olympus Optical Co. Ltd., Japan). Testicular tubular injuries were monitored, and a four-level grading scale was used to quantify the histopathological alteration [68, 69]. Grade 1 injury shows a typical testicular structure with an orderly arrangement of germinal cells. Grade 2 injury demonstrates less orderly, no cohesive germinal cells, and firmly packed seminiferous tubules. Grade 3 injury exhibits disordered, sloughed-off germinal cells with shrunken, pyknotic nuclei, and less distinct seminiferous tubule borders. Grade 4 injury shows seminiferous tubules that are tightly packed with coagulative necrosis of the germinal cells [68, 69]. For testis tubular desquamation, the seminiferous tubules were evaluated for the existence of complete spermatogenesis and focal or diffuse atrophy or tubular degeneration, depending on the number of affected tubules. Early intratubular desquamation was graded as mild (+), moderate (++), or severe (+++) [68, 69]. The existence of multinucleated cells, within the tubular lumen or among spermatogenic cells, was recorded [68, 69]. The existence of degenerative alterations in interstitial Leydig cells was also studied (e.g., nuclear atrophy or cytoplasmic vacuolization) [68, 69]. The spermatogenic index was reported based on the ratio of the number of seminiferous tubules with spermatozoids to the empty tubules [68, 69].
Statistical Analysis
Data were expressed as mean ± SD. Data analysis was performed by the one-way analysis of variance (ANOVA), and mean comparison performed using the Tukey’s multiple comparison test as the post hoc test at P < 0.05 (SPSS software, version 19, IBM Corporation, NY, USA).
Results
Body and testicular weight were lower in Pb-treated rats (Fig. 1), but carnosine (CAR; 250 and 500 mg/kg) and histidine (HIS; 500 mg/kg) supplementation mitigated the lead-induced decrease in testis and body weights (Fig. 1). The effect of CAR and HIS on animal body weight or testicular weight index was not dose-dependent in the current study (Fig. 1).
Effect of lead on live and testicular weights in the rat. Pb lead; CAR carnosine; HIS histidine. Data are given as mean ± SD (n = 8). ***Significantly different from control (P < 0.001). aSignificantly different from Pb-treated group (P < 0.001). ns not significant as compared with Pb-treated group (P > 0.05)
Assessment of sperm parameters in Pb-treated rats revealed that exposure to Pb caused a significant decrease in sperm motility, viability, and membrane integrity (Fig. 2). A significant increase in the amount of abnormal sperm was also evident in Pb-treated animals (Fig. 2). On the other hand, improvements in these attributes were observed consequent to CAR (250 and 500 mg/kg) and HIS (250 and 500 mg/kg) administration (Fig. 2).
Evaluating sperm markers of oxidative stress revealed significant ROS formation, lipid peroxidation, glutathione depletion, and decreased antioxidant capacity in Pb-treated rats (Fig. 3). It was found that (250 and 500 mg/kg) and HIS (250 and 500 mg/kg) supplementation significantly mitigated the oxidative stress markers in sperm isolated from Pb-treated animals (Fig. 3).
Reactive oxygen species (ROS) formation, lipid peroxidation, glutathione content, mitochondrial dehydrogenases activity, and mitochondrial depolarization in the sperm of lead-treated rats. Pb lead; CAR carnosine; HIS histidine. Data are shown as mean ± SD (n = 8). ***Significantly different from control (P < 0.001). aSignificantly different from Pb-treated group (P < 0.001). bSignificantly different from Pb-treated group (P < 0.05)
Impairment in sperm mitochondrial function was revealed by an increment in mitochondrial depolarization and a decline in mitochondrial dehydrogenase activity in the Pb-treated group (Fig. 3). It was found that (250 and 500 mg/kg) and HIS (250 and 500 mg/kg) abated the collapse of sperm mitochondrial membrane potential and preserved mitochondrial dehydrogenase activity in the sperm of Pb-treated rats (Fig. 3).
Testis tissue histopathological changes revealed the severe tubular injury, tubular desquamation, and low spermatogenesis index in Pb-treated rats (Fig. 4 and Table 1). CAR and HIS supplementation decreased lead-induced testicular changes (Fig. 4 and Table 1).
Photomicrographs of the testicular architecture of lead-treated rats. a Tissue from control (vehicle-treated) animals revealed normal testis tissue architecture (Table 1). b Histopathological changes in the lead (Pb)-exposed rats (20 mg/kg/day for 14 consecutive days) revealed as tubular injury, tubular desquamation, and low spermatogenic index (Table 1). c, d Carnosine (250 and 500 mg/kg respectively) treatment alleviated lead (Pb)-induced testis tissue histopathological changes (Table 1). e, f Histidine (250 and 500 mg/kg respectively)-treated rats showed mild testicular injury (Table 1).
A significant increase in biomarkers of oxidative stress in the testis tissue of Pb-treated animals was evident as elevated ROS formation and lipid peroxidation (Table 2). On the other hand, testis tissue antioxidant capacity was decreased, and glutathione reservoirs were depleted in Pb-treated animals in comparison with the control group (Table 2). It was found that CAR (250 and 500 mg/kg) and HIS (500 mg/kg) mitigated oxidative stress and its consequences in the testis tissue of Pb-treated rats (Table 2). The effect of CAR and HIS on tissue biomarkers of oxidative stress was not dose-dependent in the current study (Table 2).
Its noteworthy to mention that administration of CAR or HIS (500 mg/kg/day) alone did not significantly affect the testis and sperm parameters of interest in the current investigation (Data not shown). It should also mention that the protective properties of HIS and CAR against Pb-induced decrease in rat sperm functionality were not dose-dependent in the current study (Figs. 2 and 3). On the other hand, no significant differences between the protective properties of HIS and CAR were found neither at sperm nor the mitochondria level (Figs. 2 and 3).
Discussion
Pb exposure is well established to have detrimental effects on several organs including the reproductive system. It has been shown that Pb interrupts reproductive hormonal balance and prompts oxidative stress in the rat testes [70]. Hence, antioxidants might serve as potential protective agents against lead-induced reproductive toxicity. Results of the current investigation supported the protective effects of carnosine and histidine against Pb reproductive toxicity.
We found an increase in the testicular and sperm mitochondrial ROS biosynthesis and sperm MDA levels, in addition to severe testis morphological alterations in Pb-treated rats. Moreover, poor sperm parameters including sperm motility, viability, abnormality, and membrane integrity and impairment in reproductive mitochondrial indices such as mitochondrial depolarization and decrease in mitochondrial dehydrogenase activity were also found in the current study. On the other hand, biomarkers of oxidative stress were increased where total antioxidant capacity was decreased in Pb-treated rats.
The effect of Pb on body weight and relative testicular weight percentage were significantly lower than in other groups. These observations are in agreement with the report of Acharya et al. [71] who reported a significant decrease in the body and testicular weights in animals treated with a single dosage of Pb, which they attributed to excessive ROS generation. However, there are reports of an increase in testicular weight due to acute Pb-administration which were attributed to interstitial edema due to fluid accumulation in the testis [70, 72].
The high content of polyunsaturated fatty acids in the sperm plasma membrane makes it susceptible to lipid peroxidation and triggering a higher TBARS synthesis by ROS generation [73]. The defect in testis and sperm antioxidant enzymes and ROS-induced biomembrane disruption (Fig. 3) might be a reason for alteration in sperm membrane integrity in Pb-treated animals [74]. Our results indicated that CAR and HIS supplementation could inhibit Pb-induced oxidative stress and reproductive toxicity (Fig. 5).
Glutathione (GSH) and its associated enzyme systems are the most important cellular defense mechanism against xenobiotics; GSH is also an essential antioxidant for quenching free radicals [75]. The deleterious effects of Pb on cellular glutathione stores were also documented by previous investigators [21, 76, 77]. Lead might inactivate glutathione by binding to the sulfhydryl groups. In the current investigation, we found that Pb significantly depleted testicular and sperm glutathione contents. In line with our observation, Dkhil et al. reported considerable reduction in testicular superoxide dismutase, catalase, glutathione reductase, and glutathione peroxidase activities, following administration of Pb [70]. Similar to our findings regarding the FRAP test data as an index of total antioxidant capacity, Dkhil et al. [70] observed that Pb intoxication induced a falling down of the antioxidative defense in the rat testes. Therefore, it seems the antioxidative properties of CAR and HIS might play a primary role in their protective actions against Pb reproductive toxicity [70].
It is known that Pb adversely affects mitochondrial function. The inhibition of mitochondrial oxidative phosphorylation, collapse of mitochondrial membrane potential, inhibition of mitochondrial respiratory enzyme activities, ATP depletion, and energy crisis are adverse effects reported in different models of Pb-induced toxicity [21,22,23, 78, 79]. Our data also support these findings; hence, mitochondrial dysfunction might serve as an essential mechanism in Pb reproductive toxicity. On the other hand, the regulation of mitochondrial function is an essential feature of CAR and HIS [60, 80,81,82,83,84]. In this study, CAR and HIS supplementation preserved sperm mitochondrial membrane potential and dehydrogenase activity. Therefore, these chemicals might prevent Pb-induced toxicity through regulating mitochondrial function (Fig. 5).
Formation of ROS and mitochondrial dysfunction are also two mechanistically interrelated events [85, 86]. It is well known that cellular mitochondria are the primary source of intracellular ROS formation [86]. Excessive cellular ROS could also deteriorate proper mitochondrial function [87, 88]. Hence, oxidative stress might be a cause or a consequence of mitochondrial injury [87, 88]. Thereby, CAR and HIS could decrease ROS level not only by direct scavenging of reactive species but also by regulating sperm mitochondrial function (Fig. 5).
Acute exposure to Pb might enhance membrane susceptibility to injury and trigger a malfunction in testes and sperm by altering the major components of sperm biological membranes and polyunsaturated fatty acids. In the current study, simultaneous administration of CAR and HIS to Pb-treated rats revealed an antioxidative role and also ameliorative effects on testicular histopathological alteration against detrimental effects of Pb. Hence, the antioxidative properties of CAR and HIS might play a crucial role in scavenging the ROS induced by Pb in the testes and sperm (Fig. 5).
Metal ion chelation is an interesting feature of the peptide CAR as well as the amino acid HIS [89,90,91]. Although the capability of these chemicals for chelation of different metal ions could be variable, their metal ion-chelating properties might play a role in the mechanism of protection against Pb-induced reproductive toxicity. In the current study, a high dose of Pb (20 mg/kg/day, oral, 14 consecutive days) was administered. On the other hand, CAR and HIS were administered at least 6 h after Pb which might decrease the chance of interaction between Pb and these chemicals in the gastrointestinal tract. However, the lack of serum and testicular level of Pb might serve as a limitation for the current study. Hence, evaluating serum and tissue level of Pb, as well as CAR and HIS, might help to provide more explicit idea on their mechanism of protection against Pb toxicity.
Collectively, our data indicate that the antioxidative and mitochondria-protecting properties of CAR and HIS play a primary role in the protective properties of these molecules against Pb-induced reproductive toxicity. Therefore, CAR and HIS treatment may be used in future investigations designed for the study of reproductive toxicants where oxidative stress and mitochondrial injuries are involved.
References
Wirth JJ, Mijal RS (2010) Adverse effects of low level heavy metal exposure on male reproductive function. Syst Biol Reprod Med 56:147–167
Yu Y, Han Y, Niu R, Wang J, Manthari RK, Ommati MM et al (2017) Ameliorative effect of VE, IGF-I, and hCG on the fluoride-induced testosterone release suppression in mice Leydig cells via the up-regulation of Star and Cyp11a expression. Biol Trace Elem Res 181:95–103
Sun Z, Li S, Yu Y, Chen H, Ommati MM, Manthari RK et al (2017) Alterations in epididymal proteomics and antioxidant activity of mice exposed to fluoride. Arch Toxicol 92:169–180
Elgawish RAR, Abdelrazek HMA (2014) Effects of lead acetate on testicular function and caspase-3 expression with respect to the protective effect of cinnamon in albino rats. Toxicol Rep 1:795–801
Kalia K, Flora SJ (2005) Strategies for safe and effective therapeutic measures for chronic arsenic and lead poisoning. J Occup Health 47:1–21
Hsu PC, Guo YL (2002) Antioxidant nutrients and lead toxicity. Toxicology 180:33–44
Bressler J, Kim KA, Chakraborti T, Goldstein G (1999) Molecular mechanisms of lead neurotoxicity. Neurochem Res 24:595–600
Patra RC, Swarup D, Dwivedi SK (2001) Antioxidant effects of alpha tocopherol, ascorbic acid and L-methionine on lead induced oxidative stress to the liver, kidney and brain in rats. Toxicology 162:81–88
Sandhir R, Gill KD (1995) Effect of lead on lipid peroxidation in liver of rats. Biol Trace Elem Res 48:91–97
Othman AI, El Missiry MA (1998) Role of selenium against lead toxicity in male rats. J Biochem Mol Toxicol 12:345–349
Humphreys DJ (1991) Effects of exposure to excessive quantities of lead on animals. Br Vet J 147:18–30
Ercal N, Neal R, Treeratphan P, Lutz PM, Hammond TC, Dennery PA et al (2000) A role for oxidative stress in suppressing serum immunoglobulin levels in lead-exposed Fisher 344 rats. Arch Environ Contam Toxicol 39:251–256
Skoczynska A, Smolik R, Jelen M (1993) Lipid abnormalities in rats given small doses of lead. Arch Toxicol 67:200–204
Adonaylo VN, Oteiza PI (1999) Lead intoxication: antioxidant defenses and oxidative damage in rat brain. Toxicology 135:77–85
Abdel-Moneim AE, Dkhil MA, Al-Quraishy S (2011) The redox status in rats treated with flaxseed oil and lead-induced hepatotoxicity. Biol Trace Elem Res 143:457–467
Oberley TD, Friedman AL, Moser R, Siegel FL (1995) Effects of lead administration on developing rat kidney. II. Functional, morphologic, and immunohistochemical studies. Toxicol Appl Pharmacol 131:94–107
Hsu PC, Hsu CC, Liu MY, Chen LY, Guo YL (1998) Lead-induced changes in spermatozoa function and metabolism. J Toxicol Environ Health A 55:45–64
Shastri D, Kumar M, Kumar A (1999) Modulation of lead toxicity by Spirulina fusiformis. Phytother Res 13:258–260
Batra N, Nehru B, Bansal MP (1998) The effect of zinc supplementation on the effects of lead on the rat testis. Reprod Toxicol 12:535–540
Wang J, Yang Z, Zhu H, Lin L, Liu Z (2012) Lead-induced oxidative stress and protective effect of naringenin on testis tissues of rats. CNKI 19:2012–2019
Xu J, Ji LD, Xu LH (2006) Lead-induced apoptosis in PC 12 cells: involvement of p53, Bcl-2 family and caspase-3. Toxicol Lett 166:160–167
Yin S-T, Tang M-L, Su L, Chen L, Hu P, Wang H-L et al (2008) Effects of Epigallocatechin-3-gallate on lead-induced oxidative damage. Toxicology 249:45–54
Ma L, Liu J-Y, Dong J-X, Xiao Q, Zhao J, Jiang F-L (2017) Toxicity of Pb2+ on rat liver mitochondria induced by oxidative stress and mitochondrial permeability transition. Toxicol Res 6:822–830
Amaral A, Lourenço B, Marques M, Ramalho-Santos J (2013) Mitochondria functionality and sperm quality. Reproduction 146:R163–RR74
Piomboni P, Focarelli R, Stendardi A, Ferramosca A, Zara V (2012) The role of mitochondria in energy production for human sperm motility. Int J Androl 35:109–124
Margolis FL, Grillo M, Hempstead J, Morgan JI (1987) Monoclonal antibodies to mammalian carnosine synthetase. J Neurochem 48:593–600
Roberts PR, Zaloga GP (2000) Cardiovascular effects of carnosine. Biochemistry (Mosc) 65:856–861
Horinishi H, Grillo M, Margolis FL (1978) Purification and characterization of carnosine synthetase from mouse olfactory bulbs. J Neurochem 31:909–919
Aydın AF, Küçükgergin C, Özdemirler-Erata G, Koçak-Toker N, Uysal M (2009) The effect of carnosine treatment on prooxidant–antioxidant balance in liver, heart and brain tissues of male aged rats. Biogerontology 11:103–109
Fu H, Katsumura Y, Lin M, Muroya Y, Hata K, Fujii K et al (2009) Free radical scavenging and radioprotective effects of carnosine and anserine. Radiat Phys Chem 78:1192–1197
Guiotto A, Calderan A, Ruzza P, Borin G (2005) Carnosine and carnosine-related antioxidants: a review. Curr Med Chem 12:2293–2315
Kohen R, Yamamoto Y, Cundy KC, Ames BN (1988) Antioxidant activity of carnosine, homocarnosine, and anserine present in muscle and brain. Proc Nat Acad Sci 85:3175–3179
Nagasawa T, Yonekura T, Nishizawa N, Kitts DD (2001) In vitro and in vivo inhibition of muscle lipid and protein oxidation by carnosine. Mol Cell Biochem 225:29–34
Aldini G, Granata P, Carini M (2002) Detoxification of cytotoxic alpha,beta-unsaturated aldehydes by carnosine: characterization of conjugated adducts by electrospray ionization tandem mass spectrometry and detection by liquid chromatography/mass spectrometry in rat skeletal muscle. J Mass Spectrom 37:1219–1228
Abbasoğlu L, Kalaz EB, Soluk-Tekkeşin M, Olgaç V, Doğru-Abbasoğlu S, Uysal M (2012) Beneficial effects of taurine and carnosine in experimental ischemia/reperfusion injury in testis. Pediatr Surg Int 28:1125–1131
Aydın AF, Küçükgergin C, Çoban J, Doğan-Ekici I, Doğru-Abbasoğlu S, Uysal M et al (2018) Carnosine prevents testicular oxidative stress and advanced glycation end product formation in D-galactose-induced aged rats. Andrologia 50. https://doi.org/10.1111/and.12939
Trimeche A, Yvon JM, Vidament M, Palmer E, Magistrini M (1999) Effects of glutamine, proline, histidine and betaine on post-thaw motility of stallion spermatozoa. Theriogenology 52:181–191
Akahane T, Tsuchiya T, Matsumoto JJ (1981) Freeze denaturation of carp myosin and its prevention by sodium glutamate. Cryobiology 18:426–435
Tsuchiya T, Tsuchiya Y, Nonomura Y, Matsumoto JJ (1975) Prevention of freeze denaturation of carp actomyosin by sodium glutamate. J Biochem 77:853–862
Heinz KA, Glofcheski DJ, Lepock JR, Kruuv J (1990) Mechanism of freeze-thaw damage to liver alcohol dehydrogenase and protection by cryoprotectants and amino acids. Cryobiology 27:521–538
Carpenter JF, Crowe JH (1988) The mechanism of cryoprotection of proteins by solutes. Cryobiology 25:244–255
Lalonde RJ, Lepock JR, Kruuv J (1991) Site of freeze-thaw damage and cryoprotection by amino acids of the calcium ATPase of sarcoplasmic reticulum. Biochim Biophys Acta 1079:128–138
Carpenter JF, Hand SC, Crowe LM, Crowe JH (1986) Cryoprotection of phosphofructokinase with organic solutes: characterization of enhanced protection in the presence of divalent cations. Arch Biochem Biophys 250:505–512
Griveau JF, Dumont E, Renard P, Callegari JP, Le Lannou D (1995) Reactive oxygen species, lipid peroxidation and enzymatic defence systems in human spermatozoa. J Reprod Fertil 103:17–26
El-Batch M, Ibrahim W, Said S (2011) Effect of histidine on autotaxin activity in experimentally induced liver fibrosis. J Biochem Mol Toxicol 25:143–150
Farshid AA, Tamaddonfard E, Belasius MS, Hamzeh-Gooshchi N et al (2009) Histopathological comparison of the effects of histidine and ketotifen in a rat model of colitis. Bull Vet Inst Pulawy 53:795–800
Farshid AA, Tamaddonfard E, Yahyaee F (2010) Effects of histidine and N-acetylcysteine on diclofenac-induced anti-inflammatory response in acute inflammation in rats. Indian J Exp Biol 48:1136–1142
Ommati MM, Zamiri MJ, Akhlaghi A, Atashi H, Jafarzadeh MR, Rezvani MR et al (2013) Seminal characteristics, sperm fatty acids, and blood biochemical attributes in breeder roosters orally administered with sage (Salvia officinalis) extract. Anim Prod Sci 53:548–554
Fonseca JF, Torres CAA, Maffili VV, Borges AM, Santos ADF, Rodrigues MT et al (2005) The hypoosmotic swelling test in fresh goat spermatozoa. Anim Reprod 2:139–144
Ommati MM, Heidari R, Zamiri MJ, Shojaee S, Akhlaghi A, Sabouri S (2017) Association of open field behavior with blood and semen characteristics in roosters: as an alternative animal model. Int Androl. https://doi.org/10.1016/j.androl.2017.02.002
Pursel VG, Johnson LA, Rampacek GB (1972) Acrosome morphology of boar spermatozoa incubated before cold shock. J Anim Sci 34:278–283
Ommati MM, Heidari R, Jamshidzadeh A, Zamiri MJ, Sun Z, Sabouri S et al (2018) Dual effects of sulfasalazine on rat sperm characteristics, spermatogenesis, and steroidogenesis in two experimental models. Toxicol Lett 284:46–55
Ommati MM, Tanideh N, Rezakhaniha B, Wang J, Sabouri S, Vahedi M et al (2018) Is immunosuppression, induced by neonatal thymectomy, compatible with poor reproductive performance in adult male rats? Andrology 6:199–213
Niknahad H, Jamshidzadeh A, Heidari R, Abdoli N, Ommati MM, Jafari F et al (2016) The postulated hepatotoxic metabolite of methimazole causes mitochondrial dysfunction and energy metabolism disturbances in liver. Pharm Sci 22:217–226
Jamshidzadeh A, Heidari R, Abasvali M, Zarei M, Ommati MM, Abdoli N et al (2017) Taurine treatment preserves brain and liver mitochondrial function in a rat model of fulminant hepatic failure and hyperammonemia. Biomed Pharmacother 86:514–520
Caro AA, Adlong LW, Crocker SJ, Gardner MW, Luikart EF, Gron LU (2012) Effect of garlic-derived organosulfur compounds on mitochondrial function and integrity in isolated mouse liver mitochondria. Toxicol Lett 214:166–174
Niknahad H, Jamshidzadeh A, Heidari R, Hosseini Z, Mobini K, Khodaei F et al (2016) Paradoxical effect of methimazole on liver mitochondria: in vitro and in vivo. Toxicol Lett 259:108–115
Heidari R, Ghanbarinejad V, Mohammadi H, Ahmadi A, Esfandiari A, Azarpira N et al (2018) Dithiothreitol supplementation mitigates hepatic and renal injury in bile duct ligated mice: potential application in the treatment of cholestasis-associated complications. Biomed Pharmacother 99:1022–1032
Heidari R, Ghanbarinejad V, Mohammadi H, Ahmadi A, Ommati MM, Abdoli N et al (2018) Mitochondria protection as a mechanism underlying the hepatoprotective effects of glycine in cholestatic mice. Biomed Pharmacother 97:1086–1095
Jamshidzadeh A, Niknahad H, Heidari R, Zarei M, Ommati MM, Khodaei F (2017) Carnosine protects brain mitochondria under hyperammonemic conditions: relevance to hepatic encephalopathy treatment. PharmaNutrition 5:58–63
Sedlak J, Lindsay RH (1968) Estimation of total, protein-bound, and nonprotein sulfhydryl groups in tissue with Ellman’s reagent. Anal Biochem 25:192–205
Niknahad H, Heidari R, Mohammadzadeh R, Ommati MM, Khodaei F, Azarpira N et al (2017) Sulfasalazine induces mitochondrial dysfunction and renal injury. Ren Fail 39:745–753
Heidari R, Jamshidzadeh A, Niknahad H, Mardani E, Ommati MM, Azarpira N et al (2016) Effect of taurine on chronic and acute liver injury: focus on blood and brain ammonia. Toxicol Rep 3:870–879
Katalinic V, Modun D, Music I, Boban M (2005) Gender differences in antioxidant capacity of rat tissues determined by 2,2′-azinobis (3-ethylbenzothiazoline 6-sulfonate; ABTS) and ferric reducing antioxidant power (FRAP) assays. Comp Biochem Physiol 140:47–52
Heidari R, Moezi L, Asadi B, Ommati MM, Azarpira N (2017) Hepatoprotective effect of boldine in a bile duct ligated rat model of cholestasis/cirrhosis. PharmaNutrition 5:109–117
Heidari R, Jamshidzadeh A, Niknahad H, Safari F, Azizi H, Abdoli N et al (2016) The hepatoprotection provided by taurine and glycine against antineoplastic drugs induced liver injury in an ex vivo model of normothermic recirculating isolated perfused rat liver. Trends Pharmacol Sci 2:59–76
Bradford MM (1976) A rapid and sensitive method for the quantitation of microgram quantities of protein utilizing the principle of protein-dye binding. Anal Biochem 72:248–254
Blank ML, O'Neill PJ, Steigman CK, Cobb LM, Wilde RA, Havenstein PJ et al (1993) Reperfusion injury following testicular torsion and detorsion in prepubertal rats. Urol Res 21:389–393
Kianifard D, Sadrkhanlou R-A, Hasanzadeh S (2011) The histological, histomorphometrical and histochemical changes of testicular tissue in the metformin treated and untreated streptozotocin-induced adult diabetic rats. Vet Res Forum 3:13–24
Dkhil MA, Moneim AEA, Al-Quraishy S (2016) Indigofera oblongifolia ameliorates lead acetate-induced testicular oxidative damage and apoptosis in a rat model. Biol Trace Elem Res 173:354–361
Acharya UR, Acharya S, Mishra M (2003) Lead acetate induced cytotoxicity in male germinal cells of Swiss mice. Ind Health 41:291–294
Graca A, Ramalho-Santos J, de Lourdes PM (2004) Effect of lead chloride on spermatogenesis and sperm parameters in mice. Asian J Androl 6:237–241
Baumber J, Ball BA, Gravance CG, Medina V, Davies-Morel MC (2000) The effect of reactive oxygen species on equine sperm motility, viability, acrosomal integrity, mitochondrial membrane potential and membrane lipid peroxidation. J Androl 21:895–902
Bazrgar M, Goudarzi I, Lashkarbolouki T, Elahdadi Salmani M (2015) Melatonin ameliorates oxidative damage induced by maternal lead exposure in rat pups. Physiol Behav 151:178–188
Mate JM, Aledo JC, Perez-Gomez C, Esteban del Valle A, Segura JM (2000) Interrelationship between oxidative damage and antioxidant enzyme activities: an easy and rapid experimental approach. Biochem Educ 28:93–95
Kasperczyk A, Kasperczyk S, Horak S, Ostałowska A, Grucka-Mamczar E, Romuk E et al (2008) Assessment of semen function and lipid peroxidation among lead exposed men. Toxicol Appl Pharmacol 228:378–384
Chen L, Yang X, Jiao H, Zhao B (2003) Tea catechins protect against lead-induced ROS formation, mitochondrial dysfunction, and calcium dysregulation in PC12 cells. Chem Res Toxicol 16:1155–1161
Szynaka B, Andrzejewska A, Tomasiak M, Augustynowicz A (1999) Exocrine cell mitochondria of the rat pancreas after lead intoxication. Exp Toxicol Pathol 51:559–564
Xu J, Lian L-j WC, Wang X-f, Fu W-y, Xu L-h (2008) Lead induces oxidative stress, DNA damage and alteration of p53, Bax and Bcl-2 expressions in mice. Food Chem Toxicol 46:1488–1494
Hipkiss AR (2010) Aging, proteotoxicity, mitochondria, glycation, NAD+ and carnosine: possible inter-relationships and resolution of the oxygen paradox. Front Aging Neurosci 2:10
Corona C, Frazzini V, Silvestri E, Lattanzio R, Sorda RL, Piantelli M et al (2011) Effects of dietary supplementation of carnosine on mitochondrial dysfunction, amyloid pathology, and cognitive deficits in 3xTg-AD mice. PLoS One 6:e17971
Kang K-S, Yun J-W, Lee Y-S (2002) Protective effect of l-carnosine against 12-O-tetradecanoylphorbol-13-acetate- or hydrogen peroxide-induced apoptosis on v-myc transformed rat liver epithelial cells. Cancer Lett 178:53–62
Kukreja RC, Loesser KE, Kearns AA, Naseem SA, Hess ML (1993) Protective effects of histidine during ischemia-reperfusion in isolated perfused rat hearts. Am J Phys 264:H1370–H1H81
Alves MG, Oliveira PJ, Carvalho RA (2009) Mitochondrial preservation in celsior versus histidine buffer solution during cardiac ischemia and reperfusion. Cardiovasc Toxicol 9:185–193
Brookes PS, Yoon Y, Robotham JL, Anders MW, Sheu S-S (2004) Calcium, ATP, and ROS: a mitochondrial love-hate triangle. Am J Phys 287:C817–CC33
Schaffer SW, Suleiman MS (2010) Mitochondria: the dynamic organelle. Springer Science & Business Media, New York 359 p
Orrenius S, Gogvadze V, Zhivotovsky B (2007) Mitochondrial oxidative stress: implications for cell death. Annu Rev Pharmacol Toxicol 47:143–183
Ott M, Gogvadze V, Orrenius S, Zhivotovsky B (2007) Mitochondria, oxidative stress and cell death. Apoptosis 12:913–922
Canabady-Rochelle LLS, Harscoat-Schiavo C, Kessler V, Aymes A, Fournier F, Girardet J-M (2015) Determination of reducing power and metal chelating ability of antioxidant peptides: revisited methods. Food Chem 183:129–135
Leberman R, Rabin BR (1959) Metal complexes of histidine. Trans Faraday Soc 55:1660–1670
Sundberg RJ, Martin RB (1974) Interactions of histidine and other imidazole derivatives with transition metal ions in chemical and biological systems. Chem Rev 74:471–517
Funding
The authors gratefully acknowledge the Pharmaceutical Sciences Research Center and the Vice Chancellor for Research, Shiraz University of Medical Sciences for the financial support (grant no. 95-01-36-11290).
Author information
Authors and Affiliations
Corresponding author
Ethics declarations
The rats were killed according to an animal protocol that was approved by the Institutional Animal Ethics Committee of Shiraz University of Medicine (Shiraz, Iran; no. 11290).
Conflict of Interest
The authors declare that they have no conflicts of interest.
Rights and permissions
About this article
Cite this article
Ommati, M.M., Jamshidzadeh, A., Heidari, R. et al. Carnosine and Histidine Supplementation Blunt Lead-Induced Reproductive Toxicity through Antioxidative and Mitochondria-Dependent Mechanisms. Biol Trace Elem Res 187, 151–162 (2019). https://doi.org/10.1007/s12011-018-1358-2
Received:
Accepted:
Published:
Issue Date:
DOI: https://doi.org/10.1007/s12011-018-1358-2