Abstract
Corncob is an economic feedstock and more than 20 million tons of corncobs are produced annually in China. Abundant xylose can be potentially converted from the large amount of hemicellulosic materials in corncobs, which makes the crop residue an attractive alternative substrate for a value-added production of a variety of bioproducts. Lactic acid can be used as a precursor for poly-lactic acid production. Although current industrial lactic acid is produced by lactic acid bacteria using enriched medium, production by Rhizopus oryzae is preferred due to its exclusive formation of the l-isomer and a simple nutrition requirement by the fungus. Production of l-(+)-lactic acid by R. oryzae using xylose has been reported; however, its yield and conversion rate are poor compared with that of using glucose. In this study, we report an adapted R. oryzae strain HZS6 that significantly improved efficiency of substrate utilization and enhanced production of l-(+)-lactic acid from corncob hydrolysate. It increased l-(+)-lactic acid final concentration, yield, and volumetric productivity more than twofold compared with its parental strain. The optimized growth and fermentation conditions for Strain HZS6 were defined.
Similar content being viewed by others
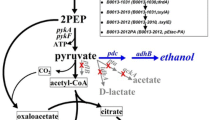
Explore related subjects
Discover the latest articles, news and stories from top researchers in related subjects.Avoid common mistakes on your manuscript.
Introduction
l-(+)-Lactic acid (L-LA) is a natural organic acid which is widely applied in food, chemical, pharmaceutical, and personal care industries, as well as production of propylene oxide, propylene glycol, and acrylic fibers. Recently, there has been an increased interest in lactic acid production because it can be used as a precursor of poly-lactic acid (PLA) production. PLA is a biodegradable and thermoplastic biomaterial commonly used as high-volume biodegradable plastics for packing and other applications which can potentially replace polyethylene, polypropylene, and polystyrene. However, the realization of this potential is dependent on whether lactic acid can be produced at a low cost which is competitive on a global scale [1, 2]. Because an optically pure L-LA is required [3], about 90% LA are produced biotechnologically worldwide [4]. Current industrial LA fermentation is mostly carried out with lactic acid bacteria from relatively expensive carbon sources such as glucose, lactose, and starch with rich nutrient [5]. Rhizopus oryzae is, however, preferred for the production of L-LA owing to its exclusive formation of the l-isomer with a simple nutritional requirement and the product can be recovered easily [6–8]. Therefore, fermentations with this fungus to produce pure L-LA have attracted increasing interest in recent years [5]. In addition, R. oryzae fermentation can use xylose, a relatively cheap simple sugar that can be readily obtained from crop and forest residues upon acid or enzymatic hydrolysis.
A large quantity of hemicellulosic materials in the form of agricultural residues such as corncobs is available in the world. For example, according to CNGOIC [9], China produces around 116 million tons of corn every year, and more than 20 million tons of corncobs generated. Xylose is the ultimate product from hemicellulose hydrolysis, and constitutes the second most abundant renewable monosaccharide in nature [10]. Therefore, corncobs could then be an economic alternative source for L-LA production [11, 12]. However, compared with the results of fermentations of glucose to LA reported by Tay and Yang [5], the production of L-LA from xylose by R. oryzae was relatively poor. Ruengruglikit and Hang [12] obtained L-LA with the yield of 299.4 g per kg dry material of corncobs from the substrate containing 50-g corncobs xylose per liter using R. oryzae after 48 h of fermentation. Such productivity is equivalent to 0.31 g l−1 h−1 compared with 2.5 g l−1 h−1 achieved using glucose. Hence, L-LA production from xylose by R. oryzae needs to be improved to make the crop residues a competent alternative substrate. The objective of this study was to develop a better strain of R. oryzae that can efficiently utilize xylose to produce high yield of L-LA from corncob hydrolysate.
Materials and Methods
Preparation of Corncob Hydrolysate
Corncobs were collected and milled to a particle size no larger than 1 mm. Acid hydrolysis of corncob was carried out in a medium containing 1.5% H2SO4 with a liquid/solid ratio of 10:1 (w/w) at 121 °C for 60 min. The remaining solid was removed from aqueous solution by filtration. The acid hydrolysate was neutralized with CaCO3 to pH 6.5, and the precipitated CaSO4 was separated from the supernatant by filtration. The composition of the corncob hydrolysate was assayed by high-performance liquid chromatography (HPLC) as described previously [13]. The corncob hydrolysate consisted of 26.5 g xylose, 2.4 g glucose, 3.2 g arabinose, and 3.5 g acetic acid per liter. The hydrolysate was concentrated by vacuum evaporation at 40 °C till the concentration of xylose reached 600 g per liter. The concentrated hydrolysate was stored at 4 °C until use. Such obtained corncob xylose was diluted at desirable concentrations before culture fermentation.
Microorganism and Culture Media
A common L-LA producer, R. oryzae strain HM11 from Collection of Institute of New Energy Technology, Tsinghua University, was used as a parental strain and maintained on potato dextrose agar (PDA). Several media were applied in this study. An adaptation medium was consisted of 30 g corncob xylose, 2 g NH4NO3, 0.2 g KH2PO4, 0.25 g MgSO4 7H2O, and 0.05 g ZnSO4 7H2O per liter. The medium used for a preculture had similar ingredients of the adaptation medium, except that corncob xylose was replaced by pure xylose. Fermentation medium contained 50–80 g of corncob xylose or pure xylose per liter with other ingredients similar as the preculture medium. To evaluate the effect of strain adaptation on LA production from corncob xylose, a mixture medium containing 40 g pure glucose and 40 g pure xylose per liter was used in fermentation experiments. To prepare a single colony isolation medium, 1 g sodium deoxycholate and 0.1 g bromocresol green were added in PDA medium per liter.
Strain Adaptation and Selection
The parent strain was cultured on 30 ml adaptation medium in a 250-ml flask at 180 rpm at 34 °C. After 36-h incubation, cells were collected by centrifugation and transferred to a fresh adaptation medium for continued cultivation. This procedure was repeated for at least 30 times until a stable culture was established. Then, a sample of 1 ml culture broth was taken, diluted in sterile physiological saline, and spread on to an isolation medium plates. The transferred culture plates were maintained at 34 °C for 36 h. A single colony was selected and transferred onto the preculture medium. This adapted strain was designated as HZS6 and was used for fermentation experiments to evaluate its L-LA productivity.
Cultural Method
Cultures were inoculated and grown on a PDA at 34 °C for 6 days. Spores were collected with an inoculating loop and suspended in distilled water. The number of spores in suspension was counted using a hemocytometer before inoculation. For each experiment, 10 ml spore suspension was inoculated to 300 ml of the preculture medium in a 1,000-ml flask to obtain a final number of spores in the medium of about 1 × 106 ml−1. Cultivation was carried out on a shaking water bath with agitation at 180 rpm at 34 °C for 48 h. To each flask, 3 g autoclaved CaCO3 was added to maintain pH after inoculation. The preculture was centrifuged and used as inoculum into the fermenter.
Fermentation experiments were performed in a 5-l BIOSTAT®B fermenter (B.Braun Biotech. Inc., Germany) with a working volume of 3 l. The cultivations were carried out at 30, 34, and 38 °C. The pH of fermentation broth was monitored using a pH sensor (Mettler Toledo, Switzerland) and maintained at 4.8, 5.3, 5.8, 6.3, 6.8, or 7.5 by automatic addition of 5 M NaOH solution. Normally, fermentations were conducted at pH 5.8 and 34 °C, unless stated otherwise. The aeration rate and agitation speed were 0.5 vvm and 300 rpm, respectively.
Analytical Methods
Culture supernatant was collected by centrifugation of 5 ml of the fermentation broth at 10,000 rpm for 5 min. Sugar consumption and LA concentrations were analyzed by HPLC as reported previously [13]. L-LA was assayed by SB-40C Biosensor analyzer (Institute of Biology, Shandong Province Academy of Science, China). The sediment was used to measure cell growth by washing the mycelia twice with distilled water. Drying was performed at 95 °C until constant weight was achieved.
Results and Discussion
Enhanced Substrate Utilization and L-LA Production by Adapted Strain HZS6
Using an adapted strain of R. oryzae HZS6, we have demonstrated its significantly improved performance in xylose utilization, and L-LA production compared with its parental strain HM11. The substrate utilization and L-LA production of strain HZS6 was significantly improved over its parental strain both on pure xylose and corncob xylose (Table 1). Its substrate utilization efficiency and L-LA yield increased almost twofold. For the fermentation of corncob hydrolysate, substrate was almost completely utilized in 78 h with an L-LA yield of 79.6%. The volumetric productivity of L-LA was improved by about 115%. In contrast, attempts to ferment these substrates with parental strains always resulted in a slow and an incomplete fermentation. An interesting observation was that the L-LA final concentration, yield, and volumetric productivity obtained in corncob hydrolysate were higher than that in pure xylose. This could be attributed by other nutrition source and by-products presented in corncob hydrolysate such as glucose and arabinose. A positive effect of glucose on xylitol production by Candida parapsilosis was also previously observed [14].
The newly developed strain HZS6 consumed glucose and xylose completely and produced L-LA at 65 g/l after 66-h fermentation (Fig. 1). In contrast, its parental strain HM11 was unable to consume xylose content and produced only about a half amount of L-LA due to consumption of glucose. It appeared that strain HZS6 acquired more efficient ability to utilize xylose and produce higher yield of L-LA. Subjected to mixed pure sugar fermentation, the adapted strain consumed glucose first, followed by almost simultaneously utilization of xylose and arabinose. Beginning with 80 g l−1 of total sugar, R. oryzae HZS6 produced 64.6 g l−1 L-LA after 66 h of fermentation with a yield of 80.8%.
The present results clearly demonstrate the significant improvement of fermentation rates and yields by the adapted strain, R. oryzae HZS6, using a relatively low-cost raw material for the manufacture of L-LA. It suggests that the inabilities of parent R. oryzae strain for producing L-LA from pentose could be improved by adaptation such as cell recycling used in this study. However, it needs to point out that mechanisms of this excellent L-LA producer generated upon repeated recycling are not known. Enhanced biotransformation of fermentation inhibitors was accomplished by more tolerant yeast strains using directed adaptation [15]. Genomic adaptation of yeast to fermentation inhibitors has been documented [16]. Numerous genes and enzymes can be significantly induced under pressure. The cell recycling could lead a selection under pressure and induction of enzymes and transporters required for efficient fermentation of pentose. Studies on the genetic changes associated with recycling and adaptation to corncob hydrolysates including isoenzymes profiles and the changes in metabolic flux distributions followed by this fungus are under investigation. Furthermore, this strain should be further improved by genetic modification to increase L-LA productivity and decrease energy consumption in aeration, although higher aeration rate could benefit the use of a novel submerged membrane bioreactor.
Optical Growth and Fermentation Conditions for Strain HZS6
The initial pH of the fermentation medium was controlled at 7.5. Then, the pH of the medium was maintained at 4.8, 5.3, 5.8, 6.3, 6.8, and 7.5, respectively, by automatic addition of 5 M NaOH to neutralize the formed L-LA. L-LA yield was the highest at pH 5.8, and reduced to either lower or higher pH values (Table 2). Cell mass was also proportional with the accumulation of L-LA. It seemed pH 5.8 was most suitable for cell growth and enzyme activities involved in the biosynthesis of L-LA production.
The effects of temperature on L-LA production from corncob hydrolysate by the adapted strain R. oryzae HZS6 were investigated beginning with xylose concentration of 80 g l−1 (total sugar 97 g l−1) at 30, 34, and 38 °C, respectively. As shown in Fig. 2, the final L-LA concentration, volumetric productivity, and biomass increased with the increase in temperature from 30 to 34 °C, but decreased with the increase in temperature from 34 to 38 °C. The optimal temperature for the production of L-LA by R. oryzae HZS6 was 34 °C.
Using corncob as a substrate, a yield of 299.4 g L-LA per kg of dry material was reported using R. oryzae [12]. Similarly, 533 g of D-LA per kg of dry material was obtained by Lactobacillus delbrueckii using corncob. Measured by yield per kg of dry material, the yield of L-LA produced by our improved strain HZS6 is converted as 772 g. Such a high yield of L-LA derived from corncob is superior to those reported performance by other microorganisms.
As demonstrated in this study, the newly adapted strain HZS6 improved L-LA productivity and yield more than twofold compared with its parental strain, and other L-LA-producing microorganisms using corncob. We conclude that xylose derived from corncob can be efficiently used in L-LA fermentation process. The adaptation of R. oryzae can be an efficient means to improve strain performance for enhanced substrate utilization and L-LA production using corncob hydrolysate. Further strain improvement is needed to improve L-LA productivity and reduce energy consumption using adaptation and genetic manipulations.
References
Datta, R., Tsai, S. P., Bonsigore, P., Moon, S. H., & Frank, J. R. (1995). FEMS Microbiology Reviews, 16, 221–231.
Yin, P., Nishhina, N., Kosakai, Y., Yahiro, K., Park, Y., & Okabe, M. (1997). Journal of Fermentation and Bioengineering, 84, 249–253.
Lunt, J. (1998). Polymer Degradation and Stability, 59, 145–152.
Hofvendahl, K., & Hahn-Hägerdal, B. (1997). Enzyme and Microbial Technology, 20, 301–307.
Tay, A., & Yang, S. T. (2002). Biotechnology and Bioengineering, 80, 1–12.
Hang, Y. D., & Suntornsuk, W. (1994). Letters in Applied Microbiology, 19, 249–252.
Kosakai, Y., Park, Y. S., & Okabe, M. (1997). Biotechnology and Bioengineering, 55, 461–470.
Yu, R. C., & Hang, Y. D. (1989). Biotechnology Letters, 11, 597–600.
CNGOIC (2003). China National Grain & Oils Information Center, Beijing, China.
Jeffries, T. W., & Jin, Y. S. (2000). Advances in Applied Microbiology, 47, 221–268.
Melzoch, K., Votruba, J., Hbov, V., & Rychtera, M. (1997). Journal of Biotechnology, 56, 25–31.
Ruengruglikit, C., & Hang, Y. D. (2003). Lebensm.-Wiss. U-Technol, 36, 573–575.
Bai, D. M., Jia, M. Z., Zhao, X. M., Ban, R., Shen, F., Li, X. G., et al. (2003). Chemical Engineering Science, 58, 785–791.
Kim, S. Y., Oh, D. K., & Kim, J. H. (1999). Biotechnology Letters, 21, 891–895.
Liu, Z. L., Slininger, P. J., & Gorsich, S. W. (2005). Applied Biochemistry and Biotechnology, 121–124, 451–460.
Liu, Z. L. (2006). Applied Microbiology and Biotechnology, 73, 27–36.
Acknowledgment
The authors are grateful to Prof. S. L. Yang (Chinese Academy of Engineering) for the helpful suggestions. This study was supported in part by a grant of UK DTI-China MOST Collaborative Research and USDA National Program 307/306.
Author information
Authors and Affiliations
Corresponding authors
Rights and permissions
About this article
Cite this article
Bai, DM., Li, SZ., Liu, Z.L. et al. Enhanced l -(+)-Lactic Acid Production by an Adapted Strain of Rhizopus oryzae using Corncob Hydrolysate. Appl Biochem Biotechnol 144, 79–85 (2008). https://doi.org/10.1007/s12010-007-8078-y
Received:
Accepted:
Published:
Issue Date:
DOI: https://doi.org/10.1007/s12010-007-8078-y