Abstract
For the last decades, the food industry has been searching for cleaner alternatives to petroleum-derived plastics to ensure food safety, such as edible films. Although tapioca starch and proteins have good film-forming abilities separately, the combination of both may enhance their properties. This study aims to investigate the effect of the combination of three concentrations (80:20 w/w, 70:30 w/w, and 60:40 w/w) of tapioca starch and protein (porcine gelatine or whey protein concentrate) and three concentrations of glycerol (2% w/w, 2.5% w/w, and 3% w/w) on the mechanical and thermo-sealing properties of edible films. Tensile strength, deformation at break, firmness, Young’s modulus, heat-sealing capacity, and the necessary energy to cause an adhesive failure were studied. Results showed that the different combinations of starch, glycerol, and proteins allow the formation of materials with a wide range of characteristics. All the tested films showed potential to be used as food containers, those obtained from tapioca starch and gelatin were stronger, firmer, and less deformable than tapioca starch: whey protein films. More studies should be carried out in order to determine their application, such as water affinity, compatibility with food matrices or other substances, and microbiological stability.
Similar content being viewed by others

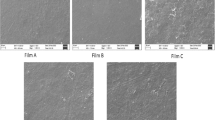
Explore related subjects
Discover the latest articles, news and stories from top researchers in related subjects.Avoid common mistakes on your manuscript.
Introduction
Petroleum-derived polymers are widely used for food-packaging fabrication, due to their low price, availability, easy heat sealability, versatility and resistance to physical damage (Otoni et al., 2017). Most of the manufactured plastic is destined to the food industry, where more than 50% of the containers used only once before disposal (Zhao et al., 2020). Therefore, for the last two decades, the packaging industry has been searching for cleaner alternatives to ensure food safety with a reduced environmental impact (Tian & Bilal, 2019). Polysaccharides, lipids and proteins, are being investigated as sources for developing edible materials, which can be potentially used as food packaging. They are compostable, biodegradable, non toxic, and intend to reduce the use of petroleum derived plastics (Dhumal & Sarkar, 2018). These biopolymers can also be used to develop edible films, which consist in thin cohesive layers of self-supported material that may be applied directly to food surfaces without risks of toxic monomers migration to food products (Díaz-Montes & Castro-Muñoz, 2021).
As edible films are developed, it is important to consider factors that determine their ability to contain and protect food. One of the main functions of packaging is to protect food from the surrounding environment, so its mechanical behavior and the ability to form durable packages are key parameters to be taken into account (Siracusa et al., 2008). The mechanical properties of edible films are affected by their composition and processing factors, for example, proteins tend to assure mechanical stability, and polysaccharides, to control oxygen and other gases' permeability (Murrieta-Martínez et al., 2018).
Starch is an energy-storage polysaccharide produced by plants, consisting of molecules of amylose and amylopectin. Tapioca’s (Manihot esculenta) tuberous root is an important source of starch. Edible films based on tapioca starch are transparent, colorless, tasteless, and flavorless. Nevertheless, films made from this source are brittle, so plasticizers must be used to improve their mechanical characteristics (Hassan et al., 2018; Ulyarti et al., 2020). Glycerol is a hydrophilic, low molecular weight polyol widely used as a plasticizer for starch edible films, as it is considered non-toxic and compatible with that polysaccharide (Basiak et al., 2018). Although glycerol enhances film flexibility, it can decrease film strength. It was reported that blending starch with proteins may improve film mechanical properties, due to their complex structures and binding capacity (Tongdeesoontorn et al., 2012). Animal-derived proteins, mostly obtained as industrial by-products, are promising resources to enhance films’ characteristics. For example, whey protein is a by-product derived from cheese production as a result of casein precipitation and it is composed of alpha and beta lactoglobulin, bovine serum albumin, and milk fat (Murrieta-Martínez et al., 2018). Whey protein films have good mechanical properties, and act as acceptable gas, aromas, and oil barriers. They are limited by their hydrophilicity, needing the use of additives to improve their characteristics (Hassan et al., 2018). Gelatine is a protein obtained as a product of the partial hydrolysis of collagen from animal bones, skin, or connective tissue (Murrieta-Martínez et al., 2018). Films obtained from gelatine are transparent, flexible, and heat-sealable (Liu et al., 2020), but tend to present a high sensibility to water, and low permeability to water vapor (Suderman et al., 2018).
The combination of more than one biopolymer for the formulation of edible films may lead to the creation of materials with improved characteristics compared to those formulated based on a single component (Galus et al., 2020). Wang et al. (2017) reported that gelatine films improved their thickness, transparency, solubility, and mechanical properties as they were combined with corn starch. Similar results were obtained by Ulyarti et al. (2020), showing that the addition of gelatine to tapioca-modified starch diminishes water vapor transmission rate, transparency, and mechanical properties (Pellá et al., 2020). Basiak et al. (2015) showed that adding whey proteins to wheat starch films improved their flexibility and tensile strength.
The aim of this investigation is to study the mechanical and thermo-sealing properties of edible films based on different proportions of tapioca starch and whey protein or gelatine. Based on the results, it will be estimated their potentiality to be applied in food preservation.
Materials and Methods
Materials
For the development of edible films, tapioca starch (TS), whey protein concentrate (WPC), porcine gelatine (PG), and glycerol (G) were used. Tapioca starch was provided by Industrias del Maíz (Argentina). Whey protein concentrate, Lacprodan 80, was provided by Arla Food Ingredients S.A. (Argentina). Porcine gelatine (220 Bloom) was provided by Gelnex Indústria e Comércio Ltda (Brazil). Glycerol was provided by Laboratorios Cicarelli (Argentina).
Film Preparation
Tapioca starch: whey protein concentrate (TS:WPC) and tapioca starch: porcine gelatine (TS:PG) edible films were prepared as described in Tables 1 and 2, respectively. Glycerol concentrations ranged between 2 and 2.5% w/w, tapioca starch between 60 and 80% of total solids, and protein between 20 and 40% w/w of total solids. Water was added up to complete 100% w/w. Films were elaborated by the casting method (Ollé Resa et al., 2014). Combinations with higher and lower proportions of starch: protein than those informed were also elaborated in preliminary experiments, but they did not form self-supporting films (data not shown).
Corresponding protein and tapioca starch were mixed with distilled water and glycerol, in constant agitation. Heat was applied at a constant rate of 5.0 °C/min, until reaching starch gelatinization temperature (~80 °C) to form the film-forming solution (FFS). Then, it was cooled at room temperature up to 40 °C, and 8 g were poured onto 7-cm diameter circular silicone molds. Solvent was evaporated at 37 °C for 48 h in a convection chamber. Once films dried, they were peeled off from the molds and, prior to the physico-chemical determinations, were stabilized at 28 °C in desiccators containing saturated solution of sodium bromide (Water activity, aw = 0.575) for 7 days.
Film Properties
Mechanical Properties
Mechanical properties of interest were tensile strength, deformation at break, firmness, and Young’s modulus. Tensile strength is defined as the maximum stress applied to films during mechanical tests before breakpoint. Deformation at break describes the maximum deformation reached by films before breakpoint, and may be used as an indicator of their flexibility. Firmness is calculated as the relationship between tensile strength and deformation and break. Young’s modulus is a good indicator of the elasticity of films. It is obtained from the initial linear slope of the tensile strength-deformation at break curves.
In order to determine the film mechanical properties, uniaxial tension tests were performed. Ten rectangular samples of films for each formulation were cut, sized 6 mm in width and 40 mm in length. A digital micrometer (Mitutoyo, Japan) was used to measure film thickness in triplicate. To determine the mechanical properties of films, tensile tests were performed, using an Instron Universal Testing Machine (Instron Corp., Canton, MA, USA). Film samples were stretched uniaxially at a constant rate of 0.8 mm/s until breakpoint, setting the initial separation between grips at 20 mm. Stress-strain curves were recorded, stress at break, strain at break, and firmness were calculated from recorded curves, as follows:
Young’s modulus was calculated as the initial linear slope of stress-strain curves.
Heat-Sealing Capacity
Heat-sealing capacity is an indicator of sealing quality. Testing was based on procedures described by López et al. (2011) and ASTM F88-00 (2001) standard method. Four samples were prepared for each film, cutting two rectangles 25 mm wide and 50 mm long. They were sealed together by one of their extremes, using an impulse-wire thermosealer (FS-400, Hualian, China), applying heat for 10 s. Seals were cooled and stabilized prior to further determinations.
Films thickness was measured in triplicate. Measures of heat-sealing capacity were performed via tensile tests, using an Instron Universal Testing Machine. Samples were attached to the grips, placing the seal equidistantly and perpendicular to the movement direction. Initial separation of grips was set at 25 mm. They were stretched uniaxially at a constant rate of 0.8 mm/s until the seal was peeled apart.
As described by ASTM standard method F88-00 (2001) for adhesive failures, media heat sealer resistance (force/deformation, expressed in MPa) and necessary energy (J/m) to peel the seal were calculated.
Statistical Analysis of Data
Data was analyzed using software Infostat version 2018 (Universidad Nacional de Córdoba, Argentina). For each parameter, differences between samples were analyzed through a two-way ANOVA (α: 0.05). A Tukey HSD (honest significance difference) test was performed in two ways:
-
Between different proportions of biomolecules (TS:Protein)
-
Between different glycerol concentrations.
Results were reported based on their means and standard deviation. Graphical representation of data was performed using GraphPad Prism version 8.0.0 for Windows (GraphPad Software, San Diego, CA, USA, www.graphpad.com).
Results and Discussion
Film Characterization
Mechanical Properties
Obtained results for tensile strength and deformation at break are summarized in Table 3. Firmness and Young’s modulus are shown in Figs. 1 and 2, for all the tested edible films. The mean and standard deviation of films’ thickness are presented in Table 4.
Effect of TS:Protein ratio and glycerol concentration on firmness (MPa). Different lowercase letters indicate statistically significant differences (p < 0.05) between composite films at the same glycerol level and different TS:Protein ratio. Different capital letters indicate statistically significant differences (p < 0.05) between composite films at the same TS:Protein and different glycerol level
Effect of TS:Protein ratio and glycerol concentration on Young modulus. Different lowercase letters indicate statistically significant differences (p < 0.05) between composite films at the same glycerol level and different TS:Protein ratio. Different capital letters indicate statistically significant differences (p < 0.05) between composite films at the same TS:Protein and different glycerol level
Tensile Strength
For TS:WPC films, tensile strength was affected by starch:protein ratio only at 2% glycerol. For films containing 2.5% and 3% glycerol levels, no differences were observed (Table 3). Films formulated with 60:40 TS:WPC ratio at 2% glycerol concentration showed the highest tensile strength. Chollakup et al. (2020) found similar results for wheat starch and whey protein isolate films: more rigid and less flexible structures were those found in films containing lower starch concentrations. This behavior may be explained by the strong inter- and intramolecular bonds and interactions associated to functional groups on protein side chains. Glycerol level had an effect on the parameter: for the 70:30 ratio, as glycerol concentration increased, so did tensile strength. On the other hand, for 80:20 ratio, glycerol concentration did not affect tensile strength. Unless higher glycerol concentration is expected to have an impact on tensile strength, previous studies have demonstrated that the use of low glycerol concentrations in starch films may have an anti plasticizing effect (Chang et al., 2006; Mali et al., 2008), which can be possibly attributed to the strong hydrogen bonds formed between the polyol and starch (van der Sman, 2019). On the contrary, when films were formulated at 60:40, the increase in glycerol content reduced the tensile strength.
TS:PG films were influenced by starch: protein ratio on tensile strength, for 2% and 3% glycerol levels. Tensile strength was higher for 70:30 TS:PG at 2% glycerol concentration followed by 80:20 TS:PG ratio, 2% glycerol. Tongdeesoontorn et al. (2012) reported a similar behavior for composite films based on tapioca starch and gelatine. They found that when gelatine was at 30% concentration, tensile strength showed the highest value, described as its “critical value.” As gelatine concentration was increased to 40%, tensile strength dropped slightly. The observed critical value may be attributed to the highest stability and integrity of films. The decrease of tensile strength observed as starch concentration was increased beyond the critical value may be associated to the formation of starch intramolecular hydrogen bonds, instead of the formation of intermolecular ones. Glycerol level also affected film behavior. For every TS:PG ratio the stronger films were those formulated with less glycerol concentration. The higher tensile strength values of TS:PG films were nearly 20 times superior to those observed in TS:WPC (1.37 MPa for TS:WPC versus 20.56 MPa for TS:PG).
Deformation at Break
For TS:WPC films, starch:protein ratio affected deformation at break: more flexible films were those formulated at 70:30 TS:WPC ratio, at every glycerol level. Within the same glycerol content, films with more WPC concentration were more deformable. It has been observed the plasticizing effect of different sugars, as lactose, when incorporated in chitosan edible films (Ra’il et al., 2022) and other types of disaccharides on starch edible films (Ploypetchara & Gohtani, 2018). Other authors (Hammam, 2019; Kandasamy et al., 2021) have also reported that the increase in WPC concentration increases the deformation at break of films based on WPC. These authors attribute this plasticizing effect to the increase in the lactose contained in the WPC. It was also observed that within 80:20 and 70:30 TS:WPC ratio, flexibility rose as glycerol content was increased; however, for 60:40 TS:WPC ratio, glycerol content did not increase the deformation at break, being always under 1. For TS:PG films, TS:PG ratio did not have an effect for 2.5% glycerol level. For 2% and 3% glycerol levels, higher gelatine concentrations led to more deformable films. It is remarkable that at 70:30 TS:PG ratio, films formulated with 2% glycerol presented the least deformation of all the studied films, in coincidence with a high tensile strength. Within 80:20 and 70:30 TS:PG ratios, higher glycerol concentration determined more flexibility. This was observed as a higher deformation at break for films containing higher glycerol concentration. Soliman and Furuta (2014) attributed this behavior to glycerol, as it is a small molecule, it easily entangles among polymeric chains, debilitating the interactions between starch and gelatine. These authors demonstrated that when blending gelatine with glycerol in composite film formulations, gelatine may act as a “co-plasticizer,” enhancing films flexibility and reducing their fragility. These results may also explain the behavior observed for 60:40 TS:PG ratio, in which the highest deformation values were found for 2% and 3% glycerol concentration.
Firmness
Films with higher firmness values are those stronger and less deformable. Two different behaviors were observed for TS:WPC films, with 2.5% and 3% glycerol levels, higher firmness was observed for 70:30 starch:protein ratio (Fig. 1). On the contrary, with the 2% glycerol level, less firmness was observed for 70:30. Glycerol affected firmness for 80:20 and 60:40 TS:WPC ratios, generating firmer films as glycerol concentration was lower (Fig. 1(1) and (3)). On the other hand, the highest firmness for TS:PG films was observed at every glycerol level within the 70:30 TS:PG ratio (Fig. 1(5)), being remarkably superior at 2% glycerol concentration (150 MPa). This behavior may be attributed to its high tensile strength and an extreme low deformation at break, associated with strong interactions between starch and gelatine. Firmness dropped for every TS:PG ratio as glycerol content increased. In general, higher glycerol concentrations lead to films with less firmness. This behavior may be explained as a consequence of the plasticizer activity of glycerol, it helps to retain moisture in the film, avoiding the formation of pores on the surface of the material. This effect is increased with glycerol concentration, as the interaction between polymeric chains is decreased, improving starch chains movement (Ben et al., 2022).
Young’s Modulus
Young modulus is an indicator of the stiffness of films (Galus & Kadzińska, 2016). For TS:WPC films, both TS:WPC ratio and glycerol concentration affected Young’s modulus values (Fig. 2(1)–(3)). Within 80:20 and 60:40 ratios, the stiffness of films decreased as glycerol concentration increased (Fig. 2(1) and (3)). For 70:30 ratio, higher Young’s modulus values were observed for 2% and 2.5% gly. Within every glycerol concentration, 70:30 TS:WPC ratio always showed the highest values (Fig. 2(2)). These results are mostly in accordance with firmness, and with values reported by Cortés-Rodríguez et al. (2020) for tapioca starch, whey protein, and bees wax film, in which Young’s modulus is also affected by starch:protein ratio. Young’s modulus values obtained for TS:PG films were notably higher than those obtained for TS:WPC films (Fig. 2(4)–(6)). Glycerol concentration and TS:PG ratio also affected stiffness, being the films formulated with less glycerol concentrations stiffer than the ones with higher concentrations, demonstrating the lowest Young’s modulus values. A similar trend was found by Saberi et al. (2016) for pea starch edible films plasticized with different glycerol concentrations. These authors attribute this behavior to the anti-plasticizing effect of glycerol and water for films, when analyzing films at 52.9% relative humidity. As observed for firmness, 70:30 TS:PG showed remarkably higher values at every glycerol level than the rest of formulations (400 MPa for 2% glycerol).
Heat-Sealing Capacity
Heat-sealing is widely used in the food packaging industry due to its simplicity and reduced cost. To avoid package failure and content spillage, acceptable thermo-sealability and seal strength are important characteristics of films (Liu et al., 2020).
Media heat resistance can be defined as the mean of required forces per film width to separate two film layers that were previously thermosealed. Necessary energy to cause adhesive failure in films was calculated as the area under the force vs. extension curve (ASTM F88-00, 2001). Adhesive failures were observed in all tested films.
Necessary Energy to Cause an Adhesive Failure
Necessary energy to cause the failure is presented in Fig. 3.
Effect of TS:Protein ratio and glycerol concentration on the required energy to cause an adhesive failure (J/m). Different lowercase letters indicate statistically significant differences (p < 0.05) between composite films at the same glycerol level and different TS:Protein ratio. Different capital letters indicate statistically significant differences (p < 0.05) between composite films at the same TS:Protein and different glycerol level
Starch:protein ratio influenced energy to cause adhesive failure. For TS:WPC films, as WPC content increased, less energy was required to open the seals (Fig. 3(1)–(3)). Although extensive research has been carried out on this behavior, no studies were found regarding WPC concentration effect on energy. On the contrary, for TS:PG films (Fig. 3(4), and (6)), higher gelatine concentration meant more necessary energy required, being 1.28 J/m for 60:40 TS:PG–2% glycerol. Said et al. (2021) reviewed studies describing edible films elaborated with gelatin-based films as food packaging, and described a similar trend. As gelatine concentration is diminished, due to the addition of other materials such as starch, so does the melting point of the films, resulting in a diminution of the required energy to disrupt the seal. Only for this TS:PG ratio, glycerol had an effect on required energy, showing lower values as glycerol concentration increased (Fig. 3(6)).
Media Heat Resistance
Media heat resistance was affected by starch:protein ratio for all formulations tested, as presented in Fig. 4.
Effect of TS:Protein ratio and glycerol concentration on media heat resistance (MPa). Different lowercase letters indicate statistically significant differences (p < 0.05) between composite films at the same glycerol level and different TS:Protein ratio. Different capital letters indicate statistically significant differences (p < 0.05) between composite films at the same TS:Protein and different glycerol level
For TS:WPC films, maximum media heat resistance was obtained for 80:20 TS:WPC films, ranging between 0.22 and 0.24 MPa, regardless of glycerol concentration (Fig. 4 (1)). These values nearly doubled media heat resistances calculated for 70:30 and 60:40 TS:WPC films (Fig. 4(2) and (3)). The effect of glycerol concentration was only observed within 70:30 TS:WPC films: Those formulated with 2% and 3% glycerol concentration exhibited higher media heat resistance than 2.5% glycerol concentration.
For TS:PG films, media heat resistance was influenced by gelatine concentration, as highest values were found for 70:30 TS:PG films (Fig. 4(5)). A similar trend was observed for mechanical properties: at this TS:PG ratio, films tended to be firmer, and with higher tensile strength than the rest of the formulations tested. Glycerol content also affected media heat resistance, as more heat-resistant films were those containing less amount of plasticizer (1.80 MPa for 70:30 TS:PG–2% glycerol films), showing the best zip quality among all films tested (Fig. 4(4), (5), and (6)).
Gelatine films had better seal quality than those formulated with WPC. As it was reported by other authors, gelatine tends to present a better heat sealability than other biopolymers, due to its chemical characteristics (Abedinia et al., 2018). When blending gelatine with soybean soluble polysaccharides, seals were stronger as gelatine proportion increased (Liu et al., 2020), this may be attributed to the development of a more homogeneous and continuous matrix, leading to the formation of triple helix junctions, that improve the formation of a new homogeneous layer.
Potential Applications
In order to extend food shelf life, edible composite films are thought to protect food from the environment. Trying to preserve the desired attributes which strongly depend on the application and the characteristics of the food to be protected by the film (Otoni et al., 2017).
Acceptable mechanical properties are required when formulating edible films. Materials which are brittle, weak, and with little flexibility have limited usefulness when it comes to production, handling or use, as package integrity is needed to protect its content from the environment (Kocira et al., 2021). It was observed that proteins play a key role on the strength and flexibility of films, as after denaturation, they interact with surrounding molecules through different kind of linkages, forming films with strength, cohesiveness, and viscoelasticity (Ribeiro et al., 2021). In the literature, there are many studies regarding the combination of starch and proteins for the formation of edible films. Pérez et al. (2021) demonstrated that the addition of zein to tapioca starch in film formulations led to the formation of materials with improved physico-chemical properties. It was also reported that quinoa protein enhanced the mechanical properties of quinoa starch edible films (Wang & Zhu, 2015). Despite the mentioned proteins are structurally different than those analyzed in this study, it is interesting to note that proteins in general may have positive effects on films’ strength and flexibility as being combined with starch, as it was observed in our results. Among the studied films, the ones that combined strength with flexibility were those formulated with 70:30 TS:WPC 3% glycerol, 80:20 TS:PG 2%, and 2.5% glycerol.
When films are intended to be used as pouches or sachets, their ability to form high-quality thermoseals is important, as it prevents the opening and food leak from the package. In this case, a high heat seal resistance is needed (Suh et al., 2020). TS:PG films showed substantially higher seal quality than TS:WPC films, as the highest resistance and energy were measured for 70:30 TS:PG 2% glycerol films.
As edible films were studied, the combination of the described parameters was observed to define potential applications in food matrices. As mechanical parameters define package integrity, it was taken into account how strong and flexible films were, prioritizing films with higher tensile strength and deformation at break. Films with the ability to form strong but deformable materials, with high sealing resistance may be applied to the elaboration of pouches, as 80:20 TS:WPC 2.5% glycerol, 70:30 TS:WPC 3% glycerol, 70:30 TS:PG 2.5% glycerol, and 70:30 TS:PG 3% glycerol. Films as 80:20 TS:PG 2% glycerol show good mechanical properties, but poor sealing ability, so they may be applied to other packaging uses that need no heat-sealing, such as wrappings (for candy or baked goods, for example), or to be used as sliced food separators, which are commonly used to avoid the adhesion of sliced cheese or cold meats, or even ready to cook dough slices.
There is no research that studies the effect of adding proteins such as gelatin and WPC to edible films based on tapioca starch, even less, and the study of different proportions of these proteins. Our results may represent a starting point on the research of the extensive scope of possible applications that these edible films may have only by altering their proportions.
Conclusions
Edible composite films formulated with three ratios of TS:PG and TS:WPC and three levels of glycerol as plasticizer were studied, testing their tensile strength, deformation at break, firmness, Young’s modulus, and resistance of thermoseals. Complex interactions between TS, glycerol, and proteins led to the formation of materials with a wide range of characteristics. TS:PG films were stronger and firmer, slightly less flexible than TS:WPC films. Five of the studied formulations showed interesting mechanical and heat-sealing attributes: 80:20 TS:WPC 2% glycerol, 70:30 TS:WPC 3% glycerol, 80:20 TS:PG 2.5% glycerol, and 70:30 TS:PG 3% glycerol and 80:20 TS:PG 2% glycerol. These might be used to protect food that requires strong but flexible packages.
In the future, it is interesting to evaluate other characteristics of the films developed in this research. Other important attributes should be taken into account for their application, such as their affinity with water, ability to support other components such as natural antimicrobials, microbiological stability, and compatibility with different foods.
Data Availability
All data generated or analyzed during this study are included in this published article.
References
Abedinia, A., Ariffin, F., Huda, N., & Mohammadi Nafchi, A. (2018). Preparation and characterization of a novel biocomposite based on duck feet gelatin as alternative to bovine gelatin. International Journal of Biological Macromolecules, 109, 855–862. https://doi.org/10.1016/j.ijbiomac.2017.11.051
American Society for Testing and Materials – ASTM. (2001). Standard test method for seal strength of flexible barrier materials – ASTM F88–00. ASTM Standards on Disc (vol. 15.09, p. 6). Philadelphia: ASTM.
Basiak, E., Galus, S., & Lenart, A. (2015). Characterisation of composite edible films based on wheat starch and whey-protein isolate. International Journal of Food Science and Technology, 50(2), 372–380. https://doi.org/10.1111/ijfs.12628
Basiak, E., Lenart, A., & Debeaufort, F. (2018). How glycerol and water contents affect the structural and functional properties of starch-based edible films. Polymers, 10(4), 412. https://doi.org/10.3390/POLYM10040412
Ben, Z. Y., & Samsudin, H.,; Yhaya, M. F. (2022). Glycerol: Its properties, polymer synthesis, and applications in starch based films. European Polymer Journal, 175, 111377. https://doi.org/10.1016/J.EURPOLYMJ.2022.111377
Chang, Y. P., Abd Karim, A., & Seow, C. C. (2006). Interactive plasticizing–antiplasticizing effects of water and glycerol on the tensile properties of tapioca starch films. Food Hydrocolloids, 20(1), 1–8. https://doi.org/10.1016/J.FOODHYD.2005.02.004
Chollakup, R., Pongburoos, S., Boonsong, W., Khanoonkon, N., Kongsin, K., Sothornvit, R., Sukyai, P., Sukatta, U., & Harnkarnsujarit, N. (2020). Antioxidant and antibacterial activities of cassava starch and whey protein blend films containing rambutan peel extract and cinnamon oil for active packaging. LWT, 130, 109573. https://doi.org/10.1016/J.LWT.2020.109573
Cortés-Rodríguez, M., Villegas-Yépez, C., Gil González, J. H., Rodríguez, P. E., & Ortega-Toro, R. (2020). Development and evaluation of edible films based on cassava starch, whey protein, and bees wax. Heliyon, 6(9), e04884. https://doi.org/10.1016/j.heliyon.2020.e04884
Dhumal, C. V., & Sarkar, P. (2018). Composite edible films and coatings from food-grade biopolymers. Journal of Food Science and Technology, 55(11), 4369–4383. https://doi.org/10.1007/S13197-018-3402-9
Díaz-Montes, E., & Castro-Muñoz, R. (2021). Edible films and coatings as food-quality preservers: an overview. Foods, 10(2), 249. https://doi.org/10.3390/foods10020249
Galus, S., & Kadzińska, J. (2016). Moisture sensitivity, optical, mechanical and structural properties of whey protein-based edible films incorporated with rapeseed oil. Food Technology and Biotechnology, 54(1), 78. https://doi.org/10.17113/FTB.54.01.16.3889
Galus, S., Kibar, E. A. A., Gniewosz, M., & Kraśniewska, K. (2020). Novel materials in the preparation of edible films and coatings—a review. Coatings, 10(7), 674. https://doi.org/10.3390/COATINGS10070674
GraphPad Prism version 8.0.0 for Windows. GraphPad Software, San Diego, California USA. Retrieved November 27, 2018, from www.graphpad.com
Hammam, A. R. A. (2019). Technological, applications, and characteristics of edible films and coatings: A review. SN Applied Sciences, 1(6), 1–11. https://doi.org/10.1007/S42452-019-0660-8/FIGURES/2
Hassan, B., Chatha, S. A. S., Hussain, A. I., Zia, K. M., & Akhtar, N. (2018). Recent advances on polysaccharides, lipids and protein based edible films and coatings: a review. International Journal of Biological Macromolecules, 109, 1095–1107. https://doi.org/10.1016/j.ijbiomac.2017.11.097. Elsevier B.V.
InfoStat. (2018). InfoStat versión 2008. FCA, Universidad Nacional de Córdoba, Argentina.
Kandasamy, S., Yoo, J., Yun, J., Kang, H.-B., Seol, K.-H., Kim, H.-W., & Ham, J.-S. (2021). Application of whey protein-based edible films and coatings in food industries: an updated overview. Coatings, 11(9), 1056. https://doi.org/10.3390/coatings11091056
Kocira, A., Kozłowicz, K., Panasiewicz, K., Staniak, M., Szpunar-Krok, E., & Hortyńska, P. (2021). Polysaccharides as edible films and coatings: characteristics and influence on fruit and vegetable quality–a review. Agronomy, 11(5), 813. https://doi.org/10.3390/agronomy11050813
Liu, C., Huang, J., Zheng, X., Liu, S., Lu, K., Tang, K., & Liu, J. (2020). Heat sealable soluble soybean polysaccharide/gelatin blend edible films for food packaging applications. Food Packaging and Shelf Life, 24, 100485. https://doi.org/10.1016/J.FPSL.2020.100485
López, O. V., Lecot, C. J., Zaritzky, N. E., & García, M. A. (2011). Biodegradable packages development from starch based heat sealable films. Journal of Food Engineering, 105(2), 254–263. https://doi.org/10.1016/J.JFOODENG.2011.02.029
Mali, S., Grossmann, M. V. E., Garcia, M. A., Martino, M. N., & Zaritzky, N. E. (2008). Antiplasticizing effect of glycerol and sorbitol on the properties of cassava starch films. Brazilian Journal Of Food Technology, 11(3), 194–200. https://doi.org/10.5433/1679-0359.2007v28n1p79
Murrieta-Martínez, C. L., Soto-Valdez, H., Pacheco-Aguilar, R., Torres-Arreola, W., Rodríguez-Felix, F., & Márquez Ríos, E. (2018). Edible protein films: Sources and behavior. Packaging Technology and Science, 31(3), 113–122. https://doi.org/10.1002/pts.2360
Ollé Resa, C. P., Gerschenson, L. N., & Jagus, R. J. (2014). Natamycin and nisin supported on starch edible films for controlling mixed culture growth on model systems and Port Salut cheese. Food Control, 44, 146–151. https://doi.org/10.1016/J.FOODCONT.2014.03.054
Otoni, C. G., Avena-Bustillos, R. J., Azeredo, H. M. C., Lorevice, M. V., Moura, M. R., Mattoso, L. H. C., & McHugh, T. H. (2017). Recent advances on edible films based on fruits and vegetables–a review. Comprehensive Reviews in Food Science and Food Safety, 16(5), 1151–1169. https://doi.org/10.1111/1541-4337.12281
Pellá, M. C. G., Silva, O. A., Pellá, M. G., Beneton, A. G., Caetano, J., Simões, M. R., & Dragunski, D. C. (2020). Effect of gelatin and casein additions on starch edible biodegradable films for fruit surface coating. Food Chemistry, 309, 125764. https://doi.org/10.1016/j.foodchem.2019.125764
Pérez, P. F., Ollé Resa, C. P., Gerschenson, L. N., et al. (2021). Addition of zein for the improvement of physicochemical properties of antimicrobial tapioca starch edible film. Food Bioprocess Technology, 14, 262–271. https://doi.org/10.1007/s11947-020-02565-z
Ploypetchara, T., & Gohtani, S. (2018). Effect of sugar on starch edible film properties: Plasticized effect. Journal of Food Science and Technology, 55(9), 3757–3766. https://doi.org/10.1007/s13197-018-3307-7
Ra’il, N. H., Saputro, A. G., Ataollahi, N., Ahmad, A., & Mobarak, N. N. (2022). The role of disaccharides as a plasticizer in improving the interaction between chitosan chain based solid polymer electrolytes (SPEs). New Journal of Chemistry, 46(8), 3844–3855. https://doi.org/10.1039/d1nj05735a
Ribeiro, A. M., Estevinho, B. N., & Rocha, F. (2021). Preparation and incorporation of functional ingredients in edible films and coatings. Food Bioprocess Technology, 14, 209–231. https://doi.org/10.1007/s11947-020-02528-4
Saberi, B., Vuong, Q., Chockchaisawasdee, S., Golding, J., Scarlett, C., & Stathopoulos, C. (2016). Mechanical and physical properties of pea starch edible films in the presence of glycerol: properties of pea starch edible films. Journal of Food Processing and Preservation, 40, 1339–1351. https://doi.org/10.1111/jfpp.12719
Said, N., Nazlin, A., Howell, K., & Sarbon, N. M. (2021). A review on potential use of gelatin-based film as active and smart biodegradable films for food packaging application. Food Reviews International. https://doi.org/10.1080/87559129.2021.1929298
Siracusa, V., Rocculi, P., Romani, S., & Rosa, M. D. (2008). Biodegradable polymers for food packaging: a review. Trends in Food Science and Technology, 19(12), 634–643. https://doi.org/10.1016/j.tifs.2008.07.003
Soliman, E. A., & Furuta, M. (2014). Influence of phase behavior and miscibility on mechanical, thermal and micro-structure of soluble starch-gelatin thermoplastic biodegradable blend films. Food and Nutrition Sciences, 05(11), 1040–1055. https://doi.org/10.4236/FNS.2014.511115
Suderman, N., Isa, M. I. N., & Sarbon, N. M. (2018). Characterization on the mechanical and physical properties of chicken skin gelatin films in comparison to mammalian gelatin films. IOP Conference Series: Materials Science and Engineering, 440(1), 012033. https://doi.org/10.1088/1757-899X/440/1/012033
Suh, J. H., Ock, S. Y., Park, G. D., Lee, M. H., & Park, H. J. (2020). Effect of moisture content on the heat-sealing property of starch films from different botanical sources. Polymer Testing, 89, 106612. https://doi.org/10.1016/J.POLYMERTESTING.2020.106612
Tian, K., & Bilal, M. (2019). Research progress of biodegradable materials in reducing environmental pollution. Abatement of environmental pollutants: trends and strategies (pp. 313–330). Elsevier. https://doi.org/10.1016/B978-0-12-818095-2.00015-1
Tongdeesoontorn, W., Mauer, L. J., Wongruong, S., Sriburi, P., & Rachtanapun, P. (2012). Mechanical and physical properties of cassava starch-gelatin composite films. International Journal of Polymeric Materials and Polymeric Biomaterials, 61(10), 778–792. https://doi.org/10.1080/00914037.2011.610049
Ulyarti, U., Nazarudin, N., Surhaini, Lisani, Ramadon, R., & Lumbanraja, P. (2020). Cassava starch edible film with addition of gelatin or modified cassava starch. IOP Conference Series: Earth and Environmental Science, 515(1), 012030. https://doi.org/10.1088/1755-1315/515/1/012030
van der Sman, R. G. M. (2019). Phase separation, antiplasticization and moisture sorption in ternary systems containing polysaccharides and polyols. Food Hydrocolloids, 87, 360–370. https://doi.org/10.1016/J.FOODHYD.2018.07.051
Wang, K., Wang, W., Ye, R., Xiao, J., Liu, Y., Ding, J., Zhang, S., & Liu, A. (2017). Mechanical and barrier properties of maize starch-gelatin composite films: effects of amylose content. Journal of the Science of Food and Agriculture, 97(11), 3613–3622. https://doi.org/10.1002/JSFA.8220
Wang, S., & Zhu, F. (2015). Formulation and quality attributes of quinoa food products. Food and Bioprocess Technology, 9, 49–68. https://doi.org/10.1007/s11947-015-1584-y
Zhao, X., Cornish, K., & Vodovotz, Y. (2020). Narrowing the gap for bioplastic use in food packaging: an update. Environmental Science & Technology, 54(8), 4712–4732. https://doi.org/10.1021/ACS.EST.9B03755
Acknowledgements
The authors also thank Arla Foods S.A. (Argentina).
Funding
This study was financially supported by the University of Buenos Aires (UBACyT 20020170100229BA, 2018–2021 and 20020170100063BA, 2018–2021) and by the National Agency of Scientific and Technical Research (PICT2019-01551, 2021–2024 and MINCyT. PICT-2017- 1146. 2019–2022).
Author information
Authors and Affiliations
Contributions
Yanina Izzi: investigation, statistical analysis of data, writing, and writing–review and editing; Lía Gerschenson: experimental design, resources, and writing–review and editing; Rosa Jagus: experimental design, resources, and writing–review and editing; Carolina Ollé: experimental design, supervision, and writing–review and editing.
Corresponding author
Ethics declarations
Conflict of Interest
The authors declare no competing interests.
Additional information
Publisher's Note
Springer Nature remains neutral with regard to jurisdictional claims in published maps and institutional affiliations.
Rights and permissions
Springer Nature or its licensor (e.g. a society or other partner) holds exclusive rights to this article under a publishing agreement with the author(s) or other rightsholder(s); author self-archiving of the accepted manuscript version of this article is solely governed by the terms of such publishing agreement and applicable law.
About this article
Cite this article
Izzi, Y.S., Gerschenson, L.N., Jagus, R.J. et al. Edible Films Based on Tapioca Starch and WPC or Gelatine Plasticized with Glycerol: Potential Food Applications Based on Their Mechanical and Heat-Sealing Properties. Food Bioprocess Technol 16, 2559–2569 (2023). https://doi.org/10.1007/s11947-023-03089-y
Received:
Accepted:
Published:
Issue Date:
DOI: https://doi.org/10.1007/s11947-023-03089-y