Abstract
We developed a micropropagation protocol for Cleome gynandra, a C4 model plant with medicinal importance. Surface-sterilized nodal segments obtained from 1 to 2-month-old field grown plant were used as explants for culture establishment and plant regeneration. Multiple shoots differentiated through bud breaking on Murashige and Skoog (MS) medium with different concentrations of benzyladenine (BA) and kinetin (Kin). The optimum shoot differentiation occurred on medium with 1.5 mg l−1 BA. Out of various concentrations and combinations of cytokinins and auxins, MS medium containing 0.5 mg l−1 BA and 0.1 mg l−1 IAA (indole-3-acetic acid) was found best for shoot multiplication. However, the differentiated shoots exhibited hyperhydration, leaf curling and early leaf fall during subculturing. To overcome these problems, regenerated shoots were transferred to the modified MS medium with reduced nitrates (825 mg l−1 NH4NO3 and 950 mg l−1 KNO3) and 100 mg l−1 (NH4)2SO4. The micropropagated shoots were rooted (i) in vitro on one-fourth strength of MS salts with 0.25 mg l−1 each of IBA (indole-3 butyric acid) and NOA (2-naphthoxyacetic acid) + 100 mg l−1 activated charcoal, and (ii) ex vitro, by treating the shoot base(s) with 200 mg l−1 of IBA for 3 min and transferred to soilrite moistened with one-fourth strength of MS macro salts in culture bottles. The plants were hardened in the greenhouse with 85 % survival rate. Micromorphological studies of the plants were conducted during hardening with reference to development and changes in vein spacing, glandular trichome and stomata. In comparison to leaves under in vitro condition, higher density of veins and glandular trichomes was observed in the leaves of hardened plants. In addition, stomata became functional during hardening which were non-functional under in vitro condition.
Similar content being viewed by others
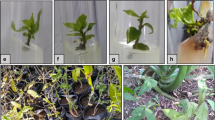
Avoid common mistakes on your manuscript.
Introduction
Global warming and increased level of green house gases (GHG) are major threats to environment and will impose constraints on biomass production on earth. These issues are critical for arid and semi arid parts of the world where plant productivities are constrained due to stresses imposed by biotic and abiotic factors (Shekhawat et al. 2012). It has been suggested that drought hardy and stress-resistant plants will survive to support life (Walther et al. 2002). Plant species with abilities to remain productive even at elevated levels of carbon dioxide are going to be important in the era of climate change and global warming. Plant species with C4 photosynthesis have genetic, biochemical, physiological and ecological traits that make them suitable for biomass production (Sage 2003; Sage and Kubien 2007). Genetic manipulation for more efficient crop production with C4 characters has been suggested and using these approaches more efficient crops like rice can be supplemented with C4 characters to increase yield (Brown et al. 2005; Hibberd et al. 2008). Nevertheless, understanding genetic basis of C4 plants using in vitro and in vivo approaches can be useful.
Cleome gynandra belongs to the family Cleomaceae formerly Capparaceae. The plant is distinguished from other coexisting species of the genus by its unique flower with gynandrophore. This species bears many characters which make it suitable for research. It is a C4 plant and exhibit Kranz anatomy i.e. more veins, larger bundle sheath cells and mesophyll cells form a ring around bundle sheath cells (Sankhla et al. 1975; Muhaidat et al. 2007). C. gynandra has the potential to be a C4 model system because it is phylogenetic near relative of Arabidopsis thaliana, the well-known C3 model plant and it has potential advantages suitable for a model system such as small size, rapid growth, small life cycle, self fertility and produces much amount of seeds. It has similarities with Arabidopsis, so the vast molecular data available for Arabidopsis can be utilized for Cleome (Brown et al. 2005; Newell et al. 2010; Koteyeva et al. 2011). The genus Cleome contains phylogenetic progression from C3 to C4 photosynthesis, so it offers the potential to understand how C4 photosynthetic pathway has evolved from C3 plants (Marshall et al. 2007).
Cleome gynandra is also well known for medicinal importance. The leaves are source of vitamin A and minerals (calcium and iron) and it also has insecticidal and repellent characteristics (Chweya and Mnzava 1997). In India, it is traditionally used in ear ache, skin disease and gastrointestinal disorders (Mishra et al. 2011; Aparadh et al. 2012). The leaves are rich source of antioxidants due to presence of flavonoids, vitamin C and the essential metals (Anbazhagi et al. 2009). The leaves of the plant are eaten as vegetable in many parts of sub-Saharan Africa (Chweya and Mnzava 1997).
This plant propagates in nature through seeds but germination rate is very low due to dormancy (Ochuodho and Modi 2007). Till date there is only one report of tissue culture protocol for C. gynandra (Naseem and Jha 1997). In the present investigation, we report for the first time in vitro propagation of this important plant using nodal segments as explants. This protocol is more reproducible in terms of number of shoots, percentage survival of hardened plant and using ex vitro approaches for rooting reduces labour and cost. The micromorphological studies during hardening can be useful to reduce mortality of plantlet during the hardening process which is a major drawback of any tissue culture protocol.
Materials and methods
Plant material and surface sterilization
Source plant was selected from Chopasani village, Jodhpur (Rajasthan, North-West India) during the rainy season i.e. July–September (Fig. 1a). Fresh axillary shoots were excised from 1 to 2-month-old field grown plant. Juvenile nodal segments of 6–8 cm length having 2–3 nodes were used as explants. The explants were initially treated with systemic fungicide 0.1 % (w/v) bavistin (BASF India Limited, Mumbai, India) for 5–7 min followed by surface sterilization with 0.1 % (w/v) solution of HgCl2 (Hi-Media, India) for 3 min under aseptic conditions. These explants were washed with autoclaved distilled water 5–6 times.
In vitro propagation of C. gynandra. a A 1-month-old field grown plant used as source of explant. b Shoot bud induction on MS medium containing 1.5 mg l−1 BA. c Shoot multiplication on MS medium containing 0.5 mg l−1 BA + 0.1 mg l−1 IAA. d Healthy shoots multiplied on MMS 2 medium with 0.5 mg l−1 BA + 0.1 mg l−1 IAA. e In vitro flowering on MMS 2 medium with 0.5 mg l−1 BA + 0.1 mg l−1 IAA. f In vitro rooting of regenerated shoots on one-fourth MS medium containing 0.25 mg l−1 IBA + 0.25 mg l−1 NOA. g Ex vitro rooted plantlets treated with 200 mg l−1 IBA. h Hardened plants in the greenhouse
Nutrient media and culture conditions
MS (Murashige and Skoog 1962) medium with 3 % (w/v) sucrose and 0.8 % (w/v) agar–agar (Bacteriological grade, Qualigens Fine Chemicals, Mumbai, India) was used for culture initiation. The pH of the medium was adjusted to 5.8 ± 0.02 and autoclaved for 15 min at 121 °C and 15 psi. The cultures were maintained under controlled conditions in culture room with 28 ± 2 °C temperature, 14-h photoperiod with a light intensity of 40–50 μmol m−2 s−1 PFD provided by cool white fluorescent lamps (Philips, India) and 60 % RH.
Shoot induction and multiplication
For shoot induction, explants were cultured on MS medium containing BA (0.5–2.5 mg l−1) or Kin (0.5–2.0 mg l−1). Medium without PGRs served as control. The in vitro-regenerated shoots were further multiplied by cutting them into 2–3 cm shoot segments with 1–2 nodes, making a clump of 4–5 shoots and transferring shoot clumps to MS medium with various concentrations of BA (0.25–1.0 mg l−1) or Kin (0.25–1.0 mg l−1) or combination of IAA (0.1–0.5 mg l−1) with BA or BA + Kin. To check hyperhydration, tip burning and early leaf fall, MS medium as well as three MMS media (MMS 1, MMS 2 and MMS 3), which differ from MS medium in nitrogen source i.e. NH4NO3 (1,650, 825 or 412.5 mg l−1), KNO3 (1,900, 950 or 475 mg l−1) and (NH4)2SO4 (50, 100 or 200 mg l−1) were used (Table 1).
Rooting of regenerated shoots
The regenerated shoots (4–5 cm long) were excised carefully and cultured on full, half or one-fourth strengths of MS salts with 100 mg l−1 of activated charcoal and various concentrations of IBA (0.25–1.0 mg l−1) and NOA (0.25–1.0 mg l−1) alone or in combination (0.25 or 0.5 mg l−1 each of IBA and NOA). The cultures were maintained under diffused light (15 μmol m−2 s−1 PFD) at 30 ± 2 °C.
Alternatively experiments were conducted for ex vitro rooting of regenerated shoots. The regenerated shoots were excised carefully and washed thoroughly with sterile water to remove the adhered nutrient agar and then treated for 3 min with 50–250 mg l−1 of IBA and 100–200 mg l−1 of NOA alone or in combination (100 or 200 mg l−1 each of IBA and NOA). The auxin-treated shoots were transferred to bottles containing autoclaved soilrite (a mixture of horticulture grade perlite with Irish peatmoss and exfoliated vermiculite supplied by Kel Perlite, Bangalore, India) moistened with one-fourth strength of MS macro salts.
Acclimatization of micropropagated plantlets
The in vitro rooted plantlets were removed from culture vessels and washed with sterile water to remove adhered nutrient agar. These were carefully transferred to bottles containing autoclaved soilrite and moistened with one-fourth strength of MS macro salts.
The in vitro as well as ex vitro rooted plantlets were placed near the pad section of the greenhouse initially, at 80–90 % RH and 28 ± 2 °C (relatively high humidity and low temperature). The caps of bottles containing rooted shoots were gradually loosened and finally removed. The rooted plantlets were gradually shifted from the pad section of the greenhouse towards the fan section at 60–70 % RH and 32 ± 2 °C (relatively low humidity and high temperature). The hardened plantlets were transferred to the polybags containing mixture of organic manure, garden soil and sand (1:1:1) and acclimatized in the greenhouse.
Micromorphological studies of plants undergoing hardening
We studied the development of veins (vein density and pattern of venation) and glandular trichomes (structure and density) in leaves of plants developed in vitro and those undergoing hardening in the greenhouse. Whole leaves (at least 3–5 from each stage of plantlets) were fixed and cleared in 70 % ethanol (v/v) until chlorophyll was removed, bleached with 5 % (w/v) NaOH, and rinsed three times in water. The leaves were then stained with 1 % safranine (Loba chemie, India) and mounted in water and examined under microscope (Leica DM 3000). To observe the changes in structure and function of developing stomata, epidermal peel was separated manually from leaves under in vitro condition and from hardened plantlet, respectively. Images for all these experiments were captured and analyzed by the software Leica application suite V 3 1.0.
Experimental design and data analysis
The experiments were set up in completely randomized block design and repeated thrice. All the experiments were conducted with a minimum of 20 replicates per treatment. One replicate means one culture vessel. The significance of differences among means was carried out using Duncan’s multiple range tests (DMRT) at P < 0.05. The results are expressed as mean ± SD of three experiments and subjected to one-way analysis of variance (ANOVA) using SPSS v.17 (SPSS, Chicago, USA).
Results and discussion
The plant C. gynandra bears extensive glandular trichomes and harbours a variety of recalcitrant microbes. It was difficult to surface sterilize the explants to establish cultures in vitro. Extensive cleansing and treatment of the explants with bavistin is essential for establishment of aseptic cultures.
Culture establishment
Irrespective of the types and concentrations of cytokinins in MS medium, bud induction from the nodal explants occurred within 4–5 days of inoculation. Shoot bud induction was also observed in MS medium without PGRs, however, response was delayed (7–10 days) in comparison to medium containing PGRs. The MS medium supplemented with 1.5 mg l−1 BA was found most effective for shoot bud induction. On this medium, 3.1 ± 0.73 shoots (each 2.96 ± 0.28 cm long) were differentiated from each node (Fig. 1b; Table 2). At higher concentrations (2.0–2.5 mg l−1) of BA, tip burning of induced shoots and callusing at the base of nodal segment were observed. On medium with lower concentrations (0.5–1.0 mg l−1) of BA, the number of shoots was significantly low. Kin was found less effective as compared to BA for multiple shoot induction. This is contrary to the findings of Naseem and Jha (1997) who reported direct (adventitious) shoot induction from cultured thalamus of this plant on Kin and coconut water. Earlier, superiority of BA over other cytokinins for the shoot multiplication was also reported in other species of this genus like C. spinosa (Albarello et al. 2006) and C. rosea (Simoes et al. 2009).
Shoot multiplication
In vitro-regenerated shoots from nodal segment were multiplied by transferring them to fresh MS medium containing lower levels of cytokinin (BA or Kin) alone or with IAA. Of the various PGRs tested, the combination of 0.5 mg l−1 BA and 0.1 mg l−1 IAA proved to be the best for shoot multiplication. A maximum number of 23.6 ± 0.84 shoots (each 5.4 ± 0.18 cm long) was achieved from each clump within 15 days with this combination (Fig. 1c; Table 3). Culture medium supplemented either with BA or Kin was less effective than the combination of BA and IAA. The number of shoots per inoculum increased significantly (P < 0.05) when IAA was added in BA or BA + Kin containing medium. However, the differentiated shoots were weak and exhibited hyperhydration, leaf curling and early leaf fall during subculturing. To overcome these problems, MMS 2 medium containing 100 mg l−1 of (NH4)2SO4 and half concentration of NH4NO3 (825 mg l−1) and KNO3 (950 mg l−1) was found best among various modified media used. On this medium, shoots were healthy and ready to root ex vitro without affecting number of shoots (Fig. 1d). It is known that the ratio of ammonium and nitrate ions influence the quality of shoots (Ivanova and Staden 2009). Also, sugar/nitrogen ratio and their interaction with PGRs play an important role in morphogenesis, growth and development of plants in vitro. Different nitrate supply in the medium containing the same level of sucrose had significant effect on the growth of some plants (Nicotiana, Cymbidium, Syringa) propagated in vitro (Caboche 1987; Ogura-Tsujita and Okubo 2006; Gabryszewska 2011). There are many reports of using reduced nitrate concentration for improving shoot quality (Shimasaki and Uemoto 1990; Shekhawat et al. 1998; Rathore et al. 2004). Addition of (NH4)2SO4 improves the shoot vigour and overcomes the problem of tip burning and defoliation (Husain and Anis 2009). The cultures were maintained for 2 years by subculturing without any loss of growth and vigour. Subculturing period is critical for the survival and multiplication of the culture. When the cultures were kept on medium for more than 30 days, they showed decline in growth. In vitro flowering in cultures was obtained once in a year on the same medium (Fig. 1e).
Rooting
The in vitro produced shoots rooted in vitro as well as ex vitro. For in vitro rooting, one-fourth strength MS medium with 0.25 mg l−1 each of IBA and NOA and 100 mg l−1 of activated charcoal was found best with maximum number (5.3 ± 0.48) of roots with 4.72 ± 0.04 cm length (Fig. 1f; Table 4). On lower concentrations of IBA, roots were lesser in number and took more time in emerging. NOA alone was found less effective than combination of NOA and IBA with respect to root quality and number of roots. In comparison to one-fourth strength MS medium (80 %), percent rooting in regenerated shoots was significantly low on full (13.33 %) or half strength (16.37 %) of MS medium.
In comparison to in vitro, higher percent of regenerated shoots were rooted under ex vitro condition. About 90 % in vitro raised shoots rooted ex vitro within 7 days when treated with IBA 200 mg l−1 for 3.0 min, transferred to soilrite and grown under the greenhouse conditions (Fig. 1g; Table 5). Treatment with NOA showed delayed and poor response as compared to IBA. Similar to our observations the effect of IBA was found significant in inducing rooting as compared to NOA in Ceropegia bulbosa (Phulwaria et al. 2013). Plants by ex vitro approach are easy to harden and require less cost, time and labour. These have more vigour to tolerate stresses experienced during hardening (Benmahioul et al. 2012).
Hardening and acclimatization
Ex vitro rooting proved to be more beneficial than in vitro rooting in terms of percent survival of plants. The survival rate was significantly higher (90 %) in ex vitro rooted plantlets than those rooted in vitro (80 %). Usually ex vitro rooted plantlets have higher tolerance to stress imposed during the process of hardening because ex vitro rooted plantlets have similar roots as found in the natural root system which increases the transplantation efficiency as compared to in vitro rooted plantlets (Yan et al. 2010; Phulwaria et al. 2012). Overall 85 % of the micropropagated plants were hardened productively after a period of 45–50 days (Fig. 1h). The hardened and acclimatized plantlets were then successfully transferred to black polybags.
Micromorphological studies of micropropagated plants
Plant produced in culture bears normal leaves with prominent thick midrib and lateral veins. However, the leaves of C. gynandra under in vitro condition possessed fewer veins than the hardened plantlets in green house, and so the leaves of plant under in vitro condition have larger vein islet than the later stage (Fig. 2a, b). Earlier, several workers also reported the venation patterns and compared C4 and C3 species of genus Cleome, C4 plants have dense veins as compared to C3 plants (Brown et al. 2005; Marshall et al. 2007). We found that there was prominent change in structure and density of glandular trichomes during hardening. Under in vitro conditions, the trichomes were less frequent and underdeveloped which became fully developed in hardened plantlets (Fig. 2c, d). Stomata of leaves under in vitro condition were non-functional as these were always open and comparatively more frequent as compared to hardened plantlets where stomata were functional (Fig. 2e, f). Our study supports the fact that under in vitro condition plants shows altered morphology, physiology and anatomy (Chandra et al. 2010).
The work shows an efficient protocol for clonal propagation of C. gynandra. The plant is not available throughout the year in nature and this protocol enables us to produce desired genotype throughout the year. The vein clearing experiment shows how the vein density increases during the hardening period. Understanding the biology of hardening is useful for improving the cloning protocol and for large scale plant production. Plants under in vitro conditions resulted in altered morphology, anatomy and physiology. It is suggested that during hardening the plantlets show gradual transition of a C3 character to a C4 character, as it is previously mentioned C4 plants have denser veins than C3 plants, but evidences are still needed to prove that.
Author contribution
N. S. Rathore designed and performed the experiments and wrote the first draft of the manuscript. N. Rathore helped in data analysis. N. S. Shekhawat guided the research and edited the final version of the manuscript.
Abbreviations
- BA:
-
6-Benzyladenine
- IAA:
-
Indole-3-acetic acid
- IBA:
-
Indole-3-butyric acid
- Kin:
-
Kinetin
- MMS:
-
Modified Murashige and Skoog medium
- MS:
-
Murashige and Skoog medium
- NOA-2:
-
Naphthoxyacetic acid
- PFD:
-
Photon flux density
- PGRs:
-
Plant growth regulators
- RH:
-
Relative humidity
References
Albarello N, Simoes C, Rosas PFG, de Castro TC, Aldoni MGG, Callado CH, Mansur E (2006) In vitro propagation of Cleome spinosa (Capparaceae) using explants from nursery-grown seedlings and axenic plants. In Vitro Cell Dev Biol Plant 42:601–606
Anbazhagi T, Kadavul K, Suguna G, Petrus AJA (2009) Studies on pharmacognostical and in vitro antioxidant potential of Cleome gynandra Linn. leaves. Nat Prod Radian 8(2):151–157
Aparadh VT, Mahamuni RJ, Karadge BA (2012) Taxonomy and physiological studies in spider flower (Cleome species): a critical review. Plant Sci Feed 2(3):25–46
Benmahioul B, Dorion N, Kaid-Harche M, Daguin F (2012) Micropropagation and ex vitro rooting of pistachio (Pistacia vera L.). Plant Cell Tissue Organ Cult 108:353–358
Brown NJ, Parsley K, Hibberd JM (2005) The future of C4 research—maize, Flaveria or Cleome? Trends Plant Sci 10(5):215–221
Caboche M (1987) Nitrogen, carbohydrate and zinc requirements for the efficient induction of shoot morphogenesis from protoplast-derived colonies of Nicotiana plumbaginifolia. Plant Cell Tissue Organ Cult 8(3):197–206
Chandra S, Bandopadhyay R, Kumar V, Chandra R (2010) Acclimatization of tissue cultured plantlets: from laboratory to land. Biotechnol Lett 32:1199–1205
Chweya JA, Mnzava NA (1997) Promoting the conservation and use of underutilized and neglected crops 11. Cat’s Whiskers Cleome gynandra L., Institute of Plant Genetics and Crop Plant Research, Gatersleben, IPK Rome
Gabryszewska E (2011) Effect of various levels of sucrose, nitrogen salts and temperature on the growth and development of Syringa vulgaris L. shoots in vitro. J Fruit Ornament Plant Res 19(2):133–148
Hibberd JM, Sheehy JE, Langdale JA (2008) Using C4 photosynthesis to increase the yield of rice-rationale and feasibility. Curr Opin Plant Biol 11:228–231
Husain MK, Anis M (2009) Rapid in vitro multiplication of Melia azedarach L. (a multipurpose woody tree). Acta Physiol Plant 31:765–772
Ivanova M, Staden JV (2009) Nitrogen source, concentration, and NH4 +: NO3 − ratio influence shoot regeneration and hyperhydricity in tissue cultured Aloe polyphylla. Plant Cell Tissue Organ Cult 99:167–174
Koteyeva NK, Voznesenskaya EV, Roalson EH, Edwards GE (2011) Diversity in forms of C4 in the genus Cleome (Cleomaceae). Ann Bot 107(2):269–283
Marshall DM, Muhaidat R, Brown NJ, Liu Z, Stanley S, Griffiths H, Sage RF, Hibberd JM (2007) Cleome, a genus closely related to Arabidopsis, contains species spanning a developmental progression from C3 to C4 photosynthesis. Plant J 51:886–896
Mishra SS, Moharana SK, Dash MR (2011) Review on Cleome gynandra. Int J Res Pharm Chem 1(3):681–689
Muhaidat R, Sage RF, Dengler NG (2007) Diversity of kranz anatomy and biochemistry in C4 eudicots. Am J Bot 94(3):362–381
Murashige T, Skoog F (1962) A revised medium for rapid growth and bio-assays with tobacco tissue cultures. Physiol Plant 15:473–497
Naseem M, Jha KK (1997) Rapid clonal multiplication of Cleome gynandra DC. through tissue culture. Phytomorphology 47:405–411
Newell CA, Brown NJ, Liu Z, Pflug A, Gowik U, Westhoff P, Hibberd JM (2010) Agrobacterium tumefaciens-mediated transformation of Cleome gynandra L., a C4 dicotyledon that is closely related to Arabidopsis thaliana. J Exp Bot 61(5):1311–1319
Ochuodho JO, Modi AT (2007) Light-induced transient dormancy in Cleome gynandra L. seeds. Afr J Agric Res 2(11):587–591
Ogura-Tsujita Y, Okubo H (2006) Effects of low nitrogen medium on endogenous changes in ethylene, auxins and cytokinins in in vitro shoot formation from rhizomes of Cymbidium kanran. In Vitro Cell Dev Biol Plant 42:614–616
Phulwaria M, Rai MK, Harish, Gupta AK, Ram K, Shekhawat NS (2012) An improved micropropagation of Terminalia bellirica from nodal explant of mature tree. Acta Physiol Plant 34:299–305
Phulwaria M, Shekhawat NS, Rathore JS, Singh RP (2013) An efficient in vitro regeneration and ex vitro rooting of Ceropegia bulbosa Roxb.—a threatened and pharmaceutical important plant of Indian Thar Desert. Ind Crop Prod 42:25–29
Rathore V, Shekhawat NS, Singh RP, Rathore JS, Dagla HR (2004) Cloning of adult trees of jamun (Syzygium cuminii). Ind J Biotechnol 3:241–245
Sage RF (2003) The evolution of C4 photosynthesis. New Phyt 161:341–370
Sage RF, Kubien D (2007) The temperature response of C3 and C4 photosynthesis. Plant Cell Environ 30:1086–1106
Sankhla N, Ziegler H, Vyas OP, Stichler W, Trimborn P (1975) Eco-physiological studies on Indian arid zone plants. Oecologia 21:123–129
Shekhawat NS, Singh RP, Deora NS, Kaur G, Kotwal RC, Choudhary N (1998) Micropropagation of plants of stressed ecosystems. In: Srivastava PS (ed) Plant tissue culture and molecular biology: application and prospects. Narosa, New Delhi, pp 579–586
Shekhawat NS, Phulwaria M, Harish, Rai MK, Kataria V, Shekhawat S, Gupta AK, Rathore NS, Vyas M, Rathore N, Vibha JB, Choudhary SK, Patel AK, Lodha D, Modi R (2012) Bioresearches of fragile ecosystem/desert. Proc Natl Acad Sci India 82:319–334
Shimasaki K, Uemoto S (1990) Micropropagation of a terrestrial Cymbidium species using rhizomes developed from seeds and pseudobulbs. Plant Cell Tissue Organ Cult 22:237–244
Simoes C, Albarello N, Callado CH, de Castro TC, Mansur E (2009) New approaches for shoot production and establishment of in vitro root cultures of Cleome rosea Vahl. Plant Cell Tissue Organ Cult 98:79–86
Walther GR, Post E, Convey P, Menzel A, Parmesank C, Beebee TJC, Fromentin JM, Guldberg OH, Bairlein F (2002) Ecological responses to recent climate change. Nature 416:389–395
Yan H, Liang C, Yang L, Li Y (2010) In vitro and ex vitro rooting of Siratia grosvenorii, a traditional medicinal plant. Acta Physiol Plant 32:115–120
Acknowledgments
We are thankful to Council of industrial and scientific research (CSIR), New Delhi and University grant commission (UGC), New Delhi for financial assistance in the form of Senior Research Fellowship (SRF) to authors Nitika Singh Rathore and Nisha Rathore, respectively. We also thank to Dr. Manoj Kumar Rai, Biotechnology Centre, Department of Botany, JNV University, Jodhpur, Rajasthan for their valuable suggestions and improvement of the manuscript.
Author information
Authors and Affiliations
Corresponding author
Additional information
Communicated by E. Lojkowska.
Rights and permissions
About this article
Cite this article
Rathore, N.S., Rathore, N. & Shekhawat, N.S. In vitro propagation and micromorphological studies of Cleome gynandra: a C4 model plant closely related to Arabidopsis thaliana . Acta Physiol Plant 35, 2691–2698 (2013). https://doi.org/10.1007/s11738-013-1301-2
Received:
Revised:
Accepted:
Published:
Issue Date:
DOI: https://doi.org/10.1007/s11738-013-1301-2