Abstract
To analyse nodular expression of antioxidant enzymes depending on plant genotype and salinity, two Phaseolus vulgaris genotypes, tolerant BAT477 and sensitive COCOT, were inoculated with the reference strain Rhizobium tropici CIAT899 and grown under 25 and 50 mM NaCl. Plant growth, nodulation and nitrogen fixing activity measured by the acetylene reducing activity (ARA) as an indicator of nitrogenase (E.C. 1.7.9.92) activity were more affected by salt concentrations in COCOT than in BAT477, particularly with 50 mM NaCl. Electrophoresis analysis of antioxidant enzymes in nodules, roots and free-living rhizobia showed that only catalase (CAT E.C. 1.11.1.6) isoenzymes varied with genotype. The sensitive genotype showed lower antioxidant enzyme activities than tolerant genotype and it was more affected by salinity. In the tolerant genotype catalase and ascorbate peroxidase (APX, E.C. 1.11.1.11) were inhibited by salt stress, whereas superoxide dismutase (SOD, E.C. 1.15.1.1) and peroxidase (POX, E.C. 1.11.1.7) were activated by salinity. Statistical analysis allowed suggesting that tolerance to salinity is associated with a differential regulation of distinct superoxide dismutase and peroxidase activities.
Similar content being viewed by others
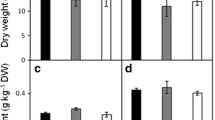
Explore related subjects
Discover the latest articles, news and stories from top researchers in related subjects.Avoid common mistakes on your manuscript.
Introduction
Salinity is one of the major abiotic stresses affecting legume production in arid and semi arid regions, particularly when these plants depend on symbiotic N2 fixation for their nitrogen requirements (Drevon et al. 2001). Soil salinity can limit productivity by adversely affecting the growth of host plant, symbiotic development of root-nodule, bacteria and nitrogen fixation capacity (Delgado et al. 1993). Nitrogenase, the key enzyme of nitrogen fixation, was subjected to a fragile equilibrium. Nevertheless, a high respiration rate is required to support the nitrogen fixation process (Jebara and Drevon 2001), and this leads to the generation of large amounts of reactive oxygen species (ROS; Blokhina et al. 2003). Moreover, during the earlier stages of plant–rhizobia interaction, the host plant react to the invasion of bacteria by the over production of ROS to initiate the hypersensitive reaction (Santos et al. 2000) which are responsible for most of the oxidative damage in biological systems (Reddy et al. 2004) including DNA nicking, amino acid and protein oxidation and lipid peroxidation (Johnson et al. 2003). Salinity induces the production of stress proteins or antioxidant enzymes to minimise damages caused by ROS (Matamoros et al. 2003). Mechanisms of ROS detoxification exist in all plants and can be categorized as non enzymatic-(flavanones, carotenoids and ascorbic acid) and enzymatic antioxidants such as superoxide dismutases (SOD, E.C. 1.15.1.1), a metalloenzyme playing a key role in protection against oxidative stress (Moran et al. 2003), peroxidases (POX, E.C. 1.11.1.7), ascorbate peroxidases (APX, E.C. 1.11.1.11) belongs to ASC–GSH cycle and collectively functions as a peroxide-scavenging mechanism (Comba et al. 1998). The catalases (CAT, E.C. 1.11.1.6) are located mostly in peroxisomes and glyoxisomes (Scandalios et al. 1997); bacteroids also contain high levels of catalase, but no peroxidases (Becana et al. 2000).
The objective of this work was to investigate the effect of salt stress on two genotypes of Phaseolus vulgaris BAT477 and COCOT inoculated by the reference strain CIAT899. The correlation between nodular antioxidant enzyme activities and symbiotic performance was also discussed in order to find out likely mechanisms that might help to cope with salinity.
Materials and methods
Biological materials
The bean seeds of BAT477 (supplied by S. Beebe, CIAT, Colombia) or COCOT (provided by M Trabelsi, ESA Mateur, Tunisia) were surface sterilized, inoculated with the reference strain Rhizobium tropici CIAT899 and germinated on perlite.
Trials were performed in a temperature-controlled glasshouse with night/day temperature of 25°/35°C and 16 h photoperiod; the irradiance was supplied by mercury vapor lamps (OSRAM HQI-T400W/DH). Plants were grown in 1-L glass bottles, wrapped with aluminium foil to maintain darkness in the rooting environment. Plants received the nutrient solution of Vadez et al. (1996) added with 1 g L−1 CaCO3 to maintain pH near 7, and renewed every 2 weeks.
Seven days after sowing (DAS), the plant seedlings were transferred to bottles and reinoculated by the supply of 1 mL of the aforementioned inoculant directly into the nutrient solution aerated with air flow of 400 mL min−1 (Jebara et al. 2001). At 20 DAS, the solution was renewed, and the plants were exposed to salinity by adding NaCl (final concentration 25 or 50 mM) to the growth medium. Plants were harvested at 42 DAS corresponding to flowering stage.
Nitrogen-fixing assay: nitrogenase activity
Nitrogenase (E.C. 1.7.9.92) activity was assayed by acetylene reduction activity, ARA, (Hardy et al. 1968) using gas chromatography with Porapak-T column. All gas measurements were performed with five replicates per treatment within 35–42 DAS, corresponding to late vegetative or early flowering stages. Nodule-bearing roots were incubated in 10% C2H2 atmosphere. After 60 min of incubation, three replicates of 0.5 mL samples were withdrawn from the root atmosphere of each plant (three plant/association), and ethylene production was measured by gas chromatography. Pure acetylene and ethylene were used as internal standards; the use of such a closed system for measuring acetylene reduction, was only for comparative purposes (Vadez et al. 1996).
Preparation of enzyme extracts
The extraction of total proteins and enzyme activities was made at 4°C with 3 g fresh nodules or roots which were harvested directly into liquid nitrogen in a mortar, with 50 mg PVP, 1 mM PMSF, 10 mM DTT and 0.1 mM EDTA, in 50 mM potassium phosphate buffer pH 7.8. For analysis of APX, the extraction buffer also contained 5 mM ascorbate (Gogorcena et al. 1997). Protein concentration was estimated according to Bradford (1976) using bovine serum albumin as standard.
Enzyme assays
Spectrophotometric determination of SOD (E.C. 1.15.1.1) activity was assayed by monitoring the inhibition of photochemical reduction of nitro blue tetrazolium (NBT) according to the protocol of Yu and Rengel (1999). One unit of SOD activity was defined as the amount of enzyme required to cause 50% inhibition of reduction of NBT as monitored at 560 nm. SOD was revealed by NBT using the procedures of Beauchamp and Fridovich (1971) on 10% acrylamide native gel (Laemmli 1970).
The peroxidase (POX, E.C. 1.11.1.7) activity was measured with a reaction medium containing 50 mM phosphate buffer pH 7, 9 mM guaiacol and 19 mM H2O2 according to Anderson et al. (1995). The kinetic evolution of tetraguaiacol formation from guaiacol at 470 nm was measured for 1 m. Peroxidase activity was calculated using the extinction coefficient (E = 26.6 mM−1 cm−1). One unit of peroxidase was defined as the amount of enzyme that caused the formation of 1 μM of tetraguaiacol/min. The electrophoretic separation of POX was performed on native 10% acrylamide gel pH 8.8. Then, the gel was incubated in acetate buffer pH 4 in the dark at 30°C for 30 min, and the revelation of acid peroxidase bands was obtained by using the peroxides in acetate buffer pH 5 (Vallejos 1983).
The ascorbate peroxidase (APX, E.C. 1.11.1.11) activity was measured by monitoring the decline at 290 nm as ascorbate (E = 2.8 mM−1 cm−1) was oxidized for 3 min (40 s lag period) according to Amako et al. (1994). In 1 mL reaction volume containing 50 mM potassium phosphate buffer pH 7; 10 μL enzyme extracts 0.1 mM hydrogen peroxide and 0.5 mM ascorbate. Adding the H2O2 started the reaction. APX isozymes were separated on nondenaturing acrylamide gels 10% supported by 10% glycerol and stained according to Mittler and Zilinskas (1993).
The catalase (CAT, E.C. 1.11.1.6) activity was measured according to the method of Aebi (1984) by following the decline at 240 nm as H2O2 (E = 36 M−1 cm−1) was catabolized, in reaction mixture containing 20 μL enzyme extract in 50 mM potassium phosphate buffer pH 7, the reaction was started by adding H2O2 at final concentration 15 mM, and its consumption was measured for about 30 s at 240 nm. CAT isoenzymes were separated on nondenaturating acrylamide gels 7% at 100 V for 2 h at 4°C. Gels were then soaked in 3.27 mM H2O2 for 15 min, rinsed in water, and stained in a solution of 2% potassium ferricyanide and 2% ferric chloride (Anderson et al. 1995).
Statistical analysis
The growth values and parameters related to nitrogen fixation were means of nine replicates per treatment. Six replicates were performed for the enzyme activity assays and protein content. All results were subjected to a two-away analysis of variance with least significant difference (LSD) test between means (P < 0.05) and Duncan’s multiple range test. Statistics regressions were computed with Statistica software.
Results
Effect of salt stress on plant growth and nodulation
Analysis of growth parameters in control condition showed that BAT477 had a superior vegetative growth and nodulation than COCOT (Table 1). Both genotypes showed a significant decrease in their biomass under 25 mM NaCl, and a higher decrease was observed under 50 mM NaCl. In BAT477, shoot dry weight (SDW) was 27 and 39% inhibited by 25 and 50 mM NaCl, respectively. The root dry weight (RDW) was less inhibited than the SDW, but only the application of 50 mM NaCl inhibited RDW up to 31%; the nodule dry weight (NDW) was the most inhibited by both salt concentrations (Table 1). The COCOT SDW inhibition was 37% under 25 mM NaCl and reached 54% under 50 mM NaCl; the RDW was significantly inhibited by salt treatments, while the most affected parameter was NDW which was inhibited about 62% by 50 mM NaCl (Table 1).
The ARA was significantly higher in BAT477 than in COCOT (Table 1) and reached a maximal value at the harvest (42 DAS). Application of 25 mM NaCl induced a 53% decrease in ARA for BAT477, whereas 50 mM NaCl induced a strong inhibition that nodules preserved only 10% residual activity. The ARA inhibition in COCOT was 66% by 25 mM NaCl, and 50 mM NaCl caused a total inhibition of the ARA.
The specific nitrogen fixation activity assessed by the ratio ARA NDW−1 decreases more accurately in COCOT than in BAT477, which confirms and differentiates tolerant from sensitive genotypes.
Salt stress effect on protein content
The total protein content in nodules and roots was higher in BAT477 than in COCOT (Table 1). Overall nodules were richer in protein content than in roots whenever the genotype and nodules were more affected by salinity than roots for both symbioses. The decrease in nodule protein in BAT477 did not exceed 10% under 25 mM NaCl and was about 23% under 50 mM NaCl, whereas in COCOT this decrease was, respectively, 16 and 44%. The proteins content in root was only affected by the highest salt treatments in BAT477, whereas the decrease reached 23% in COCOT with tested treatment.
The analysis of a total variance indicated that genotype effect was involved essentially in SDW and RDW, whereas treatment effect had a marked effect on nodulation parameters ARA and NDW. These effects increased significantly under salt stress, whereas we did not measure significant effect in genotype-treatment correlation (Table 1).
Modulation of enzymatic antioxidant activity under salinity
Superoxide dismutase
In the two studied genotypes, superoxide dismutase (SOD) showed higher activity in roots than in nodules, and this activity was significantly higher in COCOT than in BAT477, whereas SOD activity was equal in nodules for both symbioses (Table 2). BAT477 root SOD activity was significantly inhibited by both salt treatments, whereas in nodules we measured 26% activation under 25 mM NaCl and about 50% under 50 mM NaCl.
The COCOT root SOD activity was 31% inhibited under 25 mM but 25% activated under 50 mM NaCl, whereas in nodules we noted 42% decrease under 25 mM NaCl, but only 12% decrease under 50 mM NaCl. The two tested genotypes had a contrasting nodule SOD response; thus in sensitive genotype COCOT nodule SOD was more inhibited with 25 mM than 50 mM NaCl.
Peroxidase
The BAT477 genotype was richer in peroxidase (POX) activities than COCOT, and nodule POX content was higher than root one (Table 2).
The two tested salt stress concentrations induced proportional activation of BAT477 nodule and root POX activities.
The COCOT root POX activity was 71% increased with 25 mM, but 53% inhibited with 50 mM NaCl, whereas in nodules, salt treatment led to 22% activation with 25 mM NaCl while 50 mM NaCl application induced 46% inhibition (Table 2). Indeed, COCOT nodule and root POX activities were inhibited by severe salt treatment, confirming the sensibility of this symbiosis.
Ascorbate peroxidase APX
COCOT genotype was richer in nodule- and root APX than BAT477 genotype; in the latter, root APX variation was not significant under 25 mM NaCl and increased with 50 mM NaCl. The application of 25 mM NaCl showed a statically significant inhibition (16%) on BAT477 nodular APX and under 50 mM NaCl the inhibition reached 39% (Table 2). The COCOT APX root activity was strongly activated (142%) with 25 mM NaCl and this activation was two times higher (286%) with 50 mM NaCl.
The COCOT nodular APX inhibition was more important in the order of 86% in both 25 and 50 mM independently of salt concentration. Inversely to POX, APX nodular activity of COCOT genotype was more affected by salinity than BAT477.
Catalase CAT
The BAT477 nodules were richer in CAT activity than COCOT and no catalase activity was detected in roots. Application of 25 and 50 mM NaCl caused, respectively, 5 and 55% inhibition of BAT477 nodule activity (Table 2). Catalase activity was 41% inhibited with 25 mM at COCOT nodules and decreased about 57% with 50 mM NaCl which was comparable to the BAT477 response.
However, in rhizobial suspension, CAT activity was considerably low: about 0.12 ± 0.02 mM min−1 μg−1 protein. The differential inhibition of CAT was concomitant with differential responses of symbioses to salt treatment. Therefore, high CAT inhibition was registered with salt treatment either in BAT477 or in COCOT genotype.
Results of a total variance analysis showed significant genotype effect on POX and APX, whereas treatment had a significant effect on SOD and CAT. For all analysed parameters, the genotype-treatment interaction was lower; however it increased with salinity (Table 2).
The analysis of SOD, POX and APX on native polyacrylamide 10% gel did not show variability between two genotypes; the enzymes exist as well in COCOT genotype as in BAT477 genotype (Fig. 1). The effect of two used salt concentrations was only distinguished even though all samples contained the same protein quantity. However, no POX or APX activity was measured or revealed on the free CIAT899 strain. The free-living rhizobia had a low SOD activity in the order of 2.9 ± 0.4 × 10−3 U SOD which was relatively low compared with nodule or root SOD.
Detection on 10% native polyacrylamide gel of a, superoxide dismutase, b peroxidase and c, ascorbate peroxidase activities. Effect of NaCl on BAT477 and COCOT roots (1 control) and nodules (2 control; 3 25 mM NaCl treated; 4 50 mM NaCl treated). No detected APX or POX rhizobial activity. All samples contained 30 μg of protein
The CAT activity analysis on native polyacrylamide gel (7%) revealed only one isozyme in free rhizobial suspension; therefore, BAT477 nodule had two isozymes, CAT1 and CAT3 (Fig. 2). The COCOT nodule revealed CAT1 and CAT2 isozymes. The CAT1 isozyme had strain origin because it was common in two symbioses while the isozyme CAT2 was specific to COCOT genotype and the CAT3 isozyme was specific to BAT477 and no catalase activity was detected in roots. Then, each genotype had a specific nodular CAT isoenzyme, so this polymorphism detected may be related to the difference observed in response to stress. Subsequently, it was shown that host genotype can influence the behaviour of symbioses salt-treated, and these isoenzymes were interesting for further characterisation.
Discussion
Our results showed that all studied parameters were modulated dependently according to applied salt concentration which like all other abiotic constraints slowed down the plant growth and this slowing was a way to a mechanism of stress adaptation (Zhu 2001), particularly at some legumes such as P. vulgaris (Jebara et al. 2001; Tejera et al. 2004). The effect of salt was more expressed on NDW which was the most inhibited parameters as previously described (Vadez and Drevon 2001; Jebara et al. 2005). Reduced nodule formation of the common bean at low levels of salinity could be due to adverse effects on the process of nodule initiation; this process had been reported to be very sensitive to osmotic stress (Velagaleti et al. 1990). However, other reports suggested that nodule growth reduction was linked to the inhibition of enzymes associated with sucrose degradation (Gonzalez et al. 2001).
Statistical analysis of correlation between all studied parameters showed that for both genotypes, NDW was highly correlated to RDW, SDW and ARA, which allowed the suggestion that the genotype performance was closely related to nodule response to stress. It is already demonstrated that the relative genotype tolerance seems to be due to its ability to higher leaf potential, adequate leaf area and abundant and efficient nodular system, which in turn determines an important rate of SNF (Sassi Aydi et al. 2008).
The salt treatment significantly inhibited ARA which was significantly related (r = 0.95) to high NDW inhibition, and these high inhibitions concord with works that observed with the chickpea nodules an important decrease in ARA with NaCl treatment (Sheokand et al. 1995; Mhadhbi et al. 2004).
According to our results, salinity adjusted the activity of the main enzyme that protects nodular tissues against oxidative damage in relation to genotype ability. The two tested genotype had a contrasting nodule SOD response, so BAT477 response concord with result previously described by Tejera et al. (2004), whereas COCOT nodule SOD was more inhibited with 25 than 50 mM NaCl which showed a relationship with their intrinsic performance as a response to the drastic nodule-structure destruction caused by ROS and proteases generated under salinity. So, the SOD stability, mainly root ones, under moderated oxidative stress may be explained by the reduction of oxygen supply, as a consequence of the nodular cortex permeability decrease by salinity that limits the main source of ROS production (Rubio et al. 2002).
The nodular POX activation was more significant in the BAT477 and showed high correlation (r = 0.98) between SOD and POX activities which suggests these antioxidants play a crucial role in the tolerance mechanism of common bean nodules to stress. Thus, the sensibility of COCOT genotype may be due partially to the absence of this correlation between SOD and POX activities. In fact, 50 mM NaCl induced a severe osmotic pressure which caused a decrease of protein expression provoked by higher reticence of the gene expression in sensible genotype than in tolerant one (Cruz de Carvalho et al. 2001). Indeed, COCOT nodule POX root and nodule activities were inhibited by severe salt treatment, confirming the sensibility of this symbiosis and permit to deduce that nodular POX activation by salinity contributes to the symbiosis protection which confirms the observations of Sheokand et al. (1995), and it was suggested to play an important role in plant salt tolerance (Matamoros et al. 2003; Mhadhbi et al. 2004).
The APX nodular activity of COCOT genotype was more affected by salinity than BAT477 one and this inhibition was more pronounced and independent of the salt concentration. These results suggest the secondary role of this activity to protect nodular tissues. In fact, APX has been shown to enhance N2 fixation up to 4.5-fold in in vitro model system consisting of bacteria (Azorhizobium) or soybean nodules bacteroids (Ross et al. 1999). The concomitant decline in the enzyme activities of the ASC–GSH pathway suggest a key link between the process of N2 fixation and the mechanism for protection against ROS (Swaraj et al. 1993). These results suggest the secondary role of APX activity to protect nodular tissues which was strongly supported by the close correlation between ARA and APX activity (r = 1) in COCOT nodules.
The differential inhibition of CAT was concomitant with differential responses of symbioses to salt treatment. Therefore, high CAT inhibition was registered with salt treatment either in BAT477 or in COCOT genotype remembers a considerable decline in chickpea catalase nodule activity with 50 and 100 mM NaCl, up to 44 and 47% respectively (Sheokand et al. 1995) and in common bean (Tejera et al. 2004).
On the other hand, the high negative correlation between SOD and CAT activities (r = −0.90) observed essentially in BAT477 genotype suggests that SOD activity could be a protective enzyme that blocks the inhibition of nitrogenase activity by oxygen radicals or by superoxide anions. The decrease in catalase would lead to increase the hydrogen peroxide levels, although this accumulation in tissues could also be due to the action of SOD. According to COCOT genotypes we notice high correlation (r = 0.96) between APX and CAT activities, and both activities were correlated with growth and nodulation; this suggests that CAT and APX enzymes act as key detoxifying enzymes under stress conditions in sensitive symbiosis, confirming the role of antioxidant defence in P. vulgaris plant facing osmotic stress (Sassi et al. 2008).
Moreover, an interesting result that each genotype had a specific nodular CAT isoenzyme, so this only detected polymorphism in tested activities may be related to difference observed response to stress. Subsequently, it was shown that host genotype can influence the behaviour of symbioses salt treated and these isoenzymes were interesting for further characterisation. In fact, many catalase activities play an important role for maintaining nodule function (Jamet et al. 2003).
In conclusion, our results indicate that symbiotic efficiency under salinity might be related to the antioxidant enzyme activity capacities and with interaction between rhizobia and plant host. Furthermore, the high correlation coefficient of SOD and POX with genotype efficiency under salinity suggests that tolerance to salinity is associated with a differential regulation suggesting that these two antioxidant enzyme activities are molecular markers for salt tolerance.
Abbreviations
- APX:
-
Ascorbate peroxidase
- ARA:
-
Acetylene reducing activity
- ASC-GSH cycle:
-
Ascorbate–glutathione cycle
- CAT:
-
Catalase
- EDTA:
-
Ethylenediaminetetraacetic acid
- MTT:
-
3-(4,5-Dimethlthiazol-2-4)-5-5diphenyl tetrazolium bromide
- NBT:
-
Nitroblue tetrazolium
- NDW:
-
Nodule dry weight
- PMSF:
-
Phenylmethylsulfonyl fluoride
- POX:
-
Guaiacol peroxidase
- PVP:
-
Polyvinyl-pyrrolidone
- ROS:
-
Reactive oxygen species
- RDW:
-
Root dry weight
- SDW:
-
Shoot dry weight
- SOD:
-
Superoxide dismutase
- TEMED:
-
N,N,N′,N′-tetramethyl ethylene diamine
- Tris:
-
Tris (hydroxymethyl) aminomethane
References
Aebi H (1984) Catalase in vitro. Methods Enzymol 105:121–126
Amako K, Chen GX, Asada K (1994) Separate assays specific for ascorbate peroxidase and guaiacol peroxidase and for the chloroplastic and cytosolic isozymes of ascorbate peroxidase in plants. Plant Cell Physiol 35:497–504
Anderson MD, Prasad TK, Stewart CR (1995) Changes in isozymes profiles of catalase, peroxidase, and gluthatione reductase during acclimation to chilling in mesocotyls of maize seedling. Plant Physiol 109:1247–1257
Beauchamp C, Fridovich I (1971) Superoxide dismutase: improved assays and an assay applicable to acrylamide gels. Anal Biochem 44:276–287
Becana M, Dalton DA, Moran JF, Iturbe-Ormaetxe I, Matamoros MA, Rubio MC (2000) Reactive oxygen species and antioxidants in legume nodules. Physiol Plant 109:372–381
Blokhina O, Virolaonen E, Fagerstedt KV (2003) Antioxidants, oxidative damage and oxygen deprivation stress. Anal Bot 91:179–194
Bradford M (1976) A rapid and sensitive method for the quantification of microgram quantities of proteins utilising the principal of protein-dye binding. Anal Biochem 72:248–254
Comba ME, Benavides MP, Tomaro ML (1998) Effect of salinity on antioxidant defence system in soybean root nodules. Aust J Plant Physiol 25:665–671
Cruz de Carvalho MH, Arcy-Lameta A, Roy-Macauley H, Gareil M, El Maarouf H, Pham-Thi, Zuily-Fodil Y (2001) Aspartic protease in leaves of common bean (Phaseolus vulgaris L.) and cowpea (Vigna unguiculata L. Walp): enzymatic activity, gene expression and relation to drought susceptibility. FEBS Lett 492:242–246
Delgado MJ, Garrido JM, Ligero F, Lluch C (1993) Nitrogen fixation and carbon metabolism by nodules and bacteroide of pea plants under sodium chloride stress. Physiol Plant 89:824–829
Drevon JJ, Abdelly C, Amarger N, Aouani ME, Aurag J, Gherbi H, Jebara M et al (2001) An interdisciplinary research strategy to improve symbiotic nitrogen fixation and yield of common bean (Phaseolus vulgaris) in salinised areas of the Mediterranean basin. J Biotechnol 91:257–268
Gogorcena Y, Gordon AJ, Escuredo PR, Minchin FR, Witty JF, Moran JF, Becana M (1997) N2 fixation, carbon metabolism, and oxidative damage in nodules of dark-stressed common bean plants. Plant Physiol 113:1193–1201
Gonzalez EM, Galvez L, Royuria M, Aparicio-Tejo PM, Arresse-igor C (2001) Insights into regulation of nitrogen fixation in pea nodules: lessons from drought, abscisic acid and increased photoassimilate availability. Agronomy 21:607–613
Hardy RWF, Holston RD, Jakson EK, Burns RC (1968) The acetylene-ethylene assay for nitrogen fixation: laboratory and field evaluation. Plant Physiol 43:1185–1208
Jamet A, Sigaud S, Van de Sype G, Puppo A, Hérouart D (2003) Expression of the bacterial catalase genes during Sinorhizobium meliloti- Medicago sativa symbiosis and their crucial role during the infection process. Mol Plant Microbe Int 16:217–225
Jebara M, Drevon JJ (2001) Genotypic variation in nodule conductance to the oxygen diffusion in common bean (Phaseolus vulgaris L.). Agronomy 21:667–674
Jebara M, Drevon JJ, Aouani ME (2001) Effects of hydroponic culture system and NaCl on interactions between common bean lines and native rhizobia from Tunisian soils. Agronomy 21:601–605
Jebara S, Jebara M, Limam F, Aouani ME (2005) Changes of ascorbate peroxidase, catalase, guaiacol peroxidase and superoxide dismutase activities in common bean (Phaseolus vulgaris) nodules under salinity. J Plant Physiol 162:929–936
Johnson SM, Doherty SJ, Croy RRD (2003) Biphasic superoxide generation in potato tubers. A self amplifying response to stress. Plant Physiol 13:1440–1449
Laemmli UK (1970) Cleavage of structural proteins during the assembly of the head of bacteriophage T4. Nature 277:680–685
Matamoros MA, Dalton DA, Ramos J, Clemente MR, Rubio MC, Becana M (2003) Biochemistry and molecular biology of antioxidants in the rhizobia–legume symbiosis. Plant Physiol 133:499–509
Mhadhbi H, Jebara M, Limam F, Aouani ME (2004) Rhizobial strain involvement in plant growth, nodule protein composition and antioxidants enzymes expression of chickpea–rhizobia symbioses: modulation by salinity. Plant Physiol Biochem 42:717–722
Mittler R, Zilinskas BA (1993) Detection of ascorbate peroxidase activity in native gels by inhibition of ascorbate-dependent reduction of nitroblue tetrazolium. Anal Biochem 212:540–546
Moran JF, James EK, Rubio MC, Sarath G, Klucas RV, Becana M (2003) Functional characterization and expression of a cytosolic iron-superoxide dismutase from Cowpea root nodules. Plant Physiol 133:773–782
Reddy RA, Chaitanya VK, Vivekanandan M (2004) Drought-induced responses of photosynthesis and antioxidant metabolism in higher plants. J Plant Physiol 161:1189–1202
Ross EJH, Kramer SB, Dalton DA (1999) Effectiveness of ascorbate and ascorbate peroxidase in promoting nitrogen fixation in model systems. Phytochem 52:1203–1210
Rubio MC, Gonzalez EM, Minchin FR, Webb KJ, Arrese-Igor C, Ramos J, Becana M (2002) Effects of water stress on antioxidant enzymes of leaves and nodules of transgenic alfalfa overexpressing superoxide dismutases. Physiol Plant 115:531–540
Santos R, Hérouart D, Puppo A, Touati D (2000) Critical protective role of bacterial superoxide dismutase in Rhizobium–legume symbiosis. Mol Microbiol 38:750–759
Sassi Aydi S, Aydi S, Gonzalez E, Abdelly C (2008) Osmotic stress affects water relations, growth, and nitrogen fixation in Phaseolus vulgaris plants. Acta Physiol Plant 30:441–449
Sassi S, Gonzalez EM, Aydi S, Arrese-Igor C, Abdelly C (2008) Tolerance of common bean to long-term osmotic stress is related to nodule carbon flux and antioxidant defences: evidence from two cultivars with contrasting tolerance. Plant Soil 312:39–48
Scandalios JG, Guan L, Polidords AN (1997) Catalases in plants: gene structure, proprieties, and expression. In: Scandalios JG (ed) Oxidative stress and the molecular biology of antioxidant defences. Cold Spring Harbor Laboratory Press, Cold Spring Harbor, NY, pp 343–406
Sheokand S, Dhandi S, Swaraj K (1995) Studies on nodule functioning and hydrogen peroxide scavenging enzymes under salt stress in chickpea nodule. Plant Physiol Biochem 33:561–566
Swaraj K, Laura JS, Bishnoi NR (1993) Nitrate induced nodule senescence and changes in activities of enzymes scavenging H2O2 in clusterbean (Cyamopsis tetragonaloba Taub.). J Plant Physiol 41:202–205
Tejera NA, Campos R, Sanjuan J, Lluch C (2004) Nitrogenase and antioxidant enzyme activities in Phaseolus vulgaris nodules formed by Rhizobium tropici isogenic strains with varying tolerance to salt stress. J Plant Physiol 3:329–338
Vadez V, Drevon JJ (2001) Genotypic variability in phosphorus use efficiency for symbiotic N2 fixation in common bean (Phaseolus vulgaris). Agronomy 21:691–699
Vadez V, Rodier F, Payre H, Drevon JJ (1996) Nodule conductance to O2 and nitrogenase-linked respiration in bean genotypes varying in the tolerance of N2 fixation to P deficiency. Plant Physiol Biochem 34:871–878
Vallejos CE (1983) Enzyme activity staining. In: Tanksley SD, Orton TJ (eds) Isozymes in plant genetics and breeding, Part A. Elseviers, Amsterdam, p 469–516
Velagaleti RR, Marsh S, Kramer D (1990) Genotypic differences in growth and nitrogen fixation among soybean (Glycin Max (L.) Merr.) cultivars grown under salt stress. Trop Agric 67:169–177
Yu Q, Rengel Z (1999) Micronutrient deficiency influences plant growth and activities of superoxide dismutase in narrow-neafed lupins. Ann Bot 83:175–182
Zhu JK (2001) Plant salt tolerance. Plant Sci 6:66–71
Acknowledgments
This work was supported by grants from the Tunisian Ministry of Scientific Research, Technology and Competency Development. We are most grateful to Dr. Inoubli T for English revision. We thank Zitoun A, for excellent technical support.
Author information
Authors and Affiliations
Corresponding author
Additional information
Communicated by G. Klobus.
Rights and permissions
About this article
Cite this article
Jebara, S., Drevon, J.J. & Jebara, M. Modulation of symbiotic efficiency and nodular antioxidant enzyme activities in two Phaseolus vulgaris genotypes under salinity. Acta Physiol Plant 32, 925–932 (2010). https://doi.org/10.1007/s11738-010-0480-3
Received:
Revised:
Accepted:
Published:
Issue Date:
DOI: https://doi.org/10.1007/s11738-010-0480-3