Abstract
Although multiple sources link inflammation with attention difficulties, the only human study that evaluated the relationship between systemic inflammation and attention problems assessed attention at age 2 years. Parent and/or teacher completion of the Childhood Symptom Inventory-4 (CSI-4) provided information about characteristics that screen for attention deficit hyperactive disorder (ADHD) among 793 10-year-old children born before the 28th week of gestation who had an IQ ≥ 70. The concentrations of 27 proteins in blood spots obtained during the first postnatal month were measured. 151 children with ADHD behaviors were identified by parent report, while 128 children were identified by teacher report. Top-quartile concentrations of IL-6R, TNF-α, IL-8, VEGF, VEFG-R1, and VEGF-R2 on multiple days were associated with increased risk of ADHD symptoms as assessed by a teacher. Some of this increased risk was modulated by top-quartile concentrations of IL-6R, RANTES, EPO, NT-4, BDNF, bFGF, IGF-1, PIGF, Ang-1, and Ang-2. Systemic inflammation during the first postnatal month among children born extremely preterm appears to increase the risk of teacher-identified ADHD characteristics, and high concentrations of proteins with neurotrophic properties appear capable of modulating this increased risk.
Similar content being viewed by others
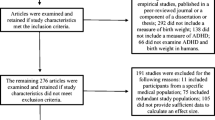
Avoid common mistakes on your manuscript.
Introduction
The lower the gestational age at birth, the higher the risk of an attention deficit hyperactivity disorder (ADHD) (Sucksdorff et al. 2015). The reasons for this heightened risk with greater immaturity remain obscure.
One hypothesis, invokes inflammation. Assisted (i.e., mechanical) ventilation and bacteremia are likely the two most common contributors to sustained or recurrent inflammation in the extremely preterm newborn (Dammann and Leviton 2014). The reasons extremely preterm newborns are at heightened risk for bacteremia are complex, but probably include exposure to the mother’s organisms, fragile skin, indwelling arterial and venous tubes, need for assisted ventilation, deficient expression of complement, as well as a deficiency of antimicrobial proteins and peptides (Dammann and Leviton 2014). Once the inflammation is initiated, it appears to be sustained (Dammann et al. 2016).
Support for an inflammation-ADHD relationship comes from human studies of early postnatal infection (Rand et al. 2016), early postnatal inflammation (O’Shea et al. 2014), and later postnatal inflammation (Ceylan et al. 2012; Shyu et al. 2012; Holtmann et al. 2013; Mitchell and Goldstein 2014), as well as genetic propensities to inflammation (Zayats et al. 2015).
Another hypothesis attributes some of this to a separation of the fetus from the source of growth factors provided by mother and/or placenta before the newborn can synthesize adequate amounts (Reuss et al. 1994; Dammann and Leviton 1999). Growth factors with neurotrophic and/or angiogenic properties have the potential to minimize the occurrence of brain damage (Larpthaveesarp et al. 2015; Chew and DeBoy 2016), which has been associated with ADHD (van Ewijk et al. 2012).
We know of no study of the relationship between early postnatal inflammation and the risk of an attention disorder assessed at school age, nor do we know of any cohort study of the relationship between early blood concentrations of growth factors and the risk of an attention disorder assessed at school age. The ELGAN (Extremely Low Gestation Age Newborn) Study not only enrolled children born before the 28th week of gestation and followed them to age 10 years, but also measured concentrations of inflammation-related proteins in multiple blood specimens collected during the first postnatal month, as well as the concentrations of selected proteins that have neurotrophic and/or angiogenic properties. The ELGAN Study, therefore, provided an opportunity to begin to fill this void.
Methods
Participants
The ELGAN study is a multi-center prospective, observational study of the risk of structural and functional neurologic disorders in extremely preterm infants (O’Shea et al. 2009). A total of 1506 infants born before the 28th week of gestation were enrolled during the years 2002–2004.
At age 10 years, 966 of these children were recruited for an age-appropriate assessment of cognition, executive function, behaviors, and achievement. Of these, 889 (92%) returned for follow up and 874 were administered the neurocognitive tests. Enrollment and consent procedures for this follow up study were approved by the institutional review boards of all participating institutions.
General Cognitive Ability
General cognitive ability (or IQ) was assessed with the School-Age Differential Ability Scales–II (DAS-II) Verbal and Nonverbal Reasoning scales (Elliott 2007). Intellectual disability (ID) was defined as a score below 70 on both the verbal and nonverbal reasoning scales.
ADHD Assessment
While the child was tested, the parent or caregiver completed questionnaires regarding the child’s medical and neurological status and behavior, including the Child Symptom Inventory-4 Parent Checklist (CSI-4) (Sprafkin et al. 2002; Gadow and Sprafkin 2002). The child’s current teacher was also asked to complete the Child Symptom Inventory-4 Teacher Checklist. Teachers and parents did not make any ADD DSM-IV diagnosis. Rather, the CSI-4 program identified children as screening positive for these diagnoses based on the parents’ or teachers’ acknowledging selected characteristics of the child that aligned with DSM-IV symptoms.
Both the parent checklist and the teacher version include the same 18 items specific for ADHD symptoms (9 for the inattentive domain and 9 for the hyperactive/impulsive domain) that are each rated on a scale from 0 (never) to 3 (very often). For this study, a child was classified as screening positive for each ADHD type if s/he was reported to have 6 or more of the 9 characteristics/symptoms either, sometimes, or very often. Parents were the source about the physician’s diagnosis, which we recognize is often based, in part, on parent and teacher assessments (Power et al. 2008).
Blood Spot Collection and Measurement
Drops of blood were collected on filter paper on the first postnatal day (range: 1–3 days), the 7th postnatal day (range: 5–8 days), the 14th postnatal day (range: 12–15 days), the 21st postnatal day (range: 19–23 days), and the 28th postnatal day (range: 26–29). All blood was from the remainder of specimens obtained for clinical indications. Dried blood spots were stored at −70 °C in sealed bags with a desiccant until processed. Details about the elution of proteins from the blood spots are provided elsewhere.(Fichorova et al. 2015) The total protein concentration in each eluted sample was determined by BCA assay (Thermo Scientific, Rockford, IL) using a multi-label Victor 2 counter (Perkin Elmer, Boston, MA) and the measurements of each analyte normalized to mg total protein (Fichorova et al. 2015).
Proteins Measured
The Genital Tract Biology Laboratory at the Brigham and Women’s Hospital in Boston Massachusetts eluted all blood spots as previously described and measured all proteins reported here. The laboratory used the Meso Scale Discovery to measure: C-Reactive Protein (CRP), serum amyloid A (SAA), Myloeperoxidase (MPO) Interleukin-1 β (IL-1β), IL-6, IL-6 Receptor (IL-6R), Tumor Necrosis Factor-α (TNF-α), TNF Receptor-1 (TNFR-1), TNFR-2, IL-8 (CXCL8), Regulated upon Activation, Normal T-cell Expressed, and Secreted (RANTES; CCL5), Intercellular Adhesion Molecule −1 (ICAM-1; CD54), Vascular Cell Adhesion Molecule-1 VCAM-1; CD106), Vascular Endothelial Growth Factor (VEGF), VEGF Receptor-1 (VEGFR-1, also known as sFLT-1), VEGFR-2 (KDR), Insulin-Like Growth Factor-1 (IGF) Binding Protein-1 (IGFBP-1), thyroid stimulating hormone (TSH), Metalloproteinase (MMP)-9, and erythropoietin (EPO).
A multiplex immunobead assay manufactured by R&D Systems (Minneapolis, MN) and a MAGPIX Luminex reader (R&D Systems) were used to measure angiopoietin-1 (Ang-1), Ang-2, placenta growth factor (PIGF), Neurotrophin-4 (NT-4), Brain Derived Neurotrophic Factor (BDNF), and basic Fibroblastic Growth Factor (bFGF). ELISA (R&D Systems) was used to measure IGF-1.
Because the concentrations of inflammation-related proteins in the ELGAN Study varied with gestational age, and with the postnatal day of collection (Leviton et al. 2011b, 2012), we divided our sample into 15 groups defined by gestational age category (23–24, 25–26, 27 weeks), and postnatal day of blood collection (1, 7, 14, 21 and 28). Because we were interested in the contribution of both high and low concentrations, and the concentrations of most proteins did not follow a normal distribution, the distribution of each protein’s concentration was divided into quartiles among children in each of the 15 groups (3 gestational age groups, 5 collection days).
Data Analyses
In the sample of all children who had protein measurements and a CSI-4 form completed by a parent or teacher, 65 children had an IQ of less than 70. These children have been excluded because we did not have a way of determining if the ADHD symptoms were “inconsistent with developmental level” as recommended by Diagnostic and Statistical Manual of Mental Disorders, 4th Edition (DSM-IV).
We evaluated five null hypotheses linking blood concentrations during the first postnatal month to ADHD symptoms 10 years later. First, compared to children who had a concentration in the lower three quartiles one day, those who had a top quartile concentration that day were no more likely of satisfying CSI-4 criteria for ADHD at age 10 years (Appendix Tables 1-5, summarized in Table 2). Second, compared to their peers who had lower concentrations of a protein on two days of the early epoch (when specimens were obtained on days 1, 7, and 14), those who had top quartile concentrations of that protein on two days of the early epoch were no more likely to have ADHD symptoms at age 10 (Appendix Table 6, summarized in Table 2). Third, compared to their peers who had lower concentrations of a protein on both days 21 and 28 (of the late epoch), those who had top quartile concentrations of that protein on those days were no more likely to have ADHD symptoms at age 10 (Appendix Table 7, summarized in Table 2). Fourth, compared to their peers who had concentrations of two proteins in the lower three quartiles on two days during the early epoch, those who had higher concentrations of one or both proteins were not at higher risk of ADHD symptoms at age 10 (Table 3 for teacher-identified ADHD and Table 5 for parent-and-teacher-identified ADHD). Fifth, compared to their peers who had concentrations of two proteins in the lower three quartiles on both days of the late epoch, those who had higher concentrations of one or both proteins were not at higher risk of teacher-identified ADHD symptoms at 10 (Table 4).
We created logistic regression models comparing the risk of ADHD symptoms among children who had a concentration in the top quartile to the risk among children who had a concentration below the 75th centile. This allowed us to calculate odds ratios and 95% confidence intervals. In the two-protein sets of analyses (Tables 3, 4, and 5), children who did not have high (i.e., top quartile) concentrations of either protein were the referent group, and their odds ratios of ADHD are set at 1.0.
In this sample, children who had top quartile concentrations of inflammation-related proteins were no more likely than their peers who had lower concentrations to have a mother who had limited educational achievement, a low score on the Kaufman Brief Intelligence Test, 2nd Edition, or was eligible for government-provided medical care insurance (Medicaid) (Leviton et al. 2016b). Thus, confounding by social class is not an issue. Similarly, other potential confounders such as sex were also not associated with systemic inflammation. On the other hand, fetal growth restriction, defined as a birth weight Z-score < −1, is associated in this sample with elevated concentrations of some proteins, (Leviton et al. 2016b) and with ADHD symptoms (Korzeniewski et al. 2017). All analyses have been adjusted for gestational age 23–24 and 25–26 weeks as well as birth weight Z-score < −1.
We examined high and low concentrations of neuroprotective proteins in relation to high and low concentrations of the three inflammatory proteins (TNF-α, IL-8, and ICAM-1) most consistently associated with brain damage and/or adverse neurodevelopmental outcomes in the ELGAN study cohort. High concentrations of IL-8, and ICAM-1 were associated with enlarged cerebral ventricles (when the infant was in the intensive care nursery), and low mental development index (MDI) and microcephaly at age 2 years, (Leviton et al. 2016a) while high concentrations of TNF-alpha, IL-8, ICAM-1 were associated with diparetic cerebral palsy at age 2 years (Kuban et al. 2014) and low IQ at age 10 years (Kuban et al. 2017).
Results
Sample Description (Table 1)
Of the 864 children who provided two blood specimens a week apart during the first postnatal month and whose parent completed a CSI-4 when the child was 10 years old, 793 had an IQ ≥ 70. Of these children, the numbers identified as having ADHD symptoms were 151 by the parent, 128 by the teacher, 52 by both the parent and teacher, 181 by a physician, and 130 by any 2 observers.
Summary of Individual Proteins on Individual Days (Table 2)
The appendix contains 7 tables. Each of first 5 are for the concentrations on the day the blood was collected (i.e., days 1, 7, 14, 21, and 28), and the last 2 are for the early and late epochs. These tables contain 945 odds ratios for ADHD symptoms (27 proteins X 5 observers X 7 times). The findings are summarized in Table 2, which identifies the 40 statistically-significant instances of increased risk and the 9 instances of statistically-significant decreased risk.
By and large the associations summarized in this table are scattered by day, protein, and observer. The most consistent associations were for VEGF-family-associated increased risks for ADHD symptoms identified by the teacher, as well as by both teacher and parent.
Early Epoch Sets of Proteins: Teacher-Identified ADHD Symptoms (Table 3)
Because the associations in Table 2 are more abundant for AHDH symptoms identified by the teacher than by the parent, we limit additional analyses to screening positive according to the teacher. We attach the name “pattern A” to the pattern of a significantly elevated odds ratio of screening positive for ADHD when the concentrations of both proteins are in the top quartile. This pattern is seen when high concentrations of TNF-α occurred with high concentrations of MMP-9, EPO, VEGF, and Ang-1. It also occurred with high concentrations of both IL-8 and MMP-9.
“Pattern B” is characterized by increased risks of ADHD symptoms when a top quartile concentration of the neurotrophic protein is associated with increased risks of ADHD symptoms even when concentrations of the inflammation-related protein are low. This pattern was seen with IL-6R regardless of the concentrations of TNF-α, IL-8, and ICAM-1, and with VEGF-R2 regardless of the concentrations of these proteins.
Pattern C is characterized by significantly increased risk associated with high concentrations of the inflammation-related protein, regardless of the concentration of the neurotrophic protein. This pattern is seen with top quartile concentrations of IL-8 regardless of the concentration of IL-6R, EPO, and VEGF.
We assign the name Pattern D to the increased risk of ADHD symptoms associated with high concentrations of the inflammation-related protein, but only when concentrations of the neurotrophic protein are lower. This pattern was seen for the combinations of TNF-α with NT-4 and bFGF, and combinations of IL-8 with RANTES, VEGF-R1, VEGF-R2, NT-4, BDNF, bFGF, PIGF, Ang-1, and Ang-2. We identify this pattern as “protection in the face of adversity.”
Late Epoch Sets of Proteins: Teacher-Identified ADHD Symptoms: Late Epoch (Table 4)
Pattern D is seen with VEGF and IL-6R, RANTES, EPO, IGF-1, PIGF, and Ang-2, as well as with VEGF-R1 and EPO, and with VEGF-R2 and EPO, VEGF-R2 and Ang-2. Pattern A is seen with VEGF and MMP-9, VEGF-R1, bFGF, and Ang-1, as well as with VEGF-R1 and VEGF, and Ang-1, and with VEGF-R2 and IL-6R, RANTES, VEGF-R1, and PIGF. Pattern C was seen with VEGF and VEGF-R2, as well as with NT-4 and VEGF, VEGF-R1, and VEGF-R2, and with Ang-1 and VEGF, VEGF-R1, and VEGF-R2.
Early Epoch Sets of Proteins: Parent and Teacher-Identified ADHD Symptoms (Table 5)
Pattern C was seen with VEGF and the three inflammation-related proteins that head the columns (i.e., TNF-α, IL-8, and ICAM-1), as well as with NT-4 and IL-8. Pattern D was exemplified by top quartile concentrations of VEGF-R2, Ang-1, and Ang-2, but only in the setting of top quartile concentrations of IL-8.
Discussion
We found 40 instances of increased risk and 9 instances of decreased risk among 27 proteins assessed by 5 observers on 7 occasions (5 days and 2 epochs) = 945 non-independent opportunities. This fraction of 49/945 = 5%, leads us to infer that what we found might reflect random phenomena. On the other hand, the 20 statistically significant associations of the VEGF family (VEGF, VEGF-R1, and VEGF-R2) out of 105 opportunities (3 proteins X 5 observers X 7 times) raise the possibility that a biologic signal might exist among the noise. So does the finding that of 12 assessments for protection against adversities associated with high concentrations of IL-8 on 2 days during the early epoch, high concentrations of RANTES, VEGF-R1, NT-4, BDNF, bFGF, PIGF, Ang-1, and Ang-2 were associated with lower risk of teacher-identified ADHD symptoms than when the concentrations of these neurotrophic proteins were lower.
We identified four patterns when we evaluated the concentrations of two proteins together, one that is part of the inflammatory process and of with presumed neurotrophic and/or angiogenic properties. The A pattern, characterized by significantly elevated risk of screening positive for ADHD when the concentrations of both proteins are in the top quartile, might be the consequence of four situations. In one, high concentrations of both proteins are needed to cause damage. This might be possible when the neurotrophic protein has pleiotropic properties. For example, in the ELGAN Study cohort, high concentrations of EPO, a protein with unquestioned neurotrophic capabilities, (Castaneda-Arellano et al. 2014) are associated with inflammation to such an extent that they appear to be associated with increased risk of indicators of brain damage (Korzeniewski et al. 2015).
Another possibility is that damage results in the release of both proteins into the circulation and reflects not damage causation, but rather damage consequence. This inference is supported by observations that brain damage can be followed by increased concentrations peripherally of inflammation-related proteins, such as TNF, (Ross et al. 1994; Stover et al. 2000; Sotgiu et al. 2006; Sriram and O’Callaghan 2007; Wiseman et al. 2014) IL-6, (Wiseman et al. 2014) IL-8, (Whalen et al. 2000) ICAM-1, (Sotgiu et al. 2006; Wiseman et al. 2014) and MMP-9 (Sotgiu et al. 2006). Brain damage can also be followed by the release and/or upregulated expression of IGF-1, (Wildburger et al. 2001; Aberg et al. 2011; Mangiola et al. 2015) BDNF,(Yang et al. 1996; Korhonen et al. 1998; Kazanis et al. 2004; Rodier et al. 2015) bFGF, (Lin et al. 1997; Guo et al. 2006) NT-4, (Royo et al. 2006) VEGF, (Slevin et al. 2000; Shore et al. 2004; Matsuo et al. 2013) EPO, (Sakanaka et al. 1998; Juul et al. 1999; Brines et al. 2000; Aberg et al. 2016).
A third possibility is that high concentrations of one protein contribute to the damage, which then results in the release of the other protein. In the fourth, high concentrations of one protein contribute to the damage, which then promotes the synthesis of the other protein.
The B pattern of increased risks of ADHD symptoms when concentrations of the neurotrophic protein are high and the concentrations of the inflammation-related protein are lower was seen with three proteins thought to have neurotrophic properties, IL-6R, VEGFR-2, and NT-4. Each of these is characterized by pleiotropy (Tjwa et al. 2003; Kishimoto 2010; Bothwell 2014). Thus, it is possible, that the high concentrations of these proteins allow or contribute to the increased risk of screening positive for ADHD.
The C pattern, characterized by significantly increased risk associated with high concentrations of the inflammation-related protein, regardless of the concentration of the neurotrophic protein, can be viewed as what would be expected if the neurotrophic protein failed to protect against brain adversities associated with inflammation.
The D pattern of increased risk of screening positive for ADHD associated with high concentrations of the inflammation-related protein, but only when concentrations of the neurotrophic protein are lower is exactly what would be expected if the neurotrophic protein modulated inflammation-associated risk. It is this hope that has prompted the recommendation that growth factors (with neurotrophic properties) be considered therapeutic (Hassell et al. 2015; Larpthaveesarp et al. 2015).
Definition of ADHD Symptoms
The CSI-4 does not provide DSM-IV diagnoses. Rather it identifies children as screening positive for the inattentive and/or hyperactive/impulsive domains. Because of poor agreement between parent and teacher on these domains, and in light of concern about the validity (Bernfeld 2012), and the stability of subtypes (Lahey et al. 2005; Valo and Tannock 2010; Willcutt et al. 2012; Srebnicki et al. 2013), we chose to view ADHD symptoms as “an important unitary component to ADHD symptoms” (Toplak et al. 2009).
We acknowledge that the CSI-4 is not routinely used to assess the existence of ADHD symptoms (Chang et al. 2016). On the other hand, it has the advantage of providing information about other disorders that can influence manifestations of ADHD, or pose diagnostic problems (DeVincent and Gadow 2009; Ondrejka et al. 2010; Nazari et al. 2016).
Observers
The Diagnostic and Statistical Manual of Mental Disorders, Fifth Edition (DSM-V) requires “several inattentive or hyperactive-impulsive symptoms … in two or more settings” (American PA 2013), while The American Academy of Pediatrics (AAP) clinical practice ADHD guideline recommends that reports of behaviors be collected from a classroom teacher and from parents (American Academy of Pediatrics 2000).
The different profiles of high concentrations of proteins associated with parent-identified ADHD symptoms and teacher-identified symptoms adds to the literature about how teachers differ from parents in the behaviors they identify under the rubric of attention-related symptoms (Dirks et al. 2012). Having agreement between teacher and parent, however, did not increase the ability to detect an association between postnatal inflammation and ADHD symptoms.
Age 2 or Age 10; CBCL or CSI-4; Parent or Teacher
At age 2 years, an attention problem was defined on the basis of a Child Behavior Checklist (CBCL) completed by the parent or caregiver (O’Shea et al. 2014). Then the inflammation signal was comprised of top quartile concentrations on 2 of the 3 sampling days (days 1, 7, and 14) of MPO, IL-6, IL-8, ICAM-3, VEGF-R1, and VEGF-R2.
We do not view a parent-completed CBCL-defined attention problem at age 2 years as equivalent to one defined by a teacher-completed CSI-4. Three differences need to be emphasized, the age at assessment, the assessor, and the instrument.
The Importance of Context (or the Limits of Univariable Analyses)
RANTES, VEGF-R1, NT-4, BDNF, bFGF, PIGF, Ang-1 and Ang-2 are not identified in Table 2 as reducers of the risk of teacher-identified ADHD symptoms. In Table 3, however, each of these proteins displays a neurotrophic effect when the concentration of IL-8 is in the top quartile. Similarly, IL-8 is not identified in Table 2 as associated with increased risk of teacher-identified ADHD symptoms. In essence, evaluating the risk is limited when we have no sense of the context that might modify the risk. We strongly encourage that context be considered.
VEGF
The neurotrophic and angiogenic capabilities of VEGF and similar proteins are so interrelated that the term “angioneurin” was created to emphasize these dual sets of capabilities (Zacchigna et al. 2008). VEGF (Raab et al. 2004) and VEGFR-2 (Flk-1)(Jin et al. 2002; Sun et al. 2003) appear to be involved in neurogenesis, as well as repair of the brain after damage (Sun et al. 2003; Madri 2009). Of the multiple VEGF receptors found in CNS tissue, VEGF-2 appears to mediate almost all of the known cellular responses to VEGF. VEGF-R1 (Flt-1) is not normally expressed on mature neurons, but can be strongly upregulated after nerve crush injury (Islamov et al. 2004), and even transient CNS ischemia (Jin et al. 2001; Choi et al. 2007), suggesting a role in brain injury, and or repair.
Despite its neuroprotective effects, VEGF can exacerbate brain damage (Ma et al. 2012). Some of this exacerbation has been attributed to “the promotion of blood-brain barrier breakdown that inevitably accompanies vascular growth.” If this is the case, VEGF and VEGF-R2 can be seen not as primary agents of brain damage, but as contributors to secondary brain damage.
BDNF
Of all the neurotrophic proteins we evaluated, BDNF appears to have been the one most often considered a candidate for a role in the occurrence of ADHD (Shim et al. 2008; Scassellati et al. 2014; Liu et al. 2015). And yet, the data are conflicting, with some studies reporting higher peripheral concentrations (Yeom et al. 2016), and others failing to find any association (Scassellati et al. 2014; Zeni et al. 2016). Gene studies have also failed to produce a coherent relationship between BDNF-related genes and the risk of ADHD (Cho et al. 2010; Zeni et al. 2016).
We found only one instance (Table 3: Teacher-identified ADHD symptoms and early epoch protein concentrations with IL-8) among 9 opportunities for top-quartile BDNF concentrations to show a beneficial effect on the risk of ADHD symptoms, even when other proteins with neurotrophic capabilities did (e.g., NT-4, IGF-1, PIGF, as well as VEGF-R2, Ang-1, and Ang-2). We do not equate our assessment of BDNF concentrations shortly after birth with studies during childhood. Nevertheless, our failure to find a convincing relationship between top-quartile BDNF concentrations and ADHD symptoms can be viewed as adding support to the “no-association” side of the balance.
ICAM-1
On occasion, we have found that high concentrations of a protein thought to be capable of damaging the brain are not so identified when the concentrations of neurotrophic proteins are not known, but are so identified when the concentrations of neurotrophic proteins are known to be low. Because elevated concentrations of ICAM-1 have been associated with indicators of brain damage in our cohort (Leviton et al. 2011a; O’Shea et al. 2013; Leviton et al. 2016a), we reasoned that since ADHD does have brain structural correlates (Rubia et al. 2014), perhaps ICAM-1, which was not associated with increased risk of ADHD symptoms on univariable analyses, would be identified when evaluated in light of the concentrations of neurotrophins. We confirm that even under such scrutiny, high concentrations of ICAM-1 are not consistently or prominently associated with increased risk of teacher-identified ADHD symptoms.
Synthesis
Although we evaluated several individual proteins, we prefer to view each inflammation-related protein as a surrogate for many other proteins in its class (Zhao et al. 2016), and each neurotrophic protein as a surrogate for the class of proteins that possess neurotrophic characteristics (Oliveira et al. 2013). In addition, the more “mature” the newborn (along the developmental regulation schedule), the more likely s/he should be able to resolve inflammation, and minimize/repair damage (Clapp 2006; Zasada et al. 2014; van Tilborg et al. 2016).
The maturation level is likely to influence the synthesis of many proteins, even if not all to the same extent. Consequently, we urge caution in drawing inferences about any individual protein, and instead encourage seeing our data as indicating the need to evaluate multiple proteins in light of biomarkers of biologic processes that might moderate their effects.
Strengths and Limitations
Our analyses had sufficient power to detect as statistically significant odds ratios as low as 1.7 attests to our power, at least for the association of early epoch protein profiles with teacher identified ADHD symptoms. Other strengths include modest attrition, and protein measurements of high quality (Fichorova et al. 2008), and high content validity (Fichorova et al. 2011; Hecht et al. 2011; Leviton et al. 2011b; McElrath et al. 2011).
We measured a limited number of the many proteins involved in inflammation, a broad and complex phenomenon (Zak and Aderem 2009; Xiao et al. 2011). We are also limited by the small number of proteins measured, and inadequate power for assessments of ADHD symptoms by both parent and teacher.
We relied on blood specimens obtained for clinical indications. As they became more stable, healthier and more mature, infants were less likely than their sicker peers to have blood drawn on days 14, 21, and 28. Consequently, statistical power decreased and selection bias probably occurred.
Our bivariate orientation of two proteins simultaneously assumes that everything else is unimportant. Obviously, that is a severe limitation. The child classified as not exposed to a high concentrations of the inflammation-associated protein identified at the top of the column may very well be exposed to high concentrations of another, potentially very important, brain-damaging protein we did not measure. We acknowledge the complexity of inflammation and our inability to provide a useful measure of the severity of the (potentially damaging) exposure to inflammation.
We have limited our vision to the first postnatal month. In the ELGAN cohort, newborns whose placenta was inflamed were more likely to have systemic inflammation, but only on the first postnatal day.(Hecht et al. 2011) Similarly, disorders associated with placenta insufficiency were associated with low concentrations of growth factors in the newborn’s blood during the first postnatal day only (unpublished observations). Thus, the effects of inflammation and neurotrophic capabilities associated with maternal-fetal interactions might have had influences we could not identify (Hsiao and Patterson 2012; Instanes et al. 2017).
Conclusions
In this high-risk cohort of children born extremely preterm, systemic inflammation is associated with increased risk of ADHD symptoms, while high concentrations of proteins with neurotrophic properties appear able to modulate this increased risk.
References
Aberg D, Jood K, Blomstrand C, Jern C, Nilsson M, Isgaard J, Aberg ND (2011) Serum IGF-I levels correlate to improvement of functional outcome after ischemic stroke. J Clin Endocrinol Metab 96:E1055–E1064
Aberg ND, Stanne TM, Jood K, Schioler L, Blomstrand C, Andreasson U, Blennow K, Zetterberg H, Isgaard J, Jern C, Svensson J (2016) Serum erythropoietin and outcome after ischaemic stroke: a prospective study. BMJ Open 6:e009827
American PA (2013) Diagnostic and Statistical Manual of Mental Disorders, 5th Edition: DSM-5
American Academy of Pediatrics (2000) Clinical Practice Guideline. Diagnosis and evaluation of the child with attention-deficit/hyperactivity disorder. Pediatrics 105:1158–1170
Bernfeld J (2012) ADHD and factor analysis: are there really three distinct subtypes of ADHD? Appl Neuropsychol Child 1:100–104
Bothwell M (2014) NGF, BDNF, NT3, and NT4. Handb Exp Pharmacol 220:3–15
Brines ML, Ghezzi P, Keenan S, Agnello D, de Lanerolle NC, Cerami C, Itri LM, Cerami A (2000) Erythropoietin crosses the blood-brain barrier to protect against experimental brain injury. Proc Natl Acad Sci U S A 97:10526–10531
Castaneda-Arellano R, Beas-Zarate C, Feria-Velasco AI, Bitar-Alatorre EW, Rivera-Cervantes MC (2014) From neurogenesis to neuroprotection in the epilepsy: signalling by erythropoietin. Front Biosci (Landmark Ed) 19:1445–1455
Ceylan MF, Sener S, Bayraktar AC, Kavutcu M (2012) Changes in oxidative stress and cellular immunity serum markers in attention-deficit/hyperactivity disorder. Psychiatry Clin Neurosci 66:220–226
Chang LY, Wang MY, Tsai PS (2016) Diagnostic accuracy of rating scales for attention-deficit/hyperactivity disorder: a meta-analysis. Pediatrics 137:e20152749
Chew LJ, DeBoy CA (2016) Pharmacological approaches to intervention in hypomyelinating and demyelinating white matter pathology. Neuropharmacology 110:605–625
Cho SC, Kim HW, Kim BN, Kim JW, Shin MS, Chung S, Cho DY, Jung SW, Yoo HJ, Chung IW, Chung US, Son JW (2010) Gender-specific association of the brain-derived neurotrophic factor gene with attention-deficit/hyperactivity disorder. Psychiatry Investig 7:285–290
Choi JS, Kim HY, Cha JH, Choi JY, Chun MH, Lee MY (2007) Upregulation of vascular endothelial growth factor receptors Flt-1 and Flk-1 in rat hippocampus after transient forebrain ischemia. J Neurotrauma 24:521–531
Clapp DW (2006) Developmental regulation of the immune system. Semin Perinatol 30:69–72
Dammann O, Leviton A (1999) Brain damage in preterm newborns: might enhancement of developmentally-regulated endogenous protection open a door for prevention? Pediatrics 104:541–550
Dammann O, Leviton A (2014) Intermittent or sustained systemic inflammation and the preterm brain. Pediatr Res 75(3):376–380
Dammann O, Allred EN, Fichorova RN, Kuban K, O’Shea TM, Leviton A (2016) Duration of systemic inflammation in the first postnatal month among infants born before the 28th week of gestation. Inflammation 39:672–677
DeVincent CJ, Gadow KD (2009) Relative clinical utility of three child symptom Inventory-4 scoring algorithms for differentiating children with autism spectrum disorder vs. attention-deficit hyperactivity disorder. Autism Res 2:312–321
Dirks MA, De Los RA, Briggs-Gowan M, Cella D, Wakschlag LS (2012) Annual research review: embracing not erasing contextual variability in children’s behavior--theory and utility in the selection and use of methods and informants in developmental psychopathology. J Child Psychol Psychiatry 53:558–574
Elliott CD (2007) Differential ability scales, 2nd edn. Pearson, San Antonio
Fichorova RN et al (2008) Biological and technical variables affecting immunoassay recovery of cytokines from human serum and simulated vaginal fluid: a multicenter study. Anal Chem 80:4741–4751
Fichorova RN, Onderdonk AB, Yamamoto H, Delaney ML, DuBois AM, Allred E, Leviton A, (2011) Microbe-specific modulation of inflammatory response in extremely low gestational age newborns. mBio 2:e00280–e00210
Fichorova RN, Beatty N, Sassi RRS, Yamamoto HS, Allred EN, Leviton A, for the ELGAN Study Investigators (2015) Systemic inflammation in the extremely low gestational age newborn following maternal genitourinary infections. Am J Reprod Immunol 73:162–174
Gadow KD, Sprafkin J (2002) Child symptom Inventory–4 screening and norms Manual. Checkmate Plus, Stony Brook
Guo H, Huang L, Cheng M, Jin X, Zhao Y, Yi M (2006) Serial measurement of serum basic fibroblast growth factor in patients with acute cerebral infarction. Neurosci Lett 393:56–59
Hassell KJ, Ezzati M, Alonso-Alconada D, Hausenloy DJ, Robertson NJ (2015) New horizons for newborn brain protection: enhancing endogenous neuroprotection. Arch Dis Child Fetal Neonatal Ed 100:F541–F552
Hecht JL, Fichorova RN, Tang VF, Allred EN, McElrath TF, Leviton A (2011) Relationship between neonatal blood protein profiles and placenta histologic characteristics in ELGANs. Pediatr Res 69:68–73
Holtmann M, Poustka L, Zepf FD, Banaschewski T, Priller J, Bolte S, Legenbauer T (2013) Severe affective and behavioral dysregulation in youths is associated with a proinflammatory state. Zeitschrift fur Kinder- und Jugendpsychiatrie und Psychotherapie 41:393–399
Hsiao EY, Patterson PH (2012) Placental regulation of maternal-fetal interactions and brain development. Dev Neurobiol 72:1317–1326
Instanes JT, Halmoy A, Engeland A, Haavik J, Furu K, Klungsoyr K (2017) Attention-deficit/hyperactivity disorder in offspring of mothers with inflammatory and immune system diseases. Biol Psychiatry 81:452–459
Islamov RR, Chintalgattu V, Pak ES, Katwa LC, Murashov AK (2004) Induction of VEGF and its Flt-1 receptor after sciatic nerve crush injury. Neuroreport 15:2117–2121
Jin K, Mao XO, Eshoo MW, Nagayama T, Minami M, Simon RP, Greenberg DA (2001) Microarray analysis of hippocampal gene expression in global cerebral ischemia. Ann Neurol 50:93–103
Jin K, Zhu Y, Sun Y, Mao XO, Xie L, Greenberg DA (2002) Vascular endothelial growth factor (VEGF) stimulates neurogenesis in vitro and in vivo. Proc Natl Acad Sci U S A 99:11946–11950
Juul SE, Stallings SA, Christensen RD (1999) Erythropoietin in the cerebrospinal fluid of neonates who sustained CNS injury. Pediatr Res 46:543–547
Kazanis I, Giannakopoulou M, Philippidis H, Stylianopoulou F (2004) Alterations in IGF-I, BDNF and NT-3 levels following experimental brain trauma and the effect of IGF-I administration. Exp Neurol 186:221–234
Kishimoto T (2010) IL-6: from its discovery to clinical applications. Int Immunol 22:347–352
Korhonen L, Riikonen R, Nawa H, Lindholm D (1998) Brain derived neurotrophic factor is increased in cerebrospinal fluid of children suffering from asphyxia. Neurosci Lett 240:151–154
Korzeniewski SJ, Allred E, Logan JW, Fichorova RN, Engelke S, Kuban KC, O’Shea TM, Paneth N, Holm M, Dammann O, Leviton A (2015) Elevated endogenous erythropoietin concentrations are associated with increased risk of brain damage in extremely preterm neonates. PLoS One 10:e0115083
Korzeniewski S, Allred E, Joseph R, Heeren T, Kuban K, O’Shea T, Leviton A, investigators. ftEs (2017) Correlates at age 10 years of fetal-growth-restriction among children who were born before the 28th week ofgestation (in press)
Kuban KC, O’Shea TM, Allred EN, Paneth N, Hirtz D, Fichorova RN, Leviton A (2014) Systemic inflammation and cerebral palsy risk in extremely preterm infants. J Child Neurol 29:1692–1698
Kuban KC, Joseph RM, O’Shea TM, Heeren T, Fichorova RN, Douglass L, Jara H, Frazier JA, Hirtz D, Rollins JV, Paneth N (2017) Circulating inflammatory-associated proteins in the first month of life and cognitive impairment at age 10 years in children born extremely preterm. J Pediatr 180:116–123.e1
Lahey BB, Pelham WE, Loney J, Lee SS, Willcutt E (2005) Instability of the DSM-IV subtypes of ADHD from preschool through elementary school. Arch Gen Psychiatry 62:896–902
Larpthaveesarp A, Ferriero DM, Gonzalez FF (2015) Growth factors for the treatment of ischemic brain injury (growth factor treatment). Brain Sci 5:165–177
Leviton A, Kuban K, O’Shea TM, Paneth N, Fichorova R, Allred EN, Dammann O (2011a) The relationship between early concentrations of 25 blood proteins and cerebral white matter injury in preterm newborns: the ELGAN study. J Pediatr 158:897–903.e895
Leviton A, Fichorova R, Yamamoto Y, Allred EN, Dammann O, Hecht J, Kuban K, McElrath T, O’Shea TM, Paneth N (2011b) Inflammation-related proteins in the blood of extremely low gestational age newborns. The contribution of inflammation to the appearance of developmental regulation. Cytokine 53:66–73
Leviton A, Allred EN, Yamamoto H, Fichorova RN, Investigators. ftES (2012) Relationships among the concentrations of 25 inflammation-associated proteins during the first postnatal weeks in the blood of infants born before the 28th week of gestation. Cytokine 57(1):182–90
Leviton A, Allred EN, Fichorova RN, Kuban KC, Michael O’Shea T, Dammann O (2016a) Systemic inflammation on postnatal days 21 and 28 and indicators of brain dysfunction 2years later among children born before the 28th week of gestation. Early Hum Dev 93:25–32
Leviton A, Allred EN, Fichorova RN, Kuban KC, O’Shea TM, Dammann O (2016b) Antecedents of inflammation biomarkers in preterm newborns on days 21 and 28. Acta Paediatr 105:274–280
Lin TN, Te J, Lee M, Sun GY, Hsu CY (1997) Induction of basic fibroblast growth factor (bFGF) expression following focal cerebral ischemia. Brain Res Mol Brain Res 49:255–265
Liu DY, Shen XM, Yuan FF, Guo OY, Zhong Y, Chen JG, Zhu LQ, Wu J (2015) The physiology of BDNF and its relationship with ADHD. Mol Neurobiol 52:1467–1476
Ma Y, Zechariah A, Qu Y, Hermann DM (2012) Effects of vascular endothelial growth factor in ischemic stroke. J Neurosci Res 90:1873–1882
Madri JA (2009) Modeling the neurovascular niche: implications for recovery from CNS injury. J Physiol Pharmacol 60(Suppl 4):95–104
Mangiola A, Vigo V, Anile C, De Bonis P, Marziali G, Lofrese G (2015) Role and importance of IGF-1 in traumatic brain injuries. Biomed Res Int 2015:736104
Matsuo R, Ago T, Kamouchi M, Kuroda J, Kuwashiro T, Hata J, Sugimori H, Fukuda K, Gotoh S, Makihara N, Fukuhara M, Awano H, Isomura T, Suzuki K, Yasaka M, Okada Y, Kiyohara Y, Kitazono T (2013) Clinical significance of plasma VEGF value in ischemic stroke - research for biomarkers in ischemic stroke (REBIOS) study. BMC Neurol 13:32
McElrath TF, Fichorova RN, Allred EN, Hecht JL, Ismail MA, Yuan H, Leviton A (2011) Blood protein profiles of infants born before 28 weeks differ by pregnancy complication. Am J Obstet Gynecol 204:418.e411–418.e412
Mitchell RH, Goldstein BI (2014) Inflammation in children and adolescents with neuropsychiatric disorders: a systematic review. J Am Acad Child Adolesc Psychiatry 53:274–296
Nazari MA, Mirloo MM, Rezaei M, Soltanlou M (2016) Emotional stimuli facilitate time perception in children with attention-deficit/hyperactivity disorder. J Neuropsychol. doi:10.1111/jnp.12111
O’Shea TM, Allred EN, Dammann O, Hirtz D, Kuban KC, Paneth N, Leviton A (2009) The ELGAN study of the brain and related disorders in extremely low gestational age newborns. Early Hum Dev 85:719–725
O’Shea TM, Shah B, Allred EN, Fichorova RN, Kuban KC, Dammann O, Leviton A (2013) Inflammation-initiating illnesses, inflammation-related proteins, and cognitive impairment in extremely preterm infants. Brain Behav Immun 29:104–112
O’Shea TM, Joseph RM, Kuban KC, Allred EN, Ware J, Coster T, Fichorova RN, Dammann O, Leviton A (2014) Elevated blood levels of inflammation-related proteins are associated with an attention problem at age 24 mo in extremely preterm infants. Pediatr Res 75:781–787
Oliveira SL, Pillat MM, Cheffer A, Lameu C, Schwindt TT, Ulrich H (2013) Functions of neurotrophins and growth factors in neurogenesis and brain repair. Cytometry A 83:76–89
Ondrejka I, Abali O, Paclt I, Gacser M, Iftene F, Walton R, Harrison G, Treuer T, Martenyi F (2010) A prospective observational study of attention-deficit/hyperactivity disorder in central and Eastern Europe and Turkey: symptom severity and treatment options in a paediatric population. Int J Psychiatry Clin Pract 14:116–126
Power TJ, Mautone JA, Manz PH, Frye L, Blum NJ (2008) Managing attention-deficit/hyperactivity disorder in primary care: a systematic analysis of roles and challenges. Pediatrics 121:e65–e72
Raab S, Beck H, Gaumann A, Yuce A, Gerber HP, Plate K, Hammes HP, Ferrara N, Breier G (2004) Impaired brain angiogenesis and neuronal apoptosis induced by conditional homozygous inactivation of vascular endothelial growth factor. Thromb Haemost 91:595–605
Rand KM, Austin NC, Inder TE, Bora S, Woodward LJ (2016) Neonatal infection and later neurodevelopmental risk in the very preterm infant. J Pediatr 170:97–104
Reuss ML, Paneth N, Susser M (1994) Does the loss of placental hormones contribute to neurodevelopmental disabilities in preterm infants? Dev Med Child Neurol 36:743–747
Rodier M, Quirie A, Prigent-Tessier A, Bejot Y, Jacquin A, Mossiat C, Marie C, Garnier P (2015) Relevance of post-stroke circulating BDNF levels as a prognostic biomarker of stroke outcome. Impact of rt-PA treatment. PLoS One 10:e0140668
Ross SA, Halliday MI, Campbell GC, Byrnes DP, Rowlands BJ (1994) The presence of tumour necrosis factor in CSF and plasma after severe head injury. Br J Neurosurg 8:419–425
Royo NC, Conte V, Saatman KE, Shimizu S, Belfield CM, Soltesz KM, Davis JE, Fujimoto ST, McIntosh TK (2006) Hippocampal vulnerability following traumatic brain injury: a potential role for neurotrophin-4/5 in pyramidal cell neuroprotection. Eur J Neurosci 23:1089–1102
Rubia K, Alegria AA, Brinson H (2014) Brain abnormalities in attention-deficit hyperactivity disorder: a review. Rev Neurol 58(Suppl 1):S3–16
Sakanaka M, Wen TC, Matsuda S, Masuda S, Morishita E, Nagao M, Sasaki R (1998) In vivo evidence that erythropoietin protects neurons from ischemic damage. Proc Natl Acad Sci U S A 95:4635–4640
Scassellati C, Zanardini R, Tiberti A, Pezzani M, Valenti V, Effedri P, Filippini E, Conte S, Ottolini A, Gennarelli M, Bocchio-Chiavetto L (2014) Serum brain-derived neurotrophic factor (BDNF) levels in attention deficit-hyperactivity disorder (ADHD). Eur Child Adolesc Psychiatry 23:173–177
Shim SH, Hwangbo Y, Kwon YJ, Jeong HY, Lee BH, Lee HJ, Kim YK (2008) Increased levels of plasma brain-derived neurotrophic factor (BDNF) in children with attention deficit-hyperactivity disorder (ADHD). Prog Neuro-Psychopharmacol Biol Psychiatry 32:1824–1828
Shore PM, Jackson EK, Wisniewski SR, Clark RS, Adelson PD, Kochanek PM (2004) Vascular endothelial growth factor is increased in cerebrospinal fluid after traumatic brain injury in infants and children. Neurosurgery 54:605–611 discussion 611-602
Shyu CS, Lin HK, Lin CH, Fu LS (2012) Prevalence of attention-deficit/hyperactivity disorder in patients with pediatric allergic disorders: a nationwide, population-based study. J Microbiol Immunol Infec 45:237–242
Slevin M, Krupinski J, Slowik A, Kumar P, Szczudlik A, Gaffney J (2000) Serial measurement of vascular endothelial growth factor and transforming growth factor-beta1 in serum of patients with acute ischemic stroke. Stroke 31:1863–1870
Sotgiu S, Zanda B, Marchetti B, Fois ML, Arru G, Pes GM, Salaris FS, Arru A, Pirisi A, Rosati G (2006) Inflammatory biomarkers in blood of patients with acute brain ischemia. Eur J Neurol 13:505–513
Sprafkin J, Gadow KD, Salisbury H, Schneider J, Loney J (2002) Further evidence of reliability and validity of the child symptom Inventory-4: parent checklist in clinically referred boys. J Clin Child Adolesc Psychol 31:513–524
Srebnicki T, Kolakowski A, Wolanczyk T (2013) Adolescent outcome of child ADHD in primary care setting: stability of diagnosis. J Atten Disord 17:655–659
Sriram K, O’Callaghan JP (2007) Divergent roles for tumor necrosis factor-alpha in the brain. J Neuroimmune Pharmacol 2:140–153
Stover JF, Schoning B, Beyer TF, Woiciechowsky C, Unterberg AW (2000) Temporal profile of cerebrospinal fluid glutamate, interleukin-6, and tumor necrosis factor-alpha in relation to brain edema and contusion following controlled cortical impact injury in rats. Neurosci Lett 288:25–28
Sucksdorff M, Lehtonen L, Chudal R, Suominen A, Joelsson P, Gissler M, Sourander A (2015) Preterm birth and poor fetal growth as risk factors of attention-deficit/ hyperactivity disorder. Pediatrics 136:e599–e608
Sun Y, Jin K, Xie L, Childs J, Mao XO, Logvinova A, Greenberg DA (2003) VEGF-induced neuroprotection, neurogenesis, and angiogenesis after focal cerebral ischemia. J Clin Invest 111:1843–1851
Tjwa M, Luttun A, Autiero M, Carmeliet P (2003) VEGF and PlGF: two pleiotropic growth factors with distinct roles in development and homeostasis. Cell Tissue Res 314:5–14
Toplak ME, Pitch A, Flora DB, Iwenofu L, Ghelani K, Jain U, Tannock R (2009) The unity and diversity of inattention and hyperactivity/impulsivity in ADHD: evidence for a general factor with separable dimensions. J Abnorm Child Psychol 37:1137–1150
Valo S, Tannock R (2010) Diagnostic instability of DSM-IV ADHD subtypes: effects of informant source, instrumentation, and methods for combining symptom reports. J Clin Child Adolesc Psychol 39:749–760
van Ewijk H, Heslenfeld DJ, Zwiers MP, Buitelaar JK, Oosterlaan J (2012) Diffusion tensor imaging in attention deficit/hyperactivity disorder: a systematic review and meta-analysis. Neurosci Biobehav Rev 36:1093–1106
van Tilborg E, Heijnen CJ, Benders MJ, van Bel F, Fleiss B, Gressens P, Nijboer CH (2016) Impaired oligodendrocyte maturation in preterm infants: potential therapeutic targets. Prog Neurobiol 136:28–49
Whalen MJ, Carlos TM, Kochanek PM, Wisniewski SR, Bell MJ, Clark RS, DeKosky ST, Marion DW, Adelson PD (2000) Interleukin-8 is increased in cerebrospinal fluid of children with severe head injury. Crit Care Med 28:929–934
Wildburger R, Zarkovic N, Leb G, Borovic S, Zarkovic K, Tatzber F (2001) Post-traumatic changes in insulin-like growth factor type 1 and growth hormone in patients with bone fractures and traumatic brain injury. Wien Klin Wochenschr 113:119–126
Willcutt EG, Nigg JT, Pennington BF, Solanto MV, Rohde LA, Tannock R, Loo SK, Carlson CL, McBurnett K, Lahey BB (2012) Validity of DSM-IV attention deficit/hyperactivity disorder symptom dimensions and subtypes. J Abnorm Psychol 121:991–1010
Wiseman S, Marlborough F, Doubal F, Webb DJ, Wardlaw J (2014) Blood markers of coagulation, fibrinolysis, endothelial dysfunction and inflammation in lacunar stroke versus non-lacunar stroke and non-stroke: systematic review and meta-analysis. Cerebrovasc Dis 37:64–75
Xiao W et al (2011) A genomic storm in critically injured humans. J Exp Med 208:2581–2590
Yang K, Perez-Polo JR, Mu XS, Yan HQ, Xue JJ, Iwamoto Y, Liu SJ, Dixon CE, Hayes RL (1996) Increased expression of brain-derived neurotrophic factor but not neurotrophin-3 mRNA in rat brain after cortical impact injury. J Neurosci Res 44:157–164
Yeom CW, Park YJ, Choi SW, Bhang SY (2016) Association of peripheral BDNF level with cognition, attention and behavior in preschool children. Child Adolesc Psychiat Ment Health 10:10
Zacchigna S, Lambrechts D, Carmeliet P (2008) Neurovascular signalling defects in neurodegeneration. Nat Rev Neurosci 9:169–181
Zak DE, Aderem A (2009) Systems biology of innate immunity. Immunol Rev 227:264–282
Zasada M, Kwinta P, Durlak W, Bik-Multanowski M, Madetko-Talowska A, Pietrzyk JJ (2014) Development and maturation of the immune system in preterm neonates: results from a whole genome expression study. Biomed Res Int 2014:498318
Zayats T, Athanasiu L, Sonderby I, Djurovic S, Westlye LT, Tamnes CK, Fladby T, Aase H, Zeiner P, Reichborn-Kjennerud T, Knappskog PM, Knudsen GP, Andreassen OA, Johansson S, Haavik J (2015) Genome-wide analysis of attention deficit hyperactivity disorder in Norway. PLoS One 10:e0122501
Zeni CP, Tramontina S, Aguiar BW, Salatino-Oliveira A, Pheula GF, Sharma A, Stertz L, Moreira Maia CR, Hutz M, Kapczinski FP, Rohde LA (2016) BDNF Val66Met polymorphism and peripheral protein levels in pediatric bipolar disorder and attention-deficit/hyperactivity disorder. Acta Psychiatr Scand 134:268–274
Zhao Y, Forst CV, Sayegh CE, Wang IM, Yang X, Zhang B (2016) Molecular and genetic inflammation networks in major human diseases. Mol BioSyst 12:2318–2341
Acknowledgements
This study was supported by The National Institute of Neurological Disorders and Stroke (5U01NS040069-05; 2R01NS040069-06A2), The National Eye Institute (1-R01-EY021820-01), and the National Institute of Child Health and Human Development (5P30HD018655-34).
The authors gratefully acknowledge the contributions of their subjects and their subjects’ families, as well as the contributions of their colleagues.
Participating Institutions and ELGAN Study Collaborators Who Made this Report Possible
Baystate Medical Center, Springfield MA (Bhavesh Shah, Karen Christianson)
Beth Israel Deaconess Medical Center, Boston MA (Camilia R. Martin, Colleen Hallisey, Caitlin Hurley, Miren Creixell)
Brigham & Women’s Hospital, Boston MA (Linda J. Van Marter)
Boston Children’s Hospital, Boston MA (Alan Leviton, Kathleen Lee, Anne McGovern, Elizabeth Allred, Jill Gambardella, Susan Ursprung, Ruth Blomquist)
Massachusetts General Hospital, Boston MA (Robert Insoft, Jennifer G. Wilson, Maureen Pimental)
New England Medical Center, Boston MA (Cynthia Cole, John Fiascone, Janet Madden, Ellen Nylen, Anne Furey)
U Mass Memorial Health Center, Worcester, MA (Francis Bednarek [deceased], Mary Naples, Beth Powers)
Yale-New Haven Hospital, New Haven CT (Richard Ehrenkranz, Joanne Williams, Elaine Romano)
Forsyth Hospital, Baptist Medical Center, Winston-Salem NC (T. Michael O’Shea, Debbie Gordon, Teresa Harold, Gail Hounsell, Debbie Hiatt)
University Health Systems of Eastern Carolina, Greenville NC (Stephen Engelke, Sherry Moseley, Linda Pare, Donna Smart, Joan Wilson)
North Carolina Children’s Hospital, Chapel Hill NC (Carl Bose, Gennie Bose, Janice Wereszczak)
DeVos Children’s Hospital, Grand Rapids MI (Mariel Portenga, Dinah Sutton)
Sparrow Hospital, Lansing MI (Padmani Karna, Carolyn Solomon)
University of Chicago Hospital, Chicago IL (Michael D. Schreiber, Grace Yoon)
William Beaumont Hospital, Royal Oak MI (Daniel Batton, Beth Kring)
Author information
Authors and Affiliations
Consortia
Corresponding author
Ethics declarations
Conflict of Interest Statement
The authors report no conflict of interest, financial or otherwise.
Electronic supplementary material
ESM 1
(DOCX 109 kb)
Rights and permissions
About this article
Cite this article
Allred, E.N., Dammann, O., Fichorova, R.N. et al. Systemic Inflammation during the First Postnatal Month and the Risk of Attention Deficit Hyperactivity Disorder Characteristics among 10 year-old Children Born Extremely Preterm. J Neuroimmune Pharmacol 12, 531–543 (2017). https://doi.org/10.1007/s11481-017-9742-9
Received:
Accepted:
Published:
Issue Date:
DOI: https://doi.org/10.1007/s11481-017-9742-9