Abstract
Aspergillus terreus, isolated from rotting bagasse, showed comparable cellulolytic activities when grown either in the free or immobilized states with cellulose as the sole carbon source. The cultural and nutritional requirements for maximum cellulase production by the organism either in the free or immobilized states were similar, except an increase in the temperature optimum from 30 to 40°C, occurred upon immobilization. In the free state, the maximum filter paper hydrolase, carboxymethylcellulase and β-glucosidase activities produced were 2.1, 13.6, and 3.2 U/ml, respectively, while in the immobilized state, the levels were 1.8, 12.0, and 2.4 U/ml. Production of cellulolytic enzymes by immobilized cells was influenced by the surface area of the support material. In addition, cells in the immobilized state sustained enzyme production for a much longer period with a 4.5-fold increase in productivity during repeated batch when compared to free cells.
Similar content being viewed by others
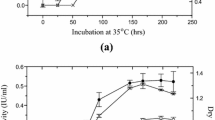
Avoid common mistakes on your manuscript.
Introduction
Enzymic hydrolysis of cellulosics, the most abundant renewable resource on earth, offers an attractive alternative for the generation of sugars which can serve as the raw materials for the production of various products of commercial interest such as ethanol (Qu et al. 2006), organic acids (Shen and Xia 2006; Lee et al. 2004) and other chemicals (Cao et al. 1997), if the process can be made economically competitive. As a consequence, much research effort has been directed towards improving the production of cellulolytic enzymes, which accounts for large fraction of the cost of producing glucose by this route (Ryu and Mandels 1980; Szijarto et al. 2004). A number of approaches has been adopted, aiming towards reducing the cost of enzyme production. These have included the use industrial wastes such as sawdust hydrolysate (Lo et al. 2005), corn cob (Liming and Xueliang 2000), bagasse (Bigelow and Wyman 2002) and agricultural waste (Jiang et al. 1998) as examples of low-cost materials which have been successfully used as substrates for cellulase fermentation by organisms such as Trichoderma reesei.
The use of immobilized cells for cellulase production has also been studied. Cellulolytic organisms immobilized by physical entrapment have been shown to have desirable characteristics such as prolonged enzyme production, better heat stability, lower substrate consumption and higher productivity in studies on T. reesei (Turker and Mavituna 1987), Sporotrichum cellulophilum (Tamada et al. 1986) and Trichoderma viride (Webb et al. 1986). In addition, the use of immobilized Aspergillus niger in bubble column bioreactors (Kang et al. 1995) and in biofilms (Villena and Gutierrez-Correa 2006) has been shown to have higher productivities compared to free cells. It is therefore conceivable that the use of immobilized organisms may offer a solution towards a reduction in the cost of producing cellulase.
This paper reports on the investigations carried out to optimize the production of cellulase by Aspergillus terreus under both free and immobilized on nylon pads as the support material as well as the production of cellulase over an extended period of time.
Materials and methods
Isolation of cellulolytic microorganisms
Aspergillus terreus was isolated from rotting bagasse by an enrichment technique in 100-ml Erlenmeyer flasks containing 20 ml of medium as described by Sternberg (1976) with 1% (w/v) cellulose as the sole carbon source. Incubation was carried out in an orbital shaker at 30°C and 200 rev/min. After five serial transfers, the cultures were streaked on nutrient agar plates and single colonies obtained purified by further plating. A. terreus spores from potato dextrose plates harvested by swirling in a small amount of sterile distilled water on the plates and used as inoculum. For maintenance purpose, the organism was stored as spores at −20°C in 20% (v/v) glycerol.
Immobilization and cultivation of microorganism
Cells were immobilized on woven nylon pads (domestic scouring pads) cut into 1 cm squares. The nylon pieces were boiled in 5% EDTA and washed in distilled water prior to being sterilized in 250-ml conical flasks containing 50 ml of basal medium. Basal medium (1 l) contains 10 ml of KH2PO4 (20%), 10 ml of MgSO4·7H2O (3%), 1 ml of CaCl2 (30%), 1 ml of FeSO4·7H2O (0.5%), 1 ml of MnSO4·4H2O (1.6%), 1 ml of ZnSO4·7H2O (1.4%), 2.0% of CoCl2, 0.075% of peptone, 0.2% of Tween 80, 1.0% of NaNO3, 1.0% of cellulose (Sigmacell Type 20) and enough distilled water to realize a final volume of 1 l. Unless otherwise stated, each flask contained four 1 cm squares. Spores, which were inoculated directly into the medium at a concentration of 2 × 105/ml, were used as the inoculum. The medium described above was used as the basal medium for the optimization of nutritional and cultural requirements of both free and immobilized cells. The parameters optimized were (1) the concentration of cellulose, the carbon source, (2) the type and concentration of inorganic and organic nitrogen sources, (3) pH of the medium and (4) the incubation temperature.
Free cells, when cultured under repeated batch conditions, were pelleted by centrifuging (5,000g, 5 min, room temperature) prior to resuspension in fresh medium, added to the original volume. Immobilized cells were aseptically filtered on Whatman No. 1 paper and then introduced into flasks containing fresh medium. Two replicates were prepared for each culture medium.
Assay of enzyme activities
Filter paper hydrolase (FPase) and carboxymethylcellulase (CMCase) activities were assayed as described by Mandels et al. (1976) at pH 5.0 and 50°C (60 min for FPase and 30 min for CMCase). Reducing sugars produced were assayed as described by Miller (1959) using glucose as the standard.
β-Glucosidase (EC 3.2.1.21) activity was assayed according to Desrochers et al. (1981) at pH 5.0 and 50°C for 30 min. Glucose produced was assayed enzymically using a glucose diagnostic kit (Sigma Diagnostic Kit, No. 315).
Enzyme activity (U) was expressed either as μmol glucose (for β-glucosidase) or glucose equivalent (for FPase and CMCase) liberated per min.
Results and discussion
Aspergillus terreus produced cellulolytic enzymes when grown in the basal medium. An enhancement of enzyme production occurred when the composition of the medium was modified with respect to its cellulose concentration and nitrogen source. The optimum cellulose concentration for maximum enzyme production by both free and immobilized cells was 1% (w/v), above which production was inhibited (Fig. 1). Similar observations on the effect of cellulose on enzyme production by cellulolytic organisms has been reported (Sternberg 1976). In free cells, the reduction in enzyme titers at increased cellulose concentrations has been attributed to either the repression of enzyme synthesis or inhibition of enzyme activity in the presence of high glucose concentrations resulting from substrate hydrolysis (Stutzenberger 1971; Sternberg 1976). It has also been suggested that the binding of the enzymes produced to the insoluble substrate could lead to the reduction of enzyme activity in the culture medium (Peitersen 1977). While all of the above could also hold true for immobilized cells, the decrease in the effective available space for mycelial growth within the matrix at increased cellulose concentrations, as suggested by Tamada et al. (1986), have to be considered. This is supported in the present studies where enzyme production by immobilized cells was found to be related to the amount of support material used during cultivation (Fig. 2). In the presence of the support material, growth was restricted to the matrix of the support with little visible growth occurring as free mycelium.
The effect of a series of nitrogen sources, all present at a concentration of 0.5 wt%-N, on cellulase production by both free and immobilized cells showed that A. terreus has a preference for NaNO3 under both culture conditions (Table 1). The optimum concentration for maximum enzyme production by both free and immobilized cells was 1.0 wt%-N. Such a preferential requirement has been shown in other cellulolytic organisms such as Penicillium pinophilum (Brown et al. 1987) and indeed NaNO3 was the preferred nitrogen source in organisms such as Sporotrichum thermophile (Coutts and Smith 1976). In S. thermophile, it was suggested that the preference for NaNO3 was due to it being a neutral salt which, unlike NH4 + salts, did not contribute to the acidification of the medium upon its utilization (Coutts and Smith 1976). Addition of various organic nitrogen sources (all at a concentration of 0.1 wt%-N) to cultures already optimized for NaNO3, further resulted in varying degrees of enhancement in enzyme production. The best organic nitrogen for free and immobilized cells were casein hydrolysate and bactopeptone, optimum at a concentration of 0.2 and 0.5 wt%-N, respectively. As such, the culture medium was accordingly modified to contain (1%) the relevant nitrogen sources at their respective optimal concentrations.
A comparison of the effect of temperature on enzyme production showed that immobilized cells had an increased temperature optimum when compared to free cells (Fig. 3). Similar observations was reported by Tamada et al. (1986) where Sporotrichum cellulophilum, immobilized on a non-woven material, exhibited a higher temperature tolerance compared to free cells. It could only be suggested that this observation was in effect due to the greater stability of the cells conferred by the immobilization matrix. Increased temperature tolerance of cells or enzymes immobilized by physical entrapment has been observed where, the greater stability was attributed to the support provided by the matrix (Chibata et al. 1986; Cheetham 1983). However, immobilization did not cause a major change in the effect of pH on enzyme production compared to free cells (Fig. 4). The pH optimum in both culture conditions was at pH 4.0 with production deteriorating beyond pH 6.0, in agreement with findings previously reported in the literature (Prior et al. 1992).
The time course for the production of cellulolytic enzymes by freely suspended and immobilized cells showed that while there were no significant differences in the enzyme titers produced, a lag in the production of the three component enzymes studied occurred in the immobilized cells (Fig. 5). This observation is similar to the findings on studies on T. reesei strain QM 9123 and S. cellulophilum where cells, passively immobilized as in the present studies, showed a lag in enzyme production when compared with free cells (Turker and Mavituna 1987; Tamada et al. 1986). In both studies, the investigators attributed the lag to diffusional constraints; in the former, to slow diffusion of enzyme from the immobilized cell matrix to the bulk liquid, while in the latter, to oxygen diffusion into the immobilized cell matrix. In the present studies, it was observed that, while there was growth within the matrix, mycelia were mostly concentrated on the periphery of the support material. It may well be that it is the diffusional constraints of oxygen and substrate movements, compounded by the presence of a thick outer mycelial layer, that limit the growth of mycelia and restricting it to the outer layers of the support material. This would also explain the observation that enzyme production was dependent on the amount of support material present during cultivation.
The results discussed above showed that the nutritional requirements and culture conditions for enzyme production by free and immobilized A. terreus were not significantly different, except for the increased temperature optimum showed by the immobilized cells (Table 2). However, the major advantage often associated with immobilization is the greater stability conferred and hence leading to increased productivity or activity of cells or enzymes, respectively (Cheetham 1983). In the present study, the stability of the free and immobilized cells with respect to enzyme production was examined under repeated batch culture conditions. Free cells, when cultured under repeated batch conditions, maintained enzyme production for only two cycles of medium changes, after which production deteriorated severely (Fig. 6). In contrast, immobilized cells were active for more than 100 days (through eight medium changes) although enzyme production decreased after 70 days of cultivation. In terms of the reactor output of enzymes, represented in Fig. 6 as the cumulative enzyme activity, under repeated batch conditions, immobilized cells were about 4.5-times more efficient compared to free cells, producing a total of 453 U of enzyme compared to 114 U, respectively. It may thus be inferred that continuous cellulase production may be made possible with cellulolytic organisms immobilized in this manner, which can contribute to decreasing the production cost of the enzyme.
Conclusions
In this study, A. terreus that was isolated from rotting bagasse is capable of cellulase enzyme production when grown either in the free or immobilized states. The immobilized cells gave cellulase activities comparable to those of freely suspend cells. Immobilized cells however showed advantages compared to free cells and it could support a continuos production of cellulase for a much longer period with a 4.5-fold increase in productivity. Furthermore, by using this immobilized method, we could get clear cellulase solution that did not contain the mycelia and growing fungus had a greater buffer to heat shock compared to free cells.
References
Bigelow M, Wyman CE (2002) Cellulase production on bagasse pretreated with hot water. Appl Biochem Biotechnol 98–100:921–934
Brown JA, Collins SA, Wood TM (1987) Development of a medium for high cellulase, xylanase and β-glucosidase production by a mutant strain (NTG III/6) of a cellulolytic fungus Penicillium pinophilum. Enzyme Microbiol Technol 9:355–360
Cao NJ, Xia YK, Gong CS, Tsao GT (1997) Production of 2, 3-butanediol from pretreated corn cob by Klebsiella oxytoca in the presence of fungal cellulase. Appl Biochem Biotechnol 63–65:129–139
Cheetham PSJ (1983) The application of immobilized enzymes, immobilized cells and bioreactors in biotechnology-principles of enzyme engineering. In: Wiseman A (ed) Principles of biotechnology. Surrey University Press, London, pp 172–207
Chibata I, Tosa T, Sato T (1986) Methods of cell immobilization. In: Demain AL, Solomon NA (eds) Manual of industrial microbiology and biotechnology. American Society for Microbiology, Washington, pp 217–229
Coutts AS, Smith RE (1976) Factors influencing the production of cellulases by Sporotrochum thermophile. Appl Environ Microbiol 31:819–825
Desrochers M, Jurasek L, Paige MG (1981) Production of cellulase, β-glucosidase, and xylanase by Schizophyllum commune grown on a cellulose-peptone medium. Dev Ind Microbiol 22:675–684
Jiang Y, Qu Y, Chen S (1998) Screening of alkaline B-glycanases producer and optimization of enzyme production. 7th International Conference on Biotechnology in the Pulp and Paper Industry. Canada, 177–180
Kang SW, Kim SW, Lee SJ (1995) Production of cellulase and xylanase in a bubble column using immobilized Aspergillus niger KKS. Appl Biochem Biotechnol 53:101–106
Lee SM, Koo YM, Lin J (2004) Production of lactic acid from paper sludge by simultaneous saccharification and fermentation. Adv Biochem Eng Biotechnol 87:173–194
Liming X, Xueliang S (2000) High-yield cellulase production by Trichoderma reesei ZU-02 on corn cob residue. Bioresour Technol 91:259–262
Lo CM, Zhang Q, Lee P, Ju LK (2005) Cellulase production by Trichoderma reesei using sawdust hydrolysate. Appl Biochem Biotechnol 121–124:561–573
Mandels M, Andreotti R, Roche C (1976) Measuring of saccharifying cellulase. Biotechnol Bioeng Symp 6:21–33
Miller GL (1959) Use of dinitrosalicylic acid reagent for determination of reducing sugar. Anal Chem 31:426–428
Peitersen N (1977) Continuous cultivation of Trichoderma viride on cellulose. Biotechnol Bioeng 19:337–348
Prior BA, Du Preez JC, Rein PW (1992) Environmental parameters. In: Doelle H, Mitchell D, Roltz C (eds) In solid substrate cultivation. Elsevier Applied Science, London, pp 1–16
Qu Y, Zhu M, Liu K, Bao X, Lin J (2006) Studies on cellulosic ethanol production for sustainable supply of liquid fuel in China. Biotechnol J 1:1235–1240
Ryu DDY, Mandels M (1980) Cellulases: biosynthesis and applications. Enzyme Microbiol Technol 2:91–101
Shen X, Xia L (2006) Lactic acid production from cellulosic material by synergistic hydrolysis and fermentation. Appl Biochem Biotechnol 133:252–262
Sternberg D (1976) Production of cellulase by Trichoderma. Biotechnol Bioeng Symp 6:35–53
Stutzenberger FJ (1971) Cellulase production by Thermomonospora curvata isolated from municipal solid waste compost. Appl Microbiol 22:147–152
Szijarto N, Szengyel Z, Liden G, Reczey K (2004) Dynamics of cellulase production by glucose grown cultures of Trichoderma reesei Rut-C30 as a response to addition of cellulose. Appl Biochem Biotechnol 113–116:115–1124
Tamada M, Kasai N, Kumakura M, Kaetsu I (1986) Periodical batch culture of the immobilized growing fungi Sporotrichum cellulophilum producing cellulase in the nonwoven materials. Biotechnol Bioeng 28:1227–1232
Turker M, Mavituna F (1987) Production of cellulase by freely suspended and immobilized cells of Trichoderma reesei. Enzyme Microbiol Technol 9:739–743
Villena GK, Gutierrez-Correa M (2006) Production of cellulase by Aspergillus niger biofilms developed on polyester cloth. Lett Appl Microbiol 43:262–268
Webb C, Fukuda H, Atkinson B (1986) The production of cellulase in a sprouted bed fermenter using cells immobilized in biomass support particles. Biotechnol Bioeng 28:41–50
Acknowledgments
The authors acknowledge the research grant provided by Universiti Sains Malaysia, Penang that has resulted in this article.
Author information
Authors and Affiliations
Corresponding author
Rights and permissions
About this article
Cite this article
Hui, Y.S., Amirul, A.A., Yahya, A.R.M. et al. Cellulase production by free and immobilized Aspergillus terreus . World J Microbiol Biotechnol 26, 79–84 (2010). https://doi.org/10.1007/s11274-009-0145-9
Received:
Accepted:
Published:
Issue Date:
DOI: https://doi.org/10.1007/s11274-009-0145-9