Abstract
Although resveratrol, an active ingredient derived from grapes and red wine, possesses chemopreventive properties against several cancers, the molecular mechanisms by which it inhibits cell growth and induces apoptosis have not been clearly understood. Here, we examined the molecular mechanisms of resveratrol and its interactive effects with TRAIL on apoptosis in prostate cancer PC-3 and DU-145 cells. Resveratrol inhibited cell viability and colony formation, and induced apoptosis in prostate cancer cells. Resveratrol downregulated the expression of Bcl-2, Bcl-XL and survivin and upregulated the expression of Bax, Bak, PUMA, Noxa, and Bim, and death receptors (TRAIL-R1/DR4 and TRAIL-R2/DR5). Treatment of prostate cancer cells with resveratrol resulted in generation of reactive oxygen species (ROS), translocation of Bax to mitochondria and subsequent drop in mitochondrial membrane potential, release of mitochondrial proteins (cytochrome c, Smac/DIABLO, and AIF) to cytosol, activation of effector caspase-3 and caspase-9, and induction of apoptosis. Resveratrol-induced ROS production, caspase-3 activity and apoptosis were inhibited by N-acetylcysteine. Bax was a major proapoptotic gene mediating the effects of resveratrol as Bax siRNA inhibited resveratrol-induced apoptosis. Resveratrol enhanced the apoptosis-inducing potential of TRAIL, and these effects were inhibited by either dominant negative FADD or caspase-8 siRNA. The combination of resveratrol and TRAIL enhanced the mitochondrial dysfunctions during apoptosis. These properties of resveratrol strongly suggest that it could be used either alone or in combination with TRAIL for the prevention and/or treatment of prostate cancer.
Similar content being viewed by others
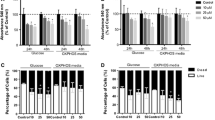
Avoid common mistakes on your manuscript.
Introduction
Prostate cancer is the most common malignancy and the second leading cause of cancer mortality in elderly men [1]. Prostate cancer is unique among human cancers because of its striking age-dependent incidence and variable penetrance. Prostate cancer has a long latency often requiring more than 10 years to develop into a clinically significant disease. The development of prostate cancer in humans has been viewed as a multistage process, involving the onset as small latent carcinoma of low histological grade to large metastatic lesion of high grade [2]. Unfortunately, there are limited treatment options available for this disease and metastatic disease frequently redevelops even after surgery [3, 4]. Therefore, there is an urgent need to discover novel and effective chemopreventive and therapeutic approaches for prostate cancer.
The age-adjusted incidence of prostate cancer has been increasing by approximately 3% annually worldwide [5]. Many risk factors for human prostate cancer have been proposed, including genetic predisposition, age, diet, hormones, and environmental factors. However, its etiology is still largely unknown. The importance of prevention in reducing the morbidity and mortality from cancer has been widely recognized. Future clinical chemoprevention studies should focus on phytochemicals, cancer preventive compounds in fruits, vegetables, and other plants. Many phytochemicals are excellent potential chemopreventive agents, because, in addition to their cancer preventive effects, they are relatively non-toxic and inexpensive, they can be taken orally and some of them have other health benefits as well. There is also a great need to investigate potential benefits and risks of administering phytochemicals before, during or after conventional therapies, such as surgery, chemotherapy, radiation, or hormonal therapy. In addition, administration of chemopreventive agents prior to surgery provides an opportunity to investigate the modulation of genetic and epigenetic pathways by putative cancer preventive compounds and nutrients.
The polyphenolic compound resveratrol is a naturally occurring phytochemical and can be found in many plant species, including grapes, peanuts, and various herbs. Resveratrol has been shown to have anti-inflammatory, antioxidant, antitumor, neuroprotective, and immunomodulatory activities [6–8]. It also has activity in the regulation of multiple cellular events associated with carcinogenesis. Furthermore, it has been examined in several model systems for its potential effect against cancer [9, 10]. Its anticancer effects include its ability to inhibit cell proliferation and angiogenesis, and induce apoptosis in pre-clinical studies [11, 12]. In addition, resveratrol enhances the therapeutic potential of anticancer drugs and sensitizes cancer cells to chemotherapy and radiotherapy [13–16]. These studies with resveratrol provide strong support for the use of resveratrol in human cancer chemoprevention and combination with chemotherapeutic drugs or cytotoxic factors in the treatment of drug refractory tumor cells.
TRAIL, a type II membrane protein belonging to the TNF cytokine family, induces apoptosis in a wide variety of transformed cells [17, 18]. TRAIL binds to several distinct receptors: (a) TRAIL-R1 (DR4) [19]; (b) TRAIL-R2 (DR5) [20]; (c) TRAIL-R3 (DcR1) [21]; and (d) TRAIL-R4 (DcR2) [22]. Both DR4 and DR5 contain the intracellular death domain (DD) essential for the induction of apoptosis following receptor ligation [23]. In contrast, neither DcR1 nor DcR2 mediates apoptosis due to a complete or partial lack of the intracellular DD, respectively [24, 25]. TRAIL receptors are expressed ubiquitously in cancer cells. The binding of TRAIL to DR4 and DR5 leads to the activation of caspase-8 or caspase-10 [26], that in turn activates downstream effector caspases such as caspase-3, and caspase-7 [18]. Activation of caspase-8 or caspase-10 by TRAIL also cleaves BID (a Bcl-2 inhibitory protein) [27]. Truncated BID (tBID) triggers mitochondrial depolarization (decrease in ΔΨm) and causes subsequent release of mitochondrial proteins (cytochrome c, Omi/HtrA2, AIF, and Smac/DIABLO) to cytosol [18, 28]. Bcl-2 and Bcl-XL preserve mitochondrial transmembrane potential and blocks the release of mitochondrial proteins [29, 30]. Once cytochrome c is released into the cytosol, it binds to Apaf-1 (apoptotic protease-activating factor 1) and, in the presence of dATP, recruits and activates procaspase-9 to form the apoptosome [31]. Activated caspases cleave several downstream death substrates and activate endonucleases resulting in apoptosis [31].
The objectives of our study were to examine the molecular mechanisms by which resveratrol enhances the therapeutic potential of TRAIL in androgen-insensitive prostate cancer cells. In the current study, we showed that resveratrol induced apoptosis, and inhibited cell-viability and colony formation, and these effects were further enhanced in the presence of TRAIL. Resveratrol induced apoptosis through regulation of Bcl-2 family members, induction of death receptors TRAIL-R1/DR4 and TRAIL-R2/DR5, translocation of Bax to mitochondria, and release of mitochondrial proteins to cytosol, inhibition of survivin, and activation of caspase(s). In conclusion, our data suggest that resveratrol can be used either alone or in combination with TRAIL for the treatment and/or prevention of prostate cancer.
Materials and methods
Reagents
Antibodies against Bcl-2, Bcl-XL, Bax, Bak, PUMA, Noxa, Bim, Bid, AIF, tubulin, survivin, XIAP, cIAP1, cIAP2, and β-actin were purchased from Santa Cruz Biotechnology Inc. (Santa Cruz, CA). Anti-cytochrome c, and anti-Smac/DIABLO antibodies were purchased from BD Biosciences/Pharmingen (San Diego, CA). N-acetylcysteine (NAC), JC-1, and 5-(and-6)-chloromethyl-2′,7′-dichlorodihydrofluorescein diacetate, acetyl ester (CM-H2DCFDA) were purchased from Invitrogen/Molecular Probes, Inc. (Eugene, OR). Caspase-8 siRNA, Bax siRNA, and control plasmids were purchased from BioVision, Inc. (Mountain View, CA). Terminal Deoxynucleotidyl Transferase Biotin-dUTP Nick End Labeling (TUNEL) assay kit, and caspase-3 and caspase-8 activity kits were purchased from EMD Biosciences/Calbiochem (San Diego, CA). Resveratrol was purchased from LKT Laboratories, Inc. (St. Paul, MN). Enhanced chemiluminescence (ECL) Western blot detection reagents were purchased from Amersham Life Sciences Inc (Arlington Heights, IL). TRAIL was purified as described elsewhere [32].
Cell culture
DU145, and PC3 cells were obtained from the American Type Culture Collection (Manassas, VA) and cultured in RPMI 1640 supplemented with 10% fetal bovine serum (FBS) and 1% antibiotic–antimycotic (Invitrogen) at 37°C in a humidified atmosphere of 95% air and 5% CO2.
XTT assay
Cells (1 × 104 in 200 μl culture medium per well) were seeded in 96-well plate (flat bottom), treated with or without drugs and incubated for various time points at 37°C and 5% CO2. Before the end of the experiment, 50 μl XTT labeling mixture (final concentration, 125 μM XTT (sodium 2,3-bis(2-methoxy-4-nitro-5-sulfophenyl)-2H-tetrazolium-5-carboxanilide inner salt) and 25 μM PMS (phenazine methosulfate) per well was added and plates were incubated for further 4 h at 37°C and 5% CO2. The spectrophotometric absorbance of the sample was measured using a microtitre plate reader. The wavelength to measure absorbance of the formazon product was 450 nm, and the reference wavelength was 650 nm.
Soft agar assay
Cells (2 × 104 cells/well) were seeded in 12-well culture dishes in RPMI/0.35% bacto-agar over a bottom layer of RPMI/0.6% bacto-agar. Cells were then fed with growth media (100-200 μl/well) once a week until colonies grew to a suitable size for observation (about 3 weeks). Number of colonies were counted after they were stained with 3-(4,5-dimethylthiazol-2-yl)-2,5-diphenyltetrazolium bromide (1 mg/ml, 100 μl/well) overnight for better visualization.
Transient transfection
Cells were plated in 60-mm dishes in RPMI 1640 containing 10% FBS and 1% penicillin-streptomycin mixture at a density of 1 × 106 cells/dish. The next day transfection mixtures were prepared. Cells were transfected with expression constructs encoding dominant negative FADD (DN-FADD) or empty vector in the presence of an expression vector pCMV-LacZ (Invitrogen life technologies) expressing β-galactosidase. For each transfection, 2 μg of DNA was diluted into 50 μl of medium without serum. After the addition of 3 μl of LipofectAMINE (Invitrogen life technologies) into 50 μl Opti-MEM medium, the transfection mixture was incubated for 10 min at room temperature. Cells were washed with serum-free medium, the transfection mixture was added, and cultures were incubated for 24 hrs in the incubator. The next day, culture medium was replaced with fresh RPMI 1640 containing 10% FBS and 1% penicillin–streptomycin mixture. Cells were treated with or without resveratrol. At the end of incubation, cells were harvested to measure protein expression by western blot analysis or apoptosis by DAPI staining.
For siRNA experiment, PC-3 cells were transiently transfected with plasmids expressing caspase-8 siRNA (pGB-Caspase-8), Bax siRNA (pGB-Bax siRNA), or control siRNA (pGB-control) in the presence of pCMV-LacZ (Invitrogen life technologies) vector expressing β-galactosidase to control transfection efficiency. Cells were treated as described above.
Measurement of apoptosis
Apoptosis was measured by the terminal deoxynucleotidyl transferase-mediated nick end-labeling method (TUNEL). Stained cells were mounted and analyzed under a fluorescence Olympus microscope (Olympus America Inc, Melville, NY). Pictures were captured using a Photometrics Coolsnap CF color camera (Olympus) and SPOT software (Diagnostic Instruments Inc., Sterling Heights, MI). In some cases, the data were confirmed by staining cells with DAPI as previously described.
Caspase-3 assay
Cells (3 × 104 per well) were seeded in a 96-well plate with 200-μl culture medium. Approximately 16 h later, cells were treated with various doses of resveratrol to induce apoptosis. Casapse-3 activity was measured as per manufacturer’s instructions (EMD Biosciences) with a fluorometer.
Cellular fractionation
Purified mitochondrial preparations were prepared as we described elsewhere [28].
Western blot analysis
Cell pellets were lysed in RIPA buffer containing 1× protease inhibitor cocktail, and protein concentrations were determined using the Bradford assay (Bio-Rad, Philadelphia, PA). Cell lysates were electrophoresed in 12.5% SDS polyacrylamide gels and then transferred onto nitrocellulose membranes. After blotting in 5% nonfat dry milk in TBS, the membranes were incubated with primary antibodies at 1:1,000 dilution in TBS-Tween 20 overnight at 4°C, and then secondary antibodies conjugated with horseradish peroxidase at 1:5,000 dilution in TBS-Tween 20 for 1 h at room temperature. Protein bands were visualized on X-ray film using an enhanced chemiluminescence system.
Reverse transcription reaction, cDNA synthesis, and PCR
The RT-PCR assay was performed as per the manufacturer’s instructions (Access RT-PCR System, Promega, Madison, WI). The primer sequences are as follows: Bak forward, 5′-AGAGCTGTCTGAACTCACGT-3′, reverse, 5′-TTACACTGTGCCAGAGCCAT-3′; Bax forward 5′-AAGAAGCTGAGCGAGTGT-3′, reverse 5′-GGAGGAAGTCCAATGTC-3′; Bim forward, 5′-0C ATGAGAAGATCCTCCCTGCT-3′; reverse 5′-AATGCATTCTCCACACCAGG-3′; Puma forward, 5′-CAGACTGTGAATCCTGTGCT-3′, reverse 5′-ACAGTATCTTACAGGCTGGG-3′; Bcl-2 forward, 5′-GTGGAGGAGCTCTTCAGGGA-3′, reverse 5′-AGGCACCCAGGGTGATGCAA-3′; Bcl-XL forward 5′-GCTGGAGTCAGTTTAGTGATGTGGAA-3′, reverse 5′-GTGGAGCTGGGATGTCAGGTC-3′; and GAPDH forward 5′-TCTGCCCCCTC TGCTGATGC-3′, reverse 5′-CCACCACCCTGTTGCTGTAG-3′. The PCR was performed for 35 cycles consisting of the following steps: denaturation at 94°C for 1 min; annealing at 66°C for 1 min; and extension at 72°C for 2 min. Both gel analysis and a second PCR reaction amplifying the glyceraldehyde-3-phosphate dehydrogenase housekeeping gene were used to assess mRNA integrity. Internal negative control reactions for the RT-PCR were performed using all of the reagents as for the experimental samples, but with lymphoblast RNA in each of the assays. None of the assays exhibited a signal from the internal negative control.
Measurement of mitochondrial membrane potential (ΔΨm)
Mitochondrial energization was determined by retention of JC-1 dye (Molecular Probes Inc., Eugene) as we described earlier [28, 33]. Briefly, drug treated cells (5 × 105) were loaded with JC-1 dye (1 μg/ml) during the last 30 min of incubation at 37°C in a 5% CO2 incubator. Cells were washed in PBS twice. Fluorescence was monitored in a fluorometer using 570-nm excitation/595-nm emission for the J-aggregate of JC1. ΔΨm was calculated as a ratio of the fluorescence of J-aggregate (aqueous phase) and monomer (membrane-bound) forms of JC1.
Determination of reactive oxygen species (ROS)
PC-3 cells were seeded in 96-well plates. After 16 h, cells were loaded with 5 μM CM-H2DCFDA dye for 30 min, and treated with either resveratrol (10 or 20 μM) or 0.05% DMSO for 0–360 min. Fluorescence was measured at excitation wavelength of 488 nm and emission wavelength of 515 nm using a fluorescence plate reader.
Immunocytochemistry
Cells were grown on fibronectin-coated coverslips (Beckton Dickinson, Bedford, MA), washed in PBS, and fixed for 15 min in 4% paraformaldehyde. Fibronectin-coated coverslips allow cells to adhere properly and reduce the chance detachment during staining. Cells were permeabilized in 0.1% Triton X-100, washed and blocked in 10% normal goat serum. Cells were incubated with anti-Bax antibody (1:200) for 18 h at 4°C. Cells were then washed and incubated with fluorescently labeled secondary antibodies (1:200) along with DAPI (1 μg/ml) for 1 h at room temperature. Cells were washed and coverslips were mounted using Vectashield (Vector Laboratories, Burlington, CA). Isotype-specific negative controls were included with each staining. Stained cells were mounted and visualized under a fluorescence Olympus microscope (Olympus America Inc.). Pictures were captured using a Photometrics Coolsnap CF color camera (Olympus) and SPOT software (Diagnostic Instruments Inc.).
Statistical analysis
All data were presented as mean ± SD from at least three sets of independent experiments. ANOVA analysis with Tukey’s multiple comparisons was used to determine the significance of statistical differences between data at the level of P < 0.05 using SPSS computer statistics software (SPSS, Inc., Chicago, IL).
Results
Interactive effects of resveratrol and TRAIL on cell viability and colony formation of prostate cancer cells
We have previously shown that TRAIL induces apoptosis in human prostate cancer cells with varying sensitivity [34], and chemotherapeutic drugs and histone deacetylase inhibitors enhanced the apoptosis-inducing potential of TRAIL [33, 35]. Here, we first measured the effects of resveratrol and/or TRAIL on cell viability in androgen-insensitive prostate cancer PC-3 (p53 null), and DU-145 (mutant p53) cells (Fig. 1). Resveratrol (0–30 μM) inhibited cell viability of PC-3 and DU-145 in a dose-dependent manner (Fig. 1A–B). TRAIL significantly inhibited cell viability in PC-3 cells and was moderately effective in DU-145 cells. Resveratrol enhanced the inhibitory effects of TRAIL on cell viability in PC-3 and DU-145 cells. These data suggest that resveratrol can enhance the biological effect of TRAIL in androgen-insensitive prostate cancer cells.
Interactive effects of resveratrol and TRAIL on cell viability, colony formation, and apoptosis in prostate cancer cells. (A) Interactive effects of resveratrol and TRAIL on cell viability. PC-3 cells were treated with various doses of resveratrol (0–30 μM) in the presence or absence of TRAIL (25 nM) for 48 h. Cell viability was measured by XTT. Data represent mean ± SE. *Significantly different from respective control, P < 0.05. (B) Interactive effects of resveratrol and TRAIL on cell viability in DU-145 cells. Cells were treated with various doses of resveratrol (0–30 μM) in the presence or absence of TRAIL (50 nM) for 48 h. Data represent mean ± SE. *Significantly different from respective control, P < 0.05. (C) Interactive effects of resveratrol and TRAIL on colony formation. PC-3 cells were treated with various doses of resveratrol (0–30 μM) in the presence or absence of TRAIL (25 nM). After 3 weeks, no of colonies were stained and counted. (D) Interactive effects of resveratrol and TRAIL on apoptosis. PC-3 cells were treated with various doses of resveratrol (0–30 μM) in the presence or absence of TRAIL (25 nM) for 48 h. Apoptosis was measured by DAPI staining
Since anchorage independent growth is one of the features of tumor formation, we sought to measure the effects of resveratrol and TRAIL on colony formation in soft agar (Fig. 1C). Resveratrol and TRAIL alone inhibited colony growth of PC-3 cells in a dose-dependent manner. Furthermore, resveratrol enhanced the inhibitory effects of TRAIL on PC-3 cell colony formation. We next measured the interactive effects of resveratrol and TRAIL on apoptosis in PC-3 cells (Fig. 1D). Resveratrol and TRAIL alone induced apoptosis in PC-3 cells. Interestingly, resveratrol enhanced the apoptosis-inducing potential of TRAIL. These data demonstrate that resveratrol inhibits anchorage-dependent and -independent growth of prostate cancer cells, and enhances the therapeutic potential of TRAIL.
We next sought to examine whether pretreatment of PC-3 and DU-145 cells with resveratrol followed by TRAIL induced more apoptosis than the concurrent treatment or TRAIL followed by resveratrol (Fig. 2). The pretreatment of cells with resveratrol may increase the apoptotic effects of TRAIL by up-regulating death receptors. PC-3 and DU-145 cells were pre-treated with resveratrol for 24 h, followed by TRAIL for another 24 h, and vice versa. Interestingly, the pretreatment of cells with resveratrol followed by TRAIL induced significantly more apoptosis than concurrent treatment or single agent alone. In order to understand the mechanism behind this interaction, reverse sequence of drug exposure was used in which cells were pre-treated with TRAIL for 24 h followed by treatment with resveratrol for additional 24 h. Reverse sequence of treatment, TRAIL followed by resveratrol, has resulted significantly less apoptosis than the sequential treatment of cells with resveratrol followed by TRAIL. However, there was no difference between concurrent treatment and TRAIL followed by resveratrol. These data suggest that sequential treatment with resveratrol followed by TRAIL can be used to enhance the apoptosis-inducing potential of TRAIL in prostate cancer cells.
Effects of different treatment combinations of resveratrol and TRAIL on apoptosis. (A) PC-3 cells were treated with resveratrol (15 μM) in the presence or absence of TRAIL (25 nM). TRAIL was added simultaneously with resveratrol (cotreatment), before or after 24 h of resveratrol treatment. Apoptosis was measured by DAPI staining. Data represent mean ± SE. *Significantly different from respective control, P < 0.05. (B) DU-145 cells were treated with resveratrol (15 μM) in the presence or absence of TRAIL (50 nM). TRAIL was added simultaneously with resveratrol (cotreatment), before or after 24 h of resveratrol treatment. Apoptosis was measured by DAPI staining. Data represent mean ± SE. *Significantly different from respective control, P < 0.05
Upregulation of TRAIL-R1/DR4 and TRAIL-R2/DR5 by resveratrol
TRAIL binds to TRAIL-R1/DR4 and TRAIL-R2/DR5 [26]. Since resveratrol enhances the apoptosis-inducing potential of TRAIL, we sought to examine the effects of resveratrol on cell-surface expression of death receptors by flow cytometry. Resveratrol induced expression of death receptors TRAIL-R1/DR4 and TRAIL-R2/DR5 in PC-3 cells (Fig. 3). By comparison, resveratrol had no effects on the expression of decoy receptors TRAIL-R3/DcR1 and TRAIL-R4/DcR2. These data suggest that upregulation of death receptors may be one of the mechanisms by which resveratrol enhances the apoptosis-inducing potential of TRAIL.
The involvement of caspases and FADD in synergistic interactions between resveratrol and TRAIL
Caspases mediate both apoptosis and inflammation through aspartate-specific cleavage of a wide number of cellular substrates [36]. We therefore examined the activation of caspase-3 and caspase-8 by fluorogenic assay in PC-3 cells (Fig. 4A–B). Resveratrol induced caspase-3 activity in PC-3 cells in a dose-dependent manner. Resveratrol slightly activated caspase-8. By comparison, TRAIL induced caspase-8 activity in PC-3 cells. Interestingly, the combination of resveratrol and TRAIL further enhanced caspase-8 activity.
Interactive effects of resveratrol and TRAIL on caspase activity and PARP cleavage. (A) Interactive effects of resveratrol and TRAIL on caspase-3 activity. PC-3 cells were treated with resveratrol (0–30 μM) in the presence or absence of TRAIL (25 nM) for 24 h. At the end of incubation period, caspase-3 activity was measured by flurometric assay. (B) Interactive effects of resveratrol and TRAIL on caspase-8 activity. PC-3 cells were treated with resveratrol (0–30 μM) in the presence or absence of TRAIL (25 nM) for 24 h. At the end of incubation period, caspase-8 activity was measured by flurometric assay. (C) Interactive effects of resveratrol and TRAIL on cleavage of caspase-8, caspase-3, caspase-9, and PARP. PC-3 cells were treated with resveratrol (0, 10, or 20 μM) in the presence or absence of TRAIL (25 nM) for 24 h. At the end of incubation period, cells were harvested. Crude proteins were run on SDS-PAGE, and immunoblotted with anti-caspase-8, anti-caspase-3, anti-caspase-9, and anti-PARP antibodies. β-actin was used as a loading control. (D) Effects of dominant negative FADD or caspase-8 siRNA on resveratrol and/or TRAIL-induced apoptosis. PC-3 cells were transiently transfected with either control plasmid or plasmid expressing dominant negative FADD (DN-FADD) or caspase-8 siRNA along with plasmid (pCMV-LacZ) encoding the β-galactosidase (β-Gal) enzyme. There was no difference in transfection efficiency among groups. Cell lysates were run on SDS-PAGE to measure the expression of FADD and caspase-8 by Western blot analysis. Transfected cells were treated with resveratrol (0, 10, or 20 μM) in the presence or absence of TRAIL (25 nM) for 48 h. Apoptosis was measured by DAPI staining. Data represent mean ± SE. *Significantly different from respective control; # and $ = treatment groups were significantly different, P < 0.05
Poly-ADP Ribose Polymerase (PARP), a major substrate, is cleaved by caspase(s) during apoptosis [33, 37–39]. The cleavage of procaspases is an indication of their activation. We therefore examined the cleavage of caspase-8, caspase-3, caspase-9, and PARP in PC-3 cells by the Western blot analysis (Fig. 4C). Casapse-8 and caspase-9 antibodies recognize the whole molecule (procaspases), whereas casaspe-3 and PARP antibodies recognize their cleavage products. Treatment of PC-3 cells with resveratrol resulted in cleavage of caspase-3, caspase-9, and PARP. By comparison, TRAIL caused cleavage of caspase-8, caspae-3, caspase-9, and PARP. The combination of resveratrol and TRAIL further enhanced the cleavage of caspase-8, caspase-3, caspase-9, and PARP. The data suggest that resveratrol activates effector caspases, whereas TRAIL activates initiator as well as effector caspases to induce apoptosis.
Since resveratrol enhanced the apoptosis-inducing potential of TRAIL, we next sought to examine the involvement of death receptor pathway in this synergistic interaction. Here, we have taken two approaches. In the first approach we have used dominant negative FADD (DN-FADD), whereas in second approach we have used caspase-8 siRNA to inhibit death receptor pathway. These proteins are essential for the formation of active TRAIL-DISC. PC-3 cells were transfected with plasmid expressing DN-FADD, caspase-8 siRNA or respective controls (Fig. 4D). Data revealed that DN-FADD and caspase-8 siRNA inhibited the expression of FADD and caspase-8, respectively. Resveratrol and TRAIL alone induced apoptosis, and the combination of these agents induced apoptosis in a synergistic manner. Overexpression of DN-FADD inhibited TRAIL-induced apoptosis and abolished the synergistic interaction between resveratrol and TRAIL (Fig. 4D). Similarly, caspase-8 siRNA inhibited the synergistic interaction between resveratrol and TRAIL (Fig. 4D). These data suggest that death receptor pathway is involved in synergistic interaction between resveratrol and TRAIL.
Effects of resveratrol on mitochondrial membrane potential, release of mitochondrial proteins, and expression of IAPs
The intrinsic pathway is engazed by the trascriptional or post-traslational regulation of Bcl-2 proteins that directly impact on mitochondrial outer-membrane permeabilization (MOMP) [40]. Upon induction of MOMP, mitochondrial proteins such as cytochrome c, Smac/DIABLO, and AIF are released to cytosol [41]. Cytochrome c causes APAF-1 oligomerization resulting in apoptosome formation. This complex, in turn, recruits and activates procaspase-9, which then activates executioner caspase-3 and caspase-7. We therefore measure the mitochondrial membrane potential, and release of cytochrome c, AIF and Smac/DIABLO from mitochondria to cytosol. Treatment of prostate cancer PC-3 cells with resveratrol resulted in dropping of mitochondrial membrane potential (Δψm) in a time-dependent manner (Fig. 5A).
Effects of resveratrol on mitochondrial membrane potential and release of mitochondrial proteins to cytosol. (A) Resveratrol induces drop in mitochondrial membrane potential (Δψm). PC-3 cells were treated with resveratrol (20 μM) for 0–24 h. Cells were stained with JC1 dye, and Δψm was measured by a fluorometer as per manufacturer’s instructions. (B) Interactive effects of resveratrol and TRAIL on mitochondrial membrane potential (Δψm). PC-3 cells were treated with resveratrol (20 μM) in the presence or absence of TRAIL (25 nM) for 0, 8, and 16 h. Cells were stained with JC1 dye, and Δψm was measured by a fluorometer as per manufacturer’s instructions. (C) PC-3 cells were treated with resveratrol (20 μM) for 0, 6, 12, or 24 h, and cytoplasmic fractions were prepared. Crude proteins were subjected to SDS-PAGE and immunoblotted with anti-cytochrome c (Cyto C), anti-AIF, or anti-Smac/DIABLO antibody. (D) Effects of resveratrol on the expressions of IAPs in PC-3 cells. Cells were treated with resveratrol (0–20 μM) for 24 or 48 h. Crude proteins were subjected to SDS-PAGE and immunoblotted with antibody specific for XIAP, survivin, cIAP1, or cIAP2
We next examined whether the combination of resveratrol and TRAIL had additive effects on mitochondrial events such as drop in mitochondrial membrane potential (Fig. 5B). Similar to resveratrol, treatment of PC-3 cells with TRAIL caused a drop in Δψm at 8 and 16 h. The combination of resveratrol and TRAIL was more effective in dropping Δψm than single agent alone.
We next examined the effects of resveratrol on the release of cytochrome c, AIF and Smac/DIABLO from mitochondria to cytosol (Fig. 5C). PC-3 cells were treated with resveratrol and cytoplasmic fractions were run on SDS-PAGE. Treatment of cells with resveratrol caused the release of cytochrome c, AIF, and Smac/DIABLO from mitochondria to cytosol. These data strongly suggest that resveratrol induces apoptosis through mitochondrial pathway.
Mammalian cells express mainly four inhibitors of apoptosis proteins (IAPs) which bind to caspases and inhibit their activity [42, 43]. We therefore sought to measure the expression of human IAPs (XIAP, survivin, cIAP1, and cIAP2) (Fig. 5D). Resveratrol inhibited the expression of survivin and had no effect on XIAP, cIAP1, and cIAP2 expression. These data suggest that survivin may be a critical regulator of cells death as it is highly expressed in prostate cancer cells.
Resveratrol regulates Bcl-2 family members and translocates Bax to mitochondria
The Bcl-2 family of proteins plays a key role in regulating apoptosis mainly at the level of mitochondria, and thus controlling the release of mitochondrial proteins, and caspase activation [28, 44]. We therefore sought to examine the effects of resveratrol on Bcl-2 family members by the Western blot analysis and RT-PCR (Fig. 6A and B). Resveratrol induced the expression of proapoptotic proteins Noxa, Bim, Bak, and Bax, and inhibited antiapoptotic proteins Bcl-2 and Bcl-XL (Fig. 6A). Resveratrol also induced Bid cleavage. Similarly, resveratrol inhibited gene expression of Bcl-2 and Bcl-XL and induced Bax, Bak, Bim, and PUMA, as measured by RT-PCR (Fig. 6B). These data suggest that resveratrol regulates both pro- and anti-apoptotic members of Bcl-2 family, and may link death receptor pathway through cleavage and activation of Bid.
Effects of resveratrol on the expressions of Bcl-2 family members, and translocation of Bax to mitochondria in PC-3 cells. (A) Effects of resveratrol on protein expression of Bcl-2 family members. Cells were treated with resveratrol (0–20 μM) for 24 or 48 h. The expressions of Noxa, Bim, Bak, Bax, Bid, Bcl-2, and Bcl-XL were examined by Western blot analysis. (B) Regulation of gene expression of Bcl-2 family members by resveratrol. Cells were treated with or without resveratrol (10 or 20 μM) for 6, 12, or 24 h. The expressions of Bak, Bax, Bim, PUMA, Bcl-2, and Bcl-XL were examined by RT-PCR. GAPDH was used as a loading control. (C) Resveratrol induces translocation of Bax to the mitochondria. PC-3 cells were treated with or without resveratrol (20 μM) for 2 or 4 h. Cells were fixed, permeabilized, and stained with anti-Bax antibody at 4°C for 18 h. After washing, cells were stained with mitotracker red (mitochondrial staining), DAPI (nuclear staining) and secondary antibody conjugated with FITC (for Bax). Red color = mitochondria, green color = Bax, blue color = nucleus, yellow = translocation of Bax or p53 to mitochondria. (D) Effects of Bax inhibition by siRNA on resveratrol and/or TRAIL-induced apoptosis. PC-3 cells were transiently transfected with either control plasmid or plasmid expressing Bax siRNA along with plasmid (pCMV-LacZ) encoding the β-galactosidase (β-Gal) enzyme. There was no difference in transfection efficiency between groups. Cell lysates were run on SDS-PAGE to measure the expression of Bax by Western blot analysis. Transfected cells were treated with or without resveratrol (10 or 20 μM) in the presence or absence of TRAIL (25 nM) for 48 h
We and others have previously shown that Bax translocation from cytosol to mitochondria is essential for Bax and Bak oligomerization, and the release of mitochondrial proteins such as cytochrome c and Smac/DIABLO [28, 45]. We therefore examined whether resveratrol induces translocation of Bax to mitochondria by immunohistochemistry (Fig. 6C). In control cells, Bax was not co-localized to mitochondria, as it was clear from the appearance of distinct red (mitochondria) and green (Bax) color. Treatment of PC-3 cells with resveratrol resulted in translocation of Bax to mitochondria (appearance of yellow mitochondria), suggesting the translocation and activation of Bax may disrupt mitochondrial homeostasis.
We next examined the effects of inhibiting Bax by siRNA on resveratrol-induced apoptosis. Transfection of PC-3 cells with plasmid expressing Bax siRNA significantly inhibited Bax expression (Fig. 6D). Resveratrol and TRAIL alone induced apoptosis in PC-3 cells. Bax siRNA significantly inhibited resveratrol and/or TRAIL-induced apoptosis. These data suggest that Bax is required for apoptosis induced by resveratrol and TRAIL alone or by their combination.
Generation of reactive oxygen species (ROS) by resveratrol
Generation of ROS by oxidative damage play an important role in apoptosis [46, 47]. We and others have shown that chemopreventive agents induce apoptosis in cancer cells through generation of ROS [48–50]. We therefore examined whether generation of ROS was responsible for apoptosis induction by resveratrol. Treatment of PC-3 cells with resveratrol resulted in ROS production which increased over time, reached a maximum level at 120 min, and declined there after (Fig. 7A). Pretreatment of PC-3 cells with N-acetylcysteine (NAC) inhibited resveratrol-induced ROS production. NAC is precursor of glutathione (GSH), an antioxidant. Since resveratrol induced caspase-3 activity and apoptosis, we next examined whether generation of ROS was responsible for caspase-3 activation and apoptosis. Resveratrol induced caspase-3 activity in a dose-dependent manner (Fig. 7B). Pretreatment of PC-3 cells with NAC followed by resveratrol treatment caused a significant inhibition of resveratrol-induced apoptosis (Fig. 7C), which was correlated with a marked reduction in resveratrol-induced caspase-3 activity (Fig. 7B). Together these results suggest that resveratrol-induced apoptosis is also mediated through generation of ROS which, in turn, may activate cell-intrinsic pathway.
Involvement of reactive oxygen species in resveratrol induced caspase-3 activity and apoptosis. (A) Generation of ROS by resveratrol. PC-3 cells were seeded in 96-well plates, loaded with 5 μM CM-H2DCFDA dye for 30 min, and treated with either resveratrol (20 μM) or N-acetylcysteine (NAC) (50 mM) plus resveratrol (20 μM) for 0–360 min. Fluorescence was measured by a fluorometer as per manufacturer’s instructions (EMD Biosciences/Molecular Probes). *Significantly different from respective controls, P < 0.05. (B) Inhibition of resveratrol-induced caspase-3 activity by NAC. PC-3 cells were pre-treated with 50 mM NAC for 2 h followed by treatment with resveratrol (10 or 20 μM) for 12 h, and caspase-3 activity was measured by a fluorometer as per manufacturer’s instructions. *Significantly different from respective control; # or $ = pointed groups were significantly different, P < 0.05. (C) Inhibition of resveratrol-induced apoptosis by NAC. PC-3 cells were pre-treated with 50 mM NAC for 2 h followed by treatment with 10 or 20 μM resveratrol for 48 h, and apoptosis was measured by TUNEL assay. a and c were significantly different from b and d, respectively, P < 0.05
Discussion
In the present study, we have demonstrated that resveratrol inhibits growth and induces apoptosis in androgen-insensitive prostate cancer cells through activation of multiple signaling pathways. Resveratrol induces apoptosis mainly through mitochondrial-dependent pathway which was evident by drop in mitochondrial membrane potential, generation of reactive oxygen species (ROS), and release of mitochondrial proteins (cytochrome c, Smac/DIABLO, and AIF) in prostate cancer cells. Resveratrol induces expression of proapoptotic proteins (Bax, Bak, PUMA, Noxa, and Bim) and inhibits expression of antiapoptotic proteins (Bcl-2 and, Bcl-XL). These proteins exert their effect mainly at the level of mitochondria and regulate the release of mitochondrial proteins. Furthermore, resveratrol enhances translocation of Bax to mitochondria where it may interact with other Bcl-2 family members to cause permeabilization of outer mitochondrial membrane (OMM) and release of mitochondrial proteins leading to caspase activation and apoptosis. Finally, resveratrol enhances the apoptosis-inducing potential of TRAIL by up-regulating death receptors (DR4 and DR5) and cleaving Bid.
In order for a mitochondria to undergo mitochondrial outer membrane permeabilization (MOMP), a coordinated effort between numerous Bcl-2 proteins must be engaged to allow for permeabilization of the OMM [46]. This permeabilization is likely achieved by the formation of membrane-spanning pores through which the intermembrane space proteins are released. Once MOMP has occurred, the cytosolic machinery responds by activating caspases. This process may utilize ROS. Our data have shown that resveratrol causes drop in mitochondrial membrane potential and release of Smac/DIABLO, cytochrome c and AIF from mitochondria to cytosol. We have recently shown that Bax and Bak differentially regulate the release of cytochrome c and Smac from mitochondria to cytosol upon treatment with TRAIL [28]. The effects of resveratrol on mitochondrial events were further enhanced in the presence of TRAIL. Smac is released from mitochondria to the cytosol after cellular insults and binds to IAPs (XIAP, survivin, c-IAP-1, or c-IAP-2), and abrogates IAP-mediated inhibition of caspase-3 and caspase-7, thereby facilitating caspase-mediated apoptosis. Our data indicate that the major IAP regulated by resveratrol is survivin. The release of mitochondrial apoptogenic factors is regulated by the pro- and anti-apoptotic Bcl-2 family proteins, which either induce or prevent the permeabilization of the OMM. Upon disruption of mitochondrial function, energy production wanes and the cell is left to die.
Recently, the Bcl-2 gene family has emerged as critical regulators of apoptosis in a variety of physiological and pathological contexts [44, 51]. Study of the mechanism of apoptosis by Bcl-2-related genes offers new possibilities for prevention and treatment of several human diseases. Members of the Bcl-2 protein family are distinguished by the presence of up to four different Bcl-2 homology domains (designated BH1-4) [51, 52]. In general, those that contain all four domains (Bcl-2, Mcl-1 and Bcl-XL) are antiapoptotic, whereas those that contain less are proapoptotic. The proapoptotic group can be further divided into two groups, multidomains or BH123 members such as Bax and Bak, and BH3-only proteins, including Bid, Bim, and PUMA. The multidomain proapoptotic proteins Bax and Bak are constitutively expressed and only induce MOMP following apoptotic stimuli, suggesting that they are inactive in non-apoptotic cells [52, 53]. Bcl-2 inhibits Bax translocation from cytosol to mitochondria during drug-induced apoptosis of human tumor cells [54]. Indeed, the ratio between these two subsets determines, in part, the susceptibility of cells to a death signal [55]. Furthermore, these death-inducing proteins heterodimerize with members of the death-inhibitory family [56]. Overexpression of Bcl-2 and Bcl-XL protein protects a wide variety of cell types from many death-inducing stimuli [29, 30, 57, 58]. In the present study, Bax appear to play a major role as Bax siRNA inhibited resveratrol-induced apoptosis. Further studies are needed to examine the role of other proapoptotic proteins such as PUMA in resveratrol-induced apoptosis.
The integrity of mitochondrial function is fundamental to cell life. The cell demands for mitochondria and their complex integration into cell biology, extends far beyond the provision of ATP. We and others have shown that disturbances of mitochondrial function lead to disruption of cell function, expressed as disease or even death. Mitochondria are major producers of free radical species and also possibly of nitric oxide, and are, at the same time, major targets for oxidative damage [59, 60]. Reactive oxygen species induce a vide range of responses depending on the cell type and the levels of ROS within the cell. High levels of ROS can lead to necrotic cell death, whereas low levels of ROS have been shown to induce apoptotic cell death [61, 62]. The induction of ROS by resveratrol in cancer cells is one of the mechanisms by which it preferentially kills cancer cells.
Resveratrol is found in high concentrations (2–40 μM) in red wine [63]. Our data on IC50 values (5–10 μM) for inhibition of cell proliferation by resveratrol are in agreement with others [64–66]. The bioavailability of free resveratrol in humans is reported to be extremely low. A recent study has shown the plasma concentrations of free resveratrol of approximately 21 nM after an oral dose of 25 mg [67]. Resveratrol can be metabolized through hydroxylation, glucuronidation, sulfation, and hydrogenation. Although the bioavailability of resveratrol itself is low in humans, peak plasma levels of total resveratrol of up to 2 μM have been reported in humans [67].
In summary, we have demonstrated that resveratrol inhibits growth and induces apoptosis of androgen-insensitive prostate cancer cells through multiple mechanisms. It regulates Bcl-2 family members, causes translocation of Bax to mitochondria, releases mitochondrial proteins (Smac/DIOABLO, cytochrome c, and AIF), generates ROS, inhibits surviving, and activates caspase(s). Furthermore, resveratrol upregulates TRAIL-R1/DR4 and TRAIL-R2/DR5 which, in turn, enhances the apoptosis-inducing potential of TRAIL. Thus, resveratrol alone or in combination with TRAIL can be used to prevent and/or treat human prostate cancer.
References
Landis SH, Murray T, Bolden S, Wingo PA (1999) Cancer statistics, 1999. CA Cancer J Clin 49:8–31, 1
Long RJ, Roberts KP, Wilson MJ, Ercole CJ, Pryor JL (1997) Prostate cancer: a clinical and basic science review. J Androl 18:15–20
Petrylak DP (1999) Chemotherapy for advanced hormone refractory prostate cancer. Urology 54:30–35
Pisters LL (1999) The challenge of locally advanced prostate cancer. Semin Oncol 26:202–216
Boyle P, Maisonneuve P, Napalkov P (1995) Geographical and temporal patterns of incidence and mortality from prostate cancer. Urology 46:47–55
Jang M, Cai L, Udeani GO, Slowing KV, Thomas CF, Beecher CW, Fong HH, Farnsworth NR, Kinghorn AD, Mehta RG, Moon RC, Pezzuto JM (1997) Cancer chemopreventive activity of resveratrol, a natural product derived from grapes. Science 275:218–220
Cos P, De Bruyne T, Apers S, Vanden Berghe D, Pieters L, Vlietinck AJ (2003) Phytoestrogens: recent developments. Planta Med 69:589–599
Inoue H, Jiang XF, Katayama T, Osada S, Umesono K, Namura S (2003) Brain protection by resveratrol and fenofibrate against stroke requires peroxisome proliferator-activated receptor alpha in mice. Neurosci Lett 352:203–206
Agarwal R (2000) Cell signaling and regulators of cell cycle as molecular targets for prostate cancer prevention by dietary agents. Biochem Pharmacol 60:1051–1059
Hsieh TC, Wu JM (2000) Grape-derived chemopreventive agent resveratrol decreases prostate-specific antigen (PSA) expression in LNCaP cells by an androgen receptor (AR)-independent mechanism. Anticancer Res 20:225–228
Bhat KP, Pezzuto JM (2002) Cancer chemopreventive activity of resveratrol. Ann NY Acad Sci 957:210–229
Garvin S, Ollinger K, Dabrosin C (2006) Resveratrol induces apoptosis and inhibits angiogenesis in human breast cancer xenografts in vivo. Cancer Lett 231:113–122
Delmas D, Rebe C, Micheau O, Athias A, Gambert P, Grazide S, Laurent G, Latruffe N, Solary E (2004) Redistribution of CD95, DR4 and DR5 in rafts accounts for the synergistic toxicity of resveratrol and death receptor ligands in colon carcinoma cells. Oncogene 23:8979–8986
Fulda S, Debatin KM (2004) Sensitization for anticancer drug-induced apoptosis by the chemopreventive agent resveratrol. Oncogene 23:6702–6711
Fulda S, Debatin KM (2005) Resveratrol-mediated sensitisation to TRAIL-induced apoptosis depends on death receptor and mitochondrial signalling. Eur J Cancer 41:786–798
Garg AK, Buchholz TA, Aggarwal BB (2005) Chemosensitization and radiosensitization of tumors by plant polyphenols. Antioxid Redox Signal 7:1630–1647
Srivastava RK (2000) Intracellular mechanisms of TRAIL and its role in cancer therapy. Mol Cell Biol Res Commun 4:67–75
Suliman A, Lam A, Datta R, Srivastava RK (2001) Intracellular mechanisms of TRAIL: apoptosis through mitochondrial-dependent and -independent pathways. Oncogene 20:2122–2133
Pan G, O’Rourke K, Chinnaiyan AM, Gentz R, Ebner R, Ni J, Dixit VM (1997) The receptor for the cytotoxic ligand TRAIL. Science 276:111–113
Walczak H, Degli-Esposti MA, Johnson RS, Smolak PJ, Waugh JY, Boiani N, Timour MS, Gerhart MJ, Schooley KA, Smith CA, Goodwin RG, Rauch CT (1997) TRAIL-R2: a novel apoptosis-mediating receptor for TRAIL. Embo J 16:5386–5397
Degli-Esposti MA, Smolak PJ, Walczak H, Waugh J, Huang CP, DuBose RF, Goodwin RG, Smith CA (1997) Cloning and characterization of TRAIL-R3, a novel member of the emerging TRAIL receptor family. J Exp Med 186:1165–1170
Degli-Esposti MA, Dougall WC, Smolak PJ, Waugh JY, Smith CA, Goodwin RG (1997) The novel receptor TRAIL-R4 induces NF-kappaB and protects against TRAIL-mediated apoptosis, yet retains an incomplete death domain. Immunity 7:813–820
Srivastava RK (2001) TRAIL/Apo-2L: mechanisms and clinical applications in cancer. Neoplasia 3:535–546
Sheridan JP, Marsters SA, Pitti RM, Gurney A, Skubatch M, Baldwin D, Ramakrishnan L, Gray CL, Baker K, Wood WI, Goddard AD, Godowski P, Ashkenazi A (1997) Control of TRAIL-induced apoptosis by a family of signaling and decoy receptors. Science 277:818–821
Marsters SA, Sheridan JP, Pitti RM, Huang A, Skubatch M, Baldwin D, Yuan J, Gurney A, Goddard AD, Godowski P, Ashkenazi A (1997) A novel receptor for Apo2L/TRAIL contains a truncated death domain. Curr Biol 7:1003–1006
Shankar S, Srivastava RK (2004) Enhancement of therapeutic potential of TRAIL by cancer chemotherapy and irradiation: mechanisms and clinical implications. Drug Resist Updat 7:139–156
Wang K, Yin XM, Chao DT, Milliman CL, Korsmeyer SJ (1996) BID: a novel BH3 domain-only death agonist. Genes Dev 10:2859–2869
Kandasamy K, Srinivasula SM, Alnemri ES, Thompson CB, Korsmeyer SJ, Bryant JL, Srivastava RK (2003) Involvement of proapoptotic molecules Bax and Bak in tumor necrosis factor-related apoptosis-inducing ligand (TRAIL)-induced mitochondrial disruption and apoptosis: differential regulation of cytochrome c and Smac/DIABLO release. Cancer Res 63:1712–1721
Srivastava RK, Sasaki CY, Hardwick JM, Longo DL (1999) Bcl-2-mediated drug resistance: inhibition of apoptosis by blocking nuclear factor of activated T lymphocytes (NFAT)-induced Fas ligand transcription. J Exp Med 190:253–265
Srivastava RK, Sollott SJ, Khan L, Hansford R, Lakatta EG, Longo DL (1999) Bcl-2 and Bcl-X(L) block thapsigargin-induced nitric oxide generation, c-Jun NH(2)-terminal kinase activity, and apoptosis. Mol Cell Biol 19:5659–5674
Green DR (2005) Apoptotic pathways: ten minutes to dead. Cell 121:671–674
Kim EJ, Suliman A, Lam A, Srivastava RK (2001) Failure of Bcl-2 to block mitochondrial dysfunction during TRAIL-induced apoptosis. Tumor necrosis-related apoptosis-inducing ligand. Int J Oncol 18:187–194
Shankar S, Chen X, Srivastava RK (2005) Effects of sequential treatments with chemotherapeutic drugs followed by TRAIL on prostate cancer in vitro and in vivo. Prostate 62:165–186
Chen X, Thakkar H, Tyan F, Gim S, Robinson H, Lee C, Pandey SK, Nwokorie C, Onwudiwe N, Srivastava RK (2001) Constitutively active Akt is an important regulator of TRAIL sensitivity in prostate cancer. Oncogene 20:6073–6083
Singh TR, Shankar S, Srivastava RK (2005) HDAC inhibitors enhance the apoptosis-inducing potential of TRAIL in breast carcinoma. Oncogene 24:4609–4623
Green DR, Reed JC (1998) Mitochondria and apoptosis. Science 281:1309–1312
Shankar S, Singh TR, Chen X, Thakkar H, Firnin J, Srivastava RK (2004) The sequential treatment with ionizing radiation followed by TRAIL/Apo-2L reduces tumor growth and induces apoptosis of breast tumor xenografts in nude mice. Int J Oncol 24:1133–1140
Shankar S, Singh TR, Fandy TE, Luetrakul T, Ross DD, Srivastava RK (2005) Interactive effects of histone deacetylase inhibitors and TRAIL on apoptosis in human leukemia cells: involvement of both death receptor and mitochondrial pathways. Int J Mol Med 16:1125–1138
Shankar S, Singh TR, Srivastava RK (2004) Ionizing radiation enhances the therapeutic potential of TRAIL in prostate cancer in vitro and in vivo: Intracellular mechanisms. Prostate 61:35–49
Spierings D, McStay G, Saleh M, Bender C, Chipuk J, Maurer U, Green DR (2005) Connected to death: the (unexpurgated) mitochondrial pathway of apoptosis. Science 310:66–67
Green DR, Kroemer G (2004) The pathophysiology of mitochondrial cell death. Science 305:626–629
Deveraux QL, Reed JC (1999) IAP family proteins–suppressors of apoptosis. Genes Dev 13:239–252
Eiben LJ, Duckett CS (1998) The IAP family of apoptotic regulators. Results Probl Cell Differ 24:91–104
Cory S, Adams JM (2002) The Bcl2 family: regulators of the cellular life-or-death switch. Nat Rev Cancer 2:647–656
Degenhardt K, Chen G, Lindsten T, White E (2002) BAX and BAK mediate p53-independent suppression of tumorigenesis. Cancer Cell 2:193–203
Kroemer G, Reed JC (2000) Mitochondrial control of cell death. Nat Med 6:513–519
Jacobson MD (1996) Reactive oxygen species and programmed cell death. Trends Biochem Sci 21:83–86
Shankar S, Srivastava RK (2007) Involvement of Bcl-2 family members, phosphatidylinositol 3′-kinase/AKT and mitochondrial p53 in curcumin (diferulolylmethane)-induced apoptosis in Prostate Cancer. Inter J Oncol 30(4): 905–918
Azam S, Hadi N, Khan NU, Hadi SM (2004) Prooxidant property of green tea polyphenols epicatechin and epigallocatechin-3-gallate: implications for anticancer properties. Toxicol In Vitro 18:555–561
Fujisawa S, Kadoma Y (2006) Anti- and pro-oxidant effects of oxidized quercetin, curcumin or curcumin-related compounds with thiols or ascorbate as measured by the induction period method. In Vivo 20:39–44
Korsmeyer SJ (1999) BCL-2 gene family and the regulation of programmed cell death. Cancer Res 59:1693s–1700s
Kuwana T, Newmeyer DD (2003) Bcl-2-family proteins and the role of mitochondria in apoptosis. Curr Opin Cell Biol 15:691–699
Letai A, Bassik MC, Walensky LD, Sorcinelli MD, Weiler S, Korsmeyer SJ (2002) Distinct BH3 domains either sensitize or activate mitochondrial apoptosis, serving as prototype cancer therapeutics. Cancer Cell 2:183–192
Murphy E, Imahashi K, Steenbergen C (2005) Bcl-2 regulation of mitochondrial energetics. Trends Cardiovasc Med 15:283–290
Oltvai ZN, Milliman CL, Korsmeyer SJ (1993) Bcl-2 heterodimerizes in vivo with a conserved homolog, Bax, that accelerates programmed cell death. Cell 74:609–619
Korsmeyer SJ (1995) Regulators of cell death. Trends Genet 11:101–105
Haldar S, Basu A, Croce CM (1997) Bcl2 is the guardian of microtubule integrity. Cancer Res 57:229–233
Levine B, Goldman JE, Jiang HH, Griffin DE, Hardwick JM (1996) Bc1-2 protects mice against fatal alphavirus encephalitis. Proc Natl Acad Sci USA 93:4810–4815
Duchen MR (2004) Mitochondria in health and disease: perspectives on a new mitochondrial biology. Mol Aspects Med 25:365–451
Finsterer J (2004) Mitochondriopathies. Eur J Neurol 11:163–186
Kroemer G, Zamzami N, Susin SA (1997) Mitochondrial control of apoptosis. Immunol Today 18:44–51
Kannan K, Jain SK (2000) Oxidative stress and apoptosis. Pathophysiology 7:153–163
Kopp P (1998) Resveratrol, a phytoestrogen found in red wine. A possible explanation for the conundrum of the ‘French paradox’? Eur J Endocrinol 138:619–620
Bhat KP, Lantvit D, Christov K, Mehta RG, Moon RC, Pezzuto JM (2001) Estrogenic and antiestrogenic properties of resveratrol in mammary tumor models. Cancer Res 61:7456–7463
Jang DS, Kang BS, Ryu SY, Chang IM, Min KR, Kim Y (1999) Inhibitory effects of resveratrol analogs on unopsonized zymosan-induced oxygen radical production. Biochem Pharmacol 57:705–712
Gescher AJ, Steward WP (2003) Relationship between mechanisms, bioavailibility, and preclinical chemopreventive efficacy of resveratrol: a conundrum. Cancer Epidemiol Biomarkers Prev 12:953–957
Walle T, Hsieh F, DeLegge MH, Oatis JE Jr, Walle UK (2004) High absorption but very low bioavailability of oral resveratrol in humans. Drug Metab Dispos 32:1377–1382
Acknowledgments
This work was supported by the National Institutes of Health. We thank Dr. Vishva Dixit (Genentech, South San Francisco, CA) for providing dominant negative FADD.
Author information
Authors and Affiliations
Corresponding author
Rights and permissions
About this article
Cite this article
Shankar, S., Siddiqui, I. & Srivastava, R.K. Molecular mechanisms of resveratrol (3,4,5-trihydroxy-trans-stilbene) and its interaction with TNF-related apoptosis inducing ligand (TRAIL) in androgen-insensitive prostate cancer cells. Mol Cell Biochem 304, 273–285 (2007). https://doi.org/10.1007/s11010-007-9510-x
Received:
Accepted:
Published:
Issue Date:
DOI: https://doi.org/10.1007/s11010-007-9510-x