Abstract
A tri-arm star-shaped oligomer with PEG arm and imidazolium-based ionic liquid moiety (TSIL) was introduced in poly(lactic acid) (PLA) blends, and the synergetic effects of PEG arm and IL moiety contained in the TSIL on the crystallization behaviors of PLA were investigated by DSC and POM. The results determined by DSC show that TSIL inhibits the crystallization of PLA when the mass content of TSIL in blend is as low as 1 mass%, while the crystallization process is accelerated greatly with increasing mass content of TSIL. As revealed by POM observations, the spherulitic morphology remains unchanged in TSIL/PLA blends and the nucleation and growth of spherulites are altered due to the different effects of PEG arm and IL moiety. The nucleation process is suppressed by IL moiety, while the growth rate of spherulites is improved because of the plasticizing effect of PEG arm. The synergistic effects of PEG arm and IL moiety contained in the TSIL also contribute to the acceleration of kinetics in non-isothermal crystallization process.
Similar content being viewed by others
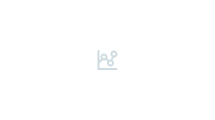
Explore related subjects
Discover the latest articles, news and stories from top researchers in related subjects.Avoid common mistakes on your manuscript.
Introduction
Poly(lactic acid) (PLA) is a kind of biodegradable aliphatic polyester, which is produced from renewable resources, such as corn, wheat straw. Since PLA is degradable and derived from sustainable resources, it has been viewed as a promising candidate to reduce the solid-waste disposal problem. However, several drawbacks of PLA including brittleness, poor thermal resistance, low heat distortion temperature and crystallization rate have limited its technological applications [1–5].
Introduction of plasticizers is commonly used to improve the physical properties of PLA. Various types of plasticizers such as citrate ester, triacetin, poly(ethylene glycol) (PEG), oligomeric lactic acid, glycerol, tributyl citrate, and partial fatty acid ester were found to be efficient plasticizers for PLA [6–13]. Among the above-mentioned plasticizers, PEG is the most suitable material for PLA modification due to its miscibility, biodegradability and food contactable applications [14–16]. Martin and Averous [17] investigated the influence of PEG chain length on the plasticization of PLA and found that shorter PEG could reduce the glass transition temperature from 58 to 12 °C. In Kulinski’s work [18], the effect of different end groups on the plasticization of both amorphous and semicrystalline PLA was studied. It was observed that both PEG and PEG monomethyl ether (mPEG) could decrease T g, while different end groups of the plasticizers have no marked effect.
Recently, research efforts have been devoted to the utilization of ionic liquids (ILs) as efficient plasticizers or lubricants for polymer materials [19, 20]. ILs are salts with low melting temperature and typically consist of a bulky inorganic cation and an anion or vice versa. Due to their low toxicity, negligible vapor pressure, low inflammability, non-explosive, high electric conductivity and thermal stability, they are considered as “green” alternatives to volatile organic compounds (VOCs) in organic synthesis, catalysis, chromatography, extraction, electroanalytical chemistry and sensing [21–24]. Besides, ILs have been widely applied in the field of PLA blend. Chen et al. [25] have reported the plasticizing effect of three kinds of imidazolium-based ILs with different anion groups on PLA. PLA blend modified with IL containing [PF6] anion showed better ductility than those modified with IL containing [BF4] and [TFSI] anions, respectively. Park et al. [26] suggested that two phosphonium-based ILs with different anions could be potential plasticizers and/or lubricants for PLLA. Both ILs lowered the glass transition temperature of PLLA and enhanced its rheological characteristics as evidenced from the reduced viscosities and apparent phase separation and lubrication.
It is well known that the plasticizing effect can influence the crystalline structure and morphology of polymers and thus plays an important role in the eventual physical properties of the materials. Recently, Xu et al. [27] investigated the influence of 1-butyl-3-methylimidazolium dibutylphosphate ([BMIM][(C4H9O)2PO2]) on the crystallization behaviors of PLA. The results showed that T g of PLLA decreased distinctly with the increase of IL content due to the plasticizing effect of IL, and the cold crystallization ability of PLA also improved evidently with the addition of IL. They also demonstrated that IL could suppress the nucleation of PLA. In our previous work [28], the existence of 3-methyl-1-(ethoxycarbonylethyl) imidazolium tetrafluoroborate ([AOEMIm][BF4]) enhanced the crystallization of PLA and induced longer elongation at break as well as higher impact strength. The crystallization behavior of PLA will be changed by the introduction of PEG or PEO as a plasticizer. Wang et al. [29, 30] studied the influence of plasticizers, such as PEG and PEO, on the crystallization behavior of PLA in double-layer films. Their results suggested that the introduction of plasticizers would accelerate the spherulitic growth rate of the PLA and the efficiency of acceleration is depended on their compatibility with PLA. Lai et al. [31] investigated the effect of PEG end groups on the crystallization behaviors of PLA and discovered that the spherulitic growth rate and isothermal crystallization rate decreased in the order of PEG(2OH), PEG(1OH–1CH3) and PEG(2CH3). Kulinski et al. [18] reported that PEGs with M w of 400 and 600 could both decrease T g and increase the cold crystallization ability of PLLA efficiently.
For the purpose of investigating the combined effects of PEG and ionic liquids on the crystallization behaviors of PLA, a novel tri-arm star-shaped oligomer with PEG arm and imidazolium-based ionic liquid moiety (TSIL) was incorporated in the PLA matrix. It is noted that the branching structure was introduced into TSIL to inhibit aggregation and phase separation that occurred for linear PEG oligomer with imidazolium-based IL moiety in PLA matrix. The crystallization behaviors of PLA blends with various TSIL contents were investigated using differential scanning calorimetry (DSC) and polarized optical microscopy (POM). Furthermore, to better understand the effects of TSIL on the crystallization behaviors of PLA, isothermal and non-isothermal crystallization kinetics was analyzed according to Avrami equation and Mo model, respectively.
Experimental
Materials
Poly(lactic acid) (PLA, 4032D) used in the investigation was purchased from NatureWorks LLC, USA, with weight-average molecular mass (M w) of 1.4 × 105 g mol−1, number-average molecular mass (M n) of 7.9 × 104 g mol−1, the content of l-lactide about 98.6 mass% with density of 1.24 g cm−3. The tri-arm star-shaped oligomer with PEG arm and imidazolium-based ionic liquid moiety (TSIL) was synthesized and purified by column chromatography in our laboratory. The structure and the procedure for synthesis of TSIL are schematically shown in Scheme 1. The 1H NMR spectra of the intermediate products and TSIL can be seen in Fig. 1.
Synthetic routes employed for the preparation of poly(ethylene glycol) monomethyl ether tosylate (1), poly(ethylene glycol) monomethyl ether imidazole (2), 2-((2-chloroacetoxy)methyl)-2-ethylpropane-1,3-diyl bis(2-chloroacetate) (TTCA), and tri-arm star-shaped oligomer with PEG arm and imidazolium-based ionic liquid moiety (TSIL)
Sample preparation
To sum up the procedure, co-precipitation method was used to prepare the TSIL/PLA blends. Prior to solution blending, both PLA pellets and TSIL were dried in vacuum at 60 °C for 24 h. The TSIL/PLA blends were prepared as follows. First, PLA was dissolved in chloroform to form a solution with concentration of 5 mass%. Then, TSIL was added to the solution and stirred to form a homogeneous mixture. The mass content of TSIL in the blends was 0, 1, 5, 9 mass%, respectively. The codes for different samples are shown in Table 1. The solutions were poured into fivefold of cyclohexane to precipitate the blends. The precipitated blends were dried in the fume cupboard for 24 h followed by vacuum drying at 60 °C for 12 h before analyses.
Differential scanning calorimetry (DSC)
The crystallization behavior of the neat PLA and the TSIL/PLA blends was investigated using differential scanning calorimetry (DSC, TA Q2000, USA). In the isothermal process, the sample of 6–8 mg was heated to 180 °C at a heating rate of 50 °C min−1 and kept for 5 min to eliminate the thermal history. It is noted that the thermal history was erased at 180 °C to minimize the possible degradation of PLA samples in the process. Then, the sample was cooled down to a predetermined isothermal crystallization temperature at a cooling rate of 50 °C min−1, held at this temperature for 2 h to ensure the completion of crystallization, and then the sample was cooled down to room temperature. In the non-isothermal crystallization process, the sample of 6–8 mg was heated to 180 °C at a heating rate of 50 °C min−1 and kept for 5 min to eliminate the previous history. Then the sample was cooled down to −20 °C at a cooling rate of 1, 2, 3, 4 and 10 °C min−1, respectively.
Polarized optical microscopy (POM)
The growth of crystallized spherulites was investigated by polarized optical microscopy (POM). The sample was put on one cover glass on the hot stage at 180 °C at first. After the sample was melted, another cover glass was put on and pressed to make a film with thickness of about 35 μm. The film was held on the hot stage at 180 °C for 5 min to eliminate the thermal history and then transferred to another hot stage set at the crystallization temperature to study isothermal crystallization. From the slopes of the changes in spherulite radius with crystallization time, the radial growth rates of the spherulites of neat PLA and TSIL/PLA could be obtained.
Results and discussion
Effects of TSIL on the isothermal crystallization behaviors by DSC
The DSC heat flow curves of all samples during the isothermal crystallization process are shown in Fig. 2 At all crystallization temperatures, neat PLA exhibits relatively slower crystallization process and the crystallization peak is broad. However, as the mass content of TSIL increases, the peak of the crystallization curve slows down first when the mass content of TSIL is below 1 mass%. The crystallization process then becomes faster and the peak becomes much narrower when the mass content of TSIL is up to 5 and 9 mass%.
The isothermal crystallization kinetics of semicrystalline polymers can be well described by the Avrami equation (Eq. 1) [32–35]:
where X t is the relative degree of crystallinity defined as the ratio of crystallinity at any time. The X t of PLA is determined using Eq. 2 as follows:
n is the so-called Avrami exponent which depends on the type of nucleation and the growth mechanism during the crystallization, and K is a rate constant related to nucleation and growth rate parameters. The theory is based on the assumption that if the type of crystallization does not change, the Avrami equation is valid to describe the crystallization kinetics of polymers during the whole isothermal crystallization process.
After taking logarithm of the Avrami equation, Eq. 1 can be modified as follows:
Generally, the plot of ln [−ln(1 − X t)] versus ln t gives a straight line. The slope of the line is n, and the intercept with the ordinate yields ln K. Furthermore, from Eq. 3, the crystallization half time t 1/2 which is also used to describe the crystallization rate of polymers can be obtained using Eq. 4 as follows:
Figure 3 shows the changes in relative crystallinity with time during isothermal crystallization at different crystallization temperatures for neat PLA and TSIL/PLA blends. More apparently, the blend in which the mass content of TSIL is 9 mass% exhibits much faster crystallization rate compared to neat PLA. The crystallization of PLA in the blend can be achieved within about 5 min. The higher the mass content of TSIL in the blends, the shorter the time required for the completion of crystallization when the mass content of TSIL is higher than 1 mass%. Figure 4 shows the plot of ln [−ln(1 − X t)] versus ln t and Table 2 shows the corresponding parameters for the isothermal crystallization kinetics. The X t from 10 to 50 % is described in Eq. 3, because when the X t is close to 100 %, the plot is not a straight line anymore. [36, 37] As shown clearly in Fig. 4, the plots of all samples exhibit straight lines in initial stages of the crystallization.
Figure 5 shows the relationship between crystallization half time and crystallization temperature. The TSIL/PLA blends exhibit much smaller t 1/2 compared with the neat PLA when the mass content of TSIL is higher than 1 mass%, although the crystallization temperature set for the TSIL/PLA blends is much higher than that of the neat PLA, indicating that relatively higher mass content of TSIL accelerate the isothermal crystallization process of the PLA matrix enormously.
As shown in Table 2, the Avrami exponent n is in close agreement with values in literatures [38, 39] which lie in the range of 2.1–2.5. Therefore, TSIL did not change the spherulitic crystal growth in a three-dimension way. However, the variation in the trend of rate constant K proves the synergetic effects of PEG arm and the IL moiety of TSIL on the crystalline process of PLA. The K of PLA with 1 mass% is lower than that of neat PLA, indicating the synergetic effects are inhibiting the crystallization behavior of PLA. A higher K value can be obtained when the mass content of TSIL is higher than 1 mass%, suggesting an acceleration effect on the crystallization behavior of PLA. At a crystallization temperature of 90 °C, the value of K decreases from 0.45 × 10−3 to 0.32 × 10−3 when the mass content of TSIL changes from 0 to 1 mass%. But when the mass content of TSIL is 9 mass%, the value of K increases to 14.3 × 10−3.
Therefore, the changes in isothermal crystallization kinetics in TSIL/PLA blend can be attributed to the synergetic effects of PEG arm and the IL moiety in TSIL. The IL moiety can suppress the crystallization of polymer [40, 41], while the PEG arm can accelerate the crystallization process due to its capability to enhance segmental mobility of PLA. When mass content of TSIL in blend is as low as 1 mass%, the suppression effect of TSIL plays a major role in the crystallization process of PLA. When a higher mass content (e.g., 5 mass%) of TSIL is incorporated in the blend, the acceleration effect becomes dominant that increases the crystallization kinetics. The different effects of PEG arm and the IL moiety during isothermal crystallization process of TSIL/PLA blends will be illustrated in next section.
Effects of TSIL on nucleation and growth of the spherulites by POM
The nucleation and growth of spherulites for neat PLA and TSIL/PLA blends during isothermal crystallization process were observed by using a polarized optical microscopy (POM). Determination of the growth rate, Gs, of spherulites during isothermal crystallization process was performed by calculating the slope of data points in the plot of spherulite radius versus the crystallization time. For the purpose of obtaining results with good reliability, three spherulites, which are marked in Fig. 6 by red arrows, are selected in the calculation process and the results are shown in Fig. 7a–c.
As shown in Fig. 6, the spherulites are observed in the micrographs for neat PLA and TSIL/PLA blends with different mass content of TSIL, indicating that the incorporation of TSIL does not change the crystalline morphology of PLA in melt crystallization process. Meanwhile, the diameter of spherulites increases, while the number of spherulites decreases with the increasing of incorporated TSIL content. It can be observed in Fig. 7 that the value of Gs for neat PLA is about 0.8 μm min−1 and the value increases to 2.1, 3.2 and 5.0 μm min−1 with the increment of mass content of TSIL to 1, 5 and 9 mass%, respectively. The value of Gs for neat PLA is in good agreement with the reported values [42]. The above results indicate that the incorporation of TSIL has two different effects on the isothermal crystallization behaviors of PLA: inhibiting the nucleation process and accelerating the growth process of the spherulites. The isothermal crystallization kinetics of PLA is influenced by both nucleation and growth rate of spherulites. When the mass content of TSIL in TSIL/PLA blend is lower than 1 mass%, the inhibition effect of IL moiety in nucleation process is dominant, resulting in the formation of less number of spherulites and retarded overall crystallization kinetics as compared to that of neat PLA. When the mass content is higher than 1 mass%, the acceleration effect of PEG arm on spherulitic growth rate becomes significant, leading to higher spherulitic growth rate and formation of spherulites with bigger size as compared to that of neat PLA.
Effects of TSIL on the non-isothermal crystallization behavior by DSC
During the industrial production, polymeric materials usually crystallize in a non-isothermal manner. Therefore, it is significant to investigate the non-isothermal crystallization behavior of semicrystalline polymer. Figure 8 shows the cooling traces of the neat PLA and the TSIL/PLA blends obtained by DSC and the corresponding crystallization parameters are shown in Table 3. At cooling rate ranging from 1 to 10 °C min−1, the exothermic peak of PLA is much wider than those of TSIL/PLA blends even when the cooling rate is just 1 °C min−1. When the cooling rate is 10 °C min−1, no apparent exothermic phenomenon can be detected from the cooling curves of the neat PLA, indicating the difficulty of crystallization for neat PLA during the non-isothermal process. The presence of TSIL greatly improves the crystallization ability of PLA during the non-isothermal crystallization process; however, the crystallization behaviors of the TSIL/PLA blends are dependent upon the cooling rates.
The relative crystallinity (X t) evolution curves at different cooling rates in non-isothermal crystallization process are illustrated in Fig. 9. The results at a cooling rate of 10 °C min−1 are not considered because only the sample with 9 mass% mass content of TSIL exhibits crystallization behavior under this condition. More apparently, the crystallization rate of the TSIL/PLA blends is greatly dependent upon the mass content of TSIL. The higher the mass content of TSIL is, the higher the crystallization rate of the blend possesses. There is a little difference from the results obtained from isothermal crystallization process when the mass content of TSIL is 1 mass%.
The non-isothermal crystallization kinetics of the neat PLA and TSIL/PLA blends can be described by Mo model, which combines the Avrami equation and Ozawa equation [43–45]:
where ϕ is the cooling rate and the kinetic parameter F(t) refers to the value of cooling rate that has to be selected at unit crystallization time when the sample has the defined degree of crystallinity. α is the ratio of the Avrami exponent n to the Ozawa exponent m. The plots of log ϕ versus log t are shown in Fig. 10, and the data of F(t) and α, obtained from the intercept and slope of the plots, are shown in Table 3. From Fig. 10, it can be seen that for all samples, the curves show good linearity. It is suggested that the Mo model can provide a satisfactory description of the non-isothermal crystallization for both the neat PLA and TSIL/PLA blends. As shown in Table 3, the changing values of α indicate that the non-isothermal crystallization process involving the nucleation and spherulites growth of the neat PLA and TSIL/PLA blends can be influenced by the mass content of TSIL. The F(t) decreases evidently as the mass content of the TSIL increases, which further indicates that the introduction of TSIL can accelerate the crystallization process in the PLA matrix.
Conclusions
In summary, to study the synergetic effects of PEG arm and IL moiety contained in the tri-arm star-shaped oligomer (TSIL) on the crystallization behaviors of PLA, the isothermal crystallization and non-isothermal crystallization of the PLA samples with or without TSIL were investigated by DSC and POM. It is found that crystallization behaviors of PLA are changed with the incorporation of TSIL. The PEG arm and IL moiety play different roles in those processes. In the isothermal crystallization process, TSIL can retard the crystallization of PLA when the mass content is as low as 1 mass% in TSIL/PLA blend. But the crystallization process is accelerated enormously when the mass content of TSIL is increased to 5 and 9 mass%. The results of POM observations indicate that the spherulitic morphology still exists in TSIL/PLA blends, but the spherulite number and diameter at the same crystallization time are different in the samples with different mass content of TSIL due to the different effects of PEG arm and IL moiety. The nucleation process of PLA is suppressed at the presence of IL moiety, leading to fewer spherulite numbers in the micrographs for TSIL/PLA blends, while the growth rate of spherulites is improved because of the enhancement of chain mobility with the assistance of PEG arm, showing spherulites with bigger size in the micrographs for TSIL/PLA blends. The synergistic effects of PEG arm and IL moiety of TSIL on non-isothermal crystallization behaviors of PLA are also testified and analyzed by Mo model.
References
Rasal RM, Janorkar AV, Hirt DE. Poly(lactic acid) modifications. Prog Polym Sci. 2010;35:338–56.
Jiang L, Wolcott MP, Zhang JW. Study of biodegradable polylactide/poly(butyleneadipate-co-terephthalate) blends. Biomacromolecules. 2006;7:199–207.
Hao QH, Li FX, Li QB, Li Y, Jia L, Yang J, Fang Q, Cao AM. Preparation and crystallization kinetics of new structurally well-defined star-shaped biodegradable poly(l-lactide)s initiated with diverse natural sugar alcohols. Biomacromolecules. 2005;6:2236–47.
Lim LT, Auras R, Rubino M. Processing technologies for poly(lactic acid). Prog Polym Sci. 2008;33:820–52.
Hu Y, Hu YS, Topolkaraev V, Hiltner A, Baer E. Aging of poly(lactide)/poly(ethylene glycol) blends. Part 2. Poly(lactide) with high stereoregularity. Polymer. 2003;44:5711–20.
Henricks J, Boyum M, Zheng W. Crystallization kinetics and structure evolution of a polylactic acid during melt and cold crystallization. J Therm Anal Calorim. 2015;120:1765–74.
Kulinski Z, Piorkowska E, Gadzinowska K, Stasiak M. Crystallization, structure and properties of plasticized poly(l-lactide). Biomacromolecules. 2006;7:2128–35.
Tabi T, Suplicz A, Czigany T, Kovacs JG. Thermal and mechanical analysis of injection moulded poly(lactic acid) filled with poly(ethylene glycol) and talc. J Therm Anal Calorim. 2014;118:1419–30.
Ljungberg N, Wesslen B. Preparation and properties of plasticized poly(lactic acid) films. Biomacromolecules. 2005;6:1789–96.
Ljungberg N, Andersson T, Wesslen B. Film extrusion and film weldability of poly(lactic acid) plasticized with triacetine and tributyl citrate. J App Polym Sci. 2003;88:3239–47.
Hu Y, Rogunova M, Topolkaraev V, Hiltner A, Baer E. Aging of poly(lactide)/poly(ethylene glycol) blends. Part 1. Poly(lactide) with low stereoregularity. Polymer. 2003;44:5701–10.
Hu Y, Hu YS, Topolkaraev V, Hiltner A, Baer E. Crystallization and phase separation in blends of high stereoregular poly(lactide) with poly(ethylene glycol). Polymer. 2003;44:5681–9.
Wei XF, Bao RY, Cao ZQ, Zhang LQ, Liu ZY, Yang W, Xie BH, Yang MB. Greatly accelerated crystallization of poly(lactic acid): cooperative effect of stereocomplex crystallites and polyethylene glycol. Colloid Polym Sci. 2013;292:163–72.
Sungsanit K, Kao N, Bhattacharya SN. Properties of linear poly(lactic acid)/polyethylene glycol blends. Polym Eng Sci. 2012;52:108–16.
Pillin I, Montrelay N, Grohens Y. Thermo-mechanical characterization of plasticized PLA: is the miscibility the only significant factor? Polymer. 2006;47:4676–82.
Baiardo M, Frisoni G, Scandola M, Rimelen M, Lips D, Ruffieux K, Wintermantel E. Thermal and mechanical properties of plasticized poly(l-lactic acid). J App Polym Sci. 2003;90:1731–8.
Martin O, Averous L. Poly(lactic acid): plasticization and properties of biodegradable multiphase systems. Polymer. 2001;42:6209–19.
Kulinski Z, Piorkowska E. Crystallization, structure and properties of plasticized poly(l-lactide). Polymer. 2005;46:10290–300.
Rahman M, Brazel CS. Ionic liquids: new generation stable plasticizers for poly(vinyl chloride). Polym Degrad Stab. 2006;91:3371–82.
Park KI, Xanthos M. A study on the degradation of polylactic acid in the presence of phosphonium ionic liquids. Polym Degrad Stab. 2009;94:834–44.
Huddleston JG, Visser AE, Reichert WM, Willauer HD, Broker GA, Rogers RD. Characterization and comparison of hydrophilic and hydrophobic room temperature ionic liquids incorporating the imidazolium cation. Green Chem. 2001;3:156–64.
Hallett JP, Welton T. Room-temperature ionic liquids: solvents for synthesis and catalysis. Chem Rev. 2011;111:3508–76.
Lovelock KRJ, Villar-Garcia IJ, Maier F, Steinruck HP, Licence P. Photoelectron spectroscopy of ionic liquid-based interfaces. Chem Rev. 2010;111:5158–90.
Pandey S. Analytical applications of room-temperature ionic liquids: a review of recent efforts. Anal Chim Acta. 2006;556:38–45.
Chen BK, Wu TY, Chang YM, Chen AF. Ductile polylactic acid prepared with ionic liquids. Chem Eng J. 2013;215–216:886–93.
Park KI, Ha JU, Xanthos M. Ionic liquids as plasticizers/lubricants for polylactic acid. Polym Eng Sci. 2010;50:1105–10.
Wei T, Pang SJ, Xu N, Pan L, Zhang Z, Xu R, Ma N, Lin Q. Crystallization behavior and isothermal crystallization kinetics of PLLA blended with ionic liquid, 1-butyl-3-methylimidazolium dibutylphosphate. J App Polym Sci. 2015;132:41308–19.
Gui HG, Li Y, Chen SY, Xu P, Zheng B, Ding YS. Effects of biodegradable imidazolium-based ionic liquid with ester group on the structure and properties of PLLA. Macromole Res. 2014;22:583–91.
Zhang YQ, Xu HJ, Yang JJ, Chen SY, Ding YS, Wang ZG. Significantly accelerated spherulitic growth rates for semicrystalline polymers through the layer-by-layer film method. J Phys Chem C. 2013;117:5882–93.
Zhang YQ, Wang ZK, Jiang F, Bai J, Wang ZG. Effect of miscibility on spherulitic growth rate for double-layer polymer films. Soft Matter. 2013;9:5771–8.
Lai WC, Liau WB, Lin TT. The effect of end groups of PEG on the crystallization behaviors of binary crystalline polymer blends PEG/PLLA. Polymer. 2004;45:3073–80.
Avrami M. Kinetics of phase change. I. General theory. J Chem Phys. 1939;7:1103–12.
Avrami M. Kinetics of phase change. II Transformation-time relations for random distribution of nuclei. J Chem Phys. 1940;8:212–24.
Avrami M. Granulation, phase change, and microstructure kinetics of phase change. III. J Chem Phys. 1941;9:177–84.
Bessard E, De Almeida O, Bernhart G. Unified isothermal and non-isothermal modelling of neat PEEK crystallization. J Therm Anal Calorim. 2014;115:1669–78.
Chaurasia SK, Singh RK, Chandra S. Effect of ionic liquid on the crystallization kinetics behaviour of polymer poly(ethylene oxide). CrystEngComm. 2013;15:6022–34.
Shalu Chaurasia SK, Singh RK. Crystallization behaviour of polymeric membrane based on polymer PVDF-HFP and ionic liquid BMIMBF4. RSC Adv. 2014;4:50914–24.
Wu DF, Wu L, Zhou WD, Zhang M, Yang T. Crystallization and biodegradation of polylactide/carbon nanotube composites. Polym Eng Sci. 2010;50:1721–33.
Shi J, Lu X, Li H, Li D. Isothermal crystallization kinetics and melting behavior of PLLA/f-MWNTs composites. J Therm Anal Calorim. 2014;117:1385–96.
Chaurasia SK, Singh RK, Chandra S. Ionic liquid assisted modification in ionic conductivity, phase transition temperature and crystallization kinetics behaviour of polymer poly(ethylene oxide). Solid State Ionics. 2014;262:790–4.
Gupta AK, Singh RK, Chandra S. Crystallization kinetics behavior of ionic liquid [EMIM][BF4] confined in mesoporous silica matrices. RSC Adv. 2014;4:22277–87.
Chen SY, Zhang YQ, Fang HG, Ding YS, Wang ZG. Can spherulitic growth rate accelerate before impingement for a semicrystalline polymer during the isothermal crystallization process? CrystEngComm. 2013;15:5464–75.
Liu TX, Mo ZS, Wang SE, Zhang HF. Nonisothermal melt and cold crystallization kinetics of poly(aryl ether ether ketone ketone). Polym Eng Sci. 1997;37:568–75.
Qiu ZB, Mo ZS, Yu YY, Zhang HF, Sheng SR, Song CS. Nonisothermal melt and cold crystallization kinetics of poly(aryl ether ketone ether ketone ketone). J App Polym Sci. 2000;77:2865–71.
Huang G, Zou Y, Luo W, Xiao M, Han D, Wang S, Meng Y. Nonisothermal crystallization behavior and kinetics of poly(l-lactide-co-propylene carbonate). J Therm Anal Calorim. 2015;121:877–83.
Acknowledgements
The authors acknowledge the financial support from the National Natural Science Foundation of China (NSFC) with Grant Nos. 51373045 and 51503055.
Author information
Authors and Affiliations
Corresponding authors
Rights and permissions
About this article
Cite this article
Li, Y., Fang, H., Zhang, D. et al. Synergetic effects of PEG arm and ionic liquid moiety contained in the tri-arm star-shaped oligomer on the crystallization behaviors of poly(lactic acid). J Therm Anal Calorim 125, 849–860 (2016). https://doi.org/10.1007/s10973-016-5481-z
Received:
Accepted:
Published:
Issue Date:
DOI: https://doi.org/10.1007/s10973-016-5481-z