Abstract
In the last decades some scientific papers have been published on the study of nuclear transmutation reactions in condensed matter, in which the formation of new nuclear products was investigated. In this contest, a specific research activity based on electrolysis process has been developed at ENEA: each step has been analyzed and deeply investigated by Instrumental Neutron Activation Analysis (INAA). The materials such as electrolysis equipment, electrolytic solutions, electrodes, etc. were preliminarily analyzed by INAA for identifying and reducing all the pollution sources. Several experiments were performed using films of copper, nickel and palladium as electrodes. At the end of each test, all the materials and the electrolytic solutions used and the blank as well were analyzed by INAA for checking and measuring the presence of nuclei originating from nuclear transmutations. INAA is in fact able to determine element content at a very low level, where other analytical techniques cannot reach with such precision and accuracy. The results obtained are strongly random demonstrating a phenomenon of apparent enrichment rather than the occurrence of transmutation phenomena in the condensed matter during the electrolysis.
Similar content being viewed by others
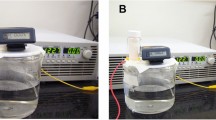
Explore related subjects
Discover the latest articles, news and stories from top researchers in related subjects.Avoid common mistakes on your manuscript.
Introduction
Since many years it has been well-known an important anomaly occurring during electrolysis process: the unexpected heat excess. In particular, in 1989 Fleischmann and Pons communicated the discovery that deuterium nuclei (D), confined in the metallic palladium (Pd) lattice, gave rise to nuclear fusion reactions at room temperature (cold fusion) [1]. They reported the astonishing results of watts of power from cold deuteron fusion without large amounts of radiation. The phenomenon provided primarily excess of power (as the production of heat excess) in Pd cathodes loaded with deuterium, during electrolysis process using D2O [2–5]. This heat excess could not be explained only through electrochemical reactions. Over the last decades [3, 5–15] several studies have supported the idea that in condensed matter nuclear processes could occur at low energy in different ways than those typical of thermo-nuclear fusion [16]. In particular, some authors [12–14] indicated the possibility of formation of new species through induced nuclear transmutation phenomena in condensed matter. After electrolysis experiments on mono- or multi-layer (Pd and Ni) thin films, performed using both light water and heavy water and various electrolytes (e.g., Na2CO3 or LiOH), Miley [12, 13] and Bockris [14] found by means of INAA, Secondary Ion Mass Spectrometry (SIMS), Energy Dispersive X-ray (EDX) analysis and Auger Electron Spectroscopy (AES) that new atomic species were observed at very low levels. They identified Ag, Al, As, Ba, Cd, Co, Cr, Cu, Dy, Eu, Fe, Ga, Gd, Ge, Ho, In, Mg, Mn, Mo, Nb, Pd, Rb, Sb, Se, Si, Sm, Sn, Sr, Te, Ti, V, Y, Yb and Zn as products of nuclear transmutation occurred in the thin films. It should be underlined that these are the only papers in this field at authors’ knowledge where INAA is applied for investigating the presence of such species.
Starting from this hypothesis and in order to test the reproducibility (as well as the reliability) of the data presented in the literature, the authors decided to undertake a series of experiments using Instrumental Neutron Activation Analysis (INAA), especially oriented to discriminate between the formation of new species by nuclear reaction and the presence of contaminants from the environment. The cleanliness level of both the single components and the apparatus in toto was investigated. A selection of the materials to be used in the experiments was performed by INAA, leading to the choice of the ones characterized by a minimum content of impurities. For each experiment, blank samples of both the electrodes and the electrolyte solutions were prepared and analyzed by INAA, to guarantee a proper reference for the analysis of the operated electrodes and the exhausted electrolyte solutions.
Among the analytical techniques, the INAA presents many advantages which make it very suitable for the present study. It is a non-destructive analytical method (i.e., all samples can be stored and analyzed again) and highly sensitive (very low limit of detection (LOD), high sensitivity and high precision) (Table 1). Furthermore, it is a bulk method (INAA is an analytical technique that allows to analyze the entire mass of the sample) [19], and it allows the determination in the same sample up to 50–60 elements with no chemical manipulations (no errors due to excess contamination, positive artifacts, or down due to loss of material, negative artifacts). In this study we analyzed Ag, Al, As, Au, Br, Cl, Co, Cr, Cu, Eu, Fe, Ga, Hf, Mg, Mn, Mo, Na, Ni, Pd, Sb, Se, Sm, Ta, V, W, Zn.
Experimental part
Electrolysis process
Equipments
The electrolysis cell (Fig. 1) consisted in a 15 mL polyethylene vessel, that was made from a pure polyethylene (PE) (Kartell S.p.A., Milan, Italy) container of the same kind of which are generally used as sample-holders during INAA analysis. The anode was a Pt wire (99.98% pure Pt) wrapped in the shape of a planar spiral. The cathode was a metallic thin film containing Cu, or Ni, or Pd, deposited onto a polyethylene disk (12 mm diameter and 1 mm thickness) made of the same ultrapure material of the cell vessel. Before deposition, the substrates were chemically cleaned and ion beam etched to improve film/substrate adhesion and to assure good film surface status after electrolysis (1 min using oxygen gas with ion energy of 290 eV, ion current of 0.6 mA at pressure of 5 × 10−4 torr). For each experiment, the films were prepared by ion beam sputtering contemporaneously over two adjacent PE substrates to obtain a blank film electrode with the same deposition of the working one (Fig. 2). The sputtering process was carried out at room temperature and 2.5 × 10−5 torr pressure; the sputtering beam was made by Ar+ ions with 1.2 keV ion beam energy and 20 mA ion beam current; the sputtering growth rate, measured by profilometry of calibration samples, was: 0.184 nm s−1 for Cu, 0.126 nm s−1 for Ni, and 0.245 nm s−1 for Pd films. The cathode contact was made of a “C” shape platinum wire inserted into a PE support to prevent the electrolysis between anode and cathode connections. The electrolysis conditions were: time duration: 1–5 h; LiSO4 solution concentration: 1 M (except than for sample #9 for which it was 10−3M); cell current 5–190 mA; cell voltage: 3–7 V.
Before the process, all the equipment was cleaned. The cleaning procedure was the following: washing with 18 MΩ × cm−1 ultrapure distilled deionized water; washing with HNO3 65% (Merck Specpure, Darmstadt, Germany) for 1 min; rinsing with 18 MΩ × cm−1 ultrapure distilled deionized water; rinsing with ethanol (ultrapure reagent by Carlo Erba, Milan, Italy); rinsing several times with 18 MΩ × cm−1 ultrapure distilled deionized water; drying at room temperature in a vertical laminar flow aspirator. The Pt wire was cleaned by acetone and ethylene in a previous supplementary step. The tools used to assemble the cell components were selected to avoid the presence of contaminants. The film preparation, cleaning procedure, cell assembly and all the experiments were performed in a class 1000 clean room using dust-free gloves (Nitrilite Silky UC 93-222, Ansell, Canada). The only exception concerned the operation of the electrolysis experiments and the consequent de-assembling of the electrolytic cells into INAA ultra-clean irradiation vessels in the first series, which were conducted in a normal chemical lab.
Electrolysis experiments
Three series of experiments were performed: (1) mono-layer (Cu, Ni, Pd), double-layer (Cu/Ni) and multi-layer (Cu/Ni/Pd/Ni/Pd) thin films electrodes, using electrolyzing solution of Li2SO4 1 M (ultrapure compounds dissolved in 18 MΩ × cm−1 ultrapure distilled deionized water); (2) mono (Cu, Ni) and double (Cu/Ni and Cu/Pd) layer electrodes, using the same electrolytic solution (Li2SO4) at the same concentration (1 M) in all but one experiments (exp. #9, 10−3 M); (3) mono (Ni) and double (Cu/Pd) layer electrodes using Li2SO4 1 M as electrolyzing solution (Table 2). In all the experiments the thickness of the films deposited on the PE substrates was kept fixed at: 45 nm for mono-layer, 25/45 nm for double-layer, and 25/45/45/45/45 nm for multi-layer films, respectively.
INAA analysis
Sample and standard preparation
After electrolysis, the thin-film cathodes were removed from the cell vessel in the clean room; the spent cathodes and the relative blanks were put directly into two separate irradiation vessels, then transferred into the TRIGA facility where they were irradiated and analyzed. The mass increment due to the electrolysis was computed, for each element, by subtracting from the inventory of the electrolyzed cathode that of the corresponding blank sample. Samples from all the materials used in the experiments (PE supports, Pt wire, lab gloves and lab papers) were put into the irradiation vessel in the clean room and then irradiated and analyzed in the same conditions of the operated samples. As concerning the electrolytic solutions, the irradiation and analysis were carried out at the same conditions on 1–2 mL of spent and fresh-made solution, stored into separate irradiation vessels in the clean room and then desiccated in stove at 40 °C.
The standards for INAA calibration were prepared starting from calibrated solutions 1000 μg mL−1 of each pure element (Carlo Erba). Known quantities of each solution were exactly dosed by means of a micropipette (Eppendorf) into the irradiation vessel, gently dried in stove at 40 °C, and irradiated.
Irradiation and measurements
The samples were submitted to two different neutron irradiations: (1) in the pneumatic channel (Rabbit) of the TRIGA MARK II reactor at the R.C. ENEA-Casaccia for 1 min at neutron flux of 1.25 × 1013 n cm−2 s−1 (fluence 7.5 × 1014 n cm−2) for measuring 108Ag, 28Al, 80Br, 38Cl, 66Cu, 27Mg, 56Mn, 24Na, 109mPd, 52V and 187W; (2) in the rotating rack (Lazy Susan) of the TRIGA MARK II reactor for 30 h at neutron flux of 2.6 × 1012 n cm−2 s−1 (fluence 2.8 × 1017 n cm−2) for measuring 110mAg, 76As, 198Au, 82Br, 58Co(Ni), 60Co, 51Cr, 64Cu, 152Eu, 59Fe, 72Ga, 181Hf, 192Ir, 99Mo, 109Pd, 122Sb, 75Se, 153Sm, 182Ta, and 65Zn. The rack was maintained in constant rotation to make consistent and uniform the flow and the energy spectrum of the incident neutrons.
The γ measurements were carried out by means of a counting system constituted by a HPGe detector (EG&G Ortec, Oak Ridge, TN, USA; FWHM 1.70 keV at 1332.50 keV, relative efficiency 23%, peak to Compton ratio 58:3), an ORTEC 672 amplifier and a multichannel buffer ORTEC 918A connected to 8 k channel analyzer through data acquiring system ADCAM MAESTRO II. The energy and efficiency calibrations were performed by 137Cs–60Co (furnished by Laboratorio Metrologia delle Radiazioni Ionizzanti, ENEA-Casaccia) and 152Eu (furnished by Centre Energie Atomique, France) sources, respectively.
Results and discussion
The reproducibility of the INAA measurements was tested on the pure standards of Ag, Cr, Co, Fe and Zn, arranged throughout the irradiation positions of the rotating rack. The high reproducibility of the specific activities of 110mAg and 65Zn (40.7 ± 1.8 Bq μg−1 with RSD 4.3 and 133.3 ± 2.3 Bq μg−1 with RSD 1.7, respectively) can be inferred as a demonstration that the irradiation and signal collection were uniform over the different position of the Lazy Susan.
Tests on electrodes, solutions and laboratory equipment
In addition to the electrodes and electrolytic solutions, all the materials which could have been in contact with them during the experiments were tested by INAA. In particular, we analyzed: blank electrodes, electrolytic solutions, uncoated PE substrates, Pt wire, lab gloves and lab paper used to handle the cells components and sputtered films. The results have been extensively discussed in a previous paper [20]: here, we would like to report briefly the main considerations that can be useful for the discussion of the impurity levels found after the electrolysis processes.
Table 3 shows the amount of impurities determined after Lazy Susan irradiations in all the equipment used for the experiments (laboratory papers, PE after etching, gloves, Pt wire), and the electrolyzing solutions (Li2SO4 1 M and 10−3 M). The main considerations concern the levels of Zn in lab gloves, Ta and Zn in lab paper and Ag, Ir, Ta and Zn in Pt wire. The highest Zn level in laboratory gloves is due to ZnO or ZnSO4 present inside the gloves as lubricant. Further contamination by Cr, Hf and Zn in the PE support may come from the etching process (i.e., mould).
Additional INAA analysis after Rabbit irradiation was performed on the electrolytic solutions. The results (Table 3) showed a moderate amount of Al, Br and Na, indicating a lower cleanliness of the electrolytic solution respect to the blank cathodes (see Tables 4, 5, 6 and discussion below). The quite high background signal due to the intense Al line emission, prevents the possible detection of the V signal, that was not detectable above an equivalent V content of about 10 ng mL−1 in the Li2SO4 1 M solution. Since the possible contamination from this element was considered of particular interest on the basis of the results of the electrolyzed samples (see next paragraph), further analysis by Inductively Coupled Plasma Optical Emission Spectroscopy (ICP-OES) was performed. The results showed a V concentration of 7 ng mL−1 in a sample of fresh Li2SO4 solution 1 M.
The blank electrodes, reported in Tables 4, 5, 6, were basically clean. Typical environmental contaminants, such as Al, Cl and Na, were at very low levels as well as Br, considered an ubiquitous pollutant, confirming the high levels of cleanness of the entire process. The interesting issue regards significant activities of Cu and Pd in mono-layer Ni electrodes, and of Ni and Pd in mono-layer Cu electrodes, due to a memory effect from the previous sputtering processes. Co, Cr, Fe, Mn, Mo and W were systematically present in all the samples and their levels increased in relationship with the film thickness and the film deposition time (see Fig. 3). The presence at trace level of Ag, Hf and Zn can be related to possible contamination from the sputtering chamber (due to previous deposition of Ag, Fe, Hf from HfO2, and Zn). Ag, Sb, Ta and Zn were also present in the laboratory paper, as we have discussed above. Furthermore, the presence of Au, As, Eu, Se and Sm at very low levels shows how unexpected contaminants can be always present and affect the transmutation investigations with positive artifacts.
Impurity determination in the three electrolysis experiments
Table 4 shows the increment (expressed as ng) of the elements detected by INAA in the first series of cathodes, after electrolysis with Li2SO4 1 M electrolytic solution; the values measured in the corresponding blank samples are also shown for comparison, in order to highlight the increase of each species respect to the original value.
The increase in the levels of Al, Cl and Na in the electrolyzed samples is quite scattered and below a few sigma from the background value of the blank sample. Since these elements are typical environmental contaminants, it can be deduced that the cleanness of the experimental environment in which electrolysis was performed was comparable with that of the sputtering process, both remaining quite low.
The systematic increment in the Zn level can be easily explained by contamination from the gloves used to handle the cathodes during cell assembling. This observation is reproduced also in the other two series of experiments (Tables 5 and 6), thus confirming this explanation.
The most reproducible and noticeable results concern the increase of the V level: the V amount ranges between 209 and 287 ng in all samples except than for the mono Pd-layer (25 ng), which are well above the background values of the blank samples (<15 ng). This element shows also a moderate increase in the third series of experiments (#12, #13, #14 in Table 6), although to a lower extent (<60 ng); it was not measured in the second series of experiments, because only long time neutron irradiations were performed in that case.
A possible explanation of these observations can be found in the presence of this element in the Li2SO4 electrolyte, as confirmed by the ICP-OES analysis. In fact, the increase of V in the electrolyzed samples could be due to surface adsorption or residue from the electrolytic solution. This hypothesis is further supported by the fact that the V increase is correlated with the increase of manganese (see Tables 4 and 6).
The higher level of environmental contamination should have been expected in the first series of samples than in the others, since in that case the cells were de-assembled, after electrolysis, not in clean room but in normal laboratory. Furthermore, it is worth noting that the increase in the V level is not correlated to the mass number of the film matrix element and this is also pointing to the direction of contamination as its possible source.
Other considerations can be applied to Cr, Fe, Mo, Mn, W, whose presence in the blank samples has been already traced to contamination from the sputtering process. The increase of these impurities respect to the blank values was not correlated with the electrolysis parameters (cell current and voltage, total faradic charge and time duration) and its amount was within a few sigma from the values of the blank background; for these reasons, such values can be considered within the statistical fluctuations of the contaminants coming from the electrode preparation process.
The levels of the other elements were around or below the LOD (e.g., As), with the only exception of sample #2, in which the levels of Ag and Au showed a moderate increase over the blank values (310 ng for Ag and 37 ng for Au, respectively). The presence of Ag can be explained here by electrochemical deposition of this specie following anodic erosion of the platinum anode, that, indeed, did contain a moderate amount of Ag, as reported in Table 3. Actually, the total electric charge flowed during this experiment was the higher in the series; furthermore, the presence of Ni cathode may have favored the Ag deposition from the solution to the cathode surface, since Ni delivered into the electrolyte is weakly competing with Ag in cathodic process.
The same arguments can be used to justify the increased amount of Ag in samples #7 and #10 of the second series (Table 5); in fact, both experiments were characterized by high total electric charge (1029 and 2010 C, respectively) and by the presence of Ni in the cathode film.
The higher Au increase in sample #2 could come from anodic Pt wire dissolution, being Au a typical contaminant of Pt, although it was not measured in the bare Pt wire because this last was analyzed by INAA only in the Lazy Susan configuration. Contamination by anodic corrosion of the Pt anode also occurred in the samples of second series, in which the Ir increase after electrolysis is a clear signature of anode dissolution, being Ir the more abundant impurity of the Pt wire. The presence at trace level of Ag and Ta, which were the other main contaminants of the anode material, may analogously be due to the same process.
In the third series of experiments the INAA investigation was conducted on as many elements as possible, as shown in Table 6. Although the background spectrum was significantly higher due to the Compton emission, the electrodes of these third series were less contaminated after electrolysis, probably due to the increased ability in avoiding the occasional contaminations. There were no species detectable above the background other than those already detected in the previous series of experiments, and their levels were lower than in the previous cases.
In summary, looking at the results of Tables 4, 5, 6, elements such as Ag, Al, Au, Br, Cl, Co, Cr, Cu, Fe, Hf, Ir, Mn, Mo, Na, Ni, Pd, Sb, Se, Ta, V, W, Zn were present both in the blank and electrolyzed electrodes. Some of them, which increase after electrolysis, can be anyway traced to contamination or anodic dissolution.
In particular, it should be noted that, except than in the case of evident electrochemical deposition from anodic dissolution, the amount of contaminants is not correlated with the total faradic charge flown through the cells, that is indicative of the hydrogen mass transfer through the sample, as it should be expected on the basis of the assumption that transmutation reactions involving hydrogen had occurred.
Conclusion
INAA was useful to investigate the eventual formation of new species attributable to transmutation phenomena. Resuming the results, it can be evidenced that: (a) the Ag, Au, Ir and Ta levels are due to contamination from platinum wire anodic dissolution; the higher Ag amount in specific samples could also be explained by favored cathodic deposition of this specie at the cathode surface; (b) the presence of Cr, Hf, Fe, Mo, and W is likely due to mould during the etching process and to their participation to the thin-film preparation; (c) the low levels of the typical environmental contaminants (Cl, Br, Na and Mn) indicated that the experiments have been carried on in a clean environment; (d) the increment in the Zn level can be explained by contamination from the gloves used to handle the cathodes during cell assembling. The increments in the electrolyzed cathodes do not seem to be correlated with the electrolysis parameters, but rather random: then, the hypothesis that transmutation phenomena could be responsible for the detected impurities is not supported.
References
Fleishmann M, Pons S (1989) Electrochemically induced nuclear fusion of deuterium. J Electroanal Chem 261:301
Bertalot L, Bettinali L, De Marco F, Violante V, De Logu P, Dikonimos Makris T, La Barbera A (1991) In: Bressani T, Del Giudice E, Preparata G (eds) Proceedings of the II annual conference on cold fusion. SIF, vol 33. Como, p 3
Miles MH, Bush BF, Ostrom GS, Lagowsky JJ (1991) In: Bressani T, Del Giudice E, Preparata G (eds) Procedings of the II annual conference on cold fusion. SIF, vol 33. Como, p 363
McKubre MCH, Rocha-Filho R, Smedley SI, Tanzella FL, Crouch-Baker S, Passell TO, Santucci J (1991) In: Bressani T, Del Giudice E, Preparata G (eds) Procedings of the II annual conference on cold fusion. SIF, vol 33. Como, p 419
Gozzi D, Cellucci F, Cignini PL, Gigli G, Tomellini M, Cisbani E, Frullani S, Urciuoli GM (1998) J Electroanal Chem 452:253
Scaramuzzi F (2000) Account Res 8:77
Jones SE, Scaramuzzi F, Fabrizio M, Manduchi C, Mengoli G, Milli E, Zannoni G (1993) In: Stella B (ed) Proceedings of the Rome workshop on the status of cold fusion in Italy, vol 74, Rome
Yamaguchi E, Nishioka T (1992) In: Ikegami H (ed) Proceedings of the 3rd international conference on cold fusion, vol 179, Nagoya
Taylor SF, Claytor TN, Tuggle DG, Jones SE (1994) Fusion Technol 26T:180
Aoki T, Kurata Y, Ebihara H, Yoshikawa N (1994) Fusion Technol 26T:214
Violante V, Santoro E, Sarto F, Capobianco L, Rosada A (2003) Energia Ambiente Innovazione 2:38
Miley GH, Patterson A (1996) J New Energy 1:5
Miley GH, Narne G, Williams MJ, Patterson A, Nix J, Cravens D, Hora H (1996) In: Okamoto M (ed) Proceedings of the 6th international conference on cold fusion, vol 629, Lake Toya, Japan
Bockris J, Lin GH (1996) J New Energy 1:111
Iwamura Y, Itoh T, Sakano M (2000) In: Scaramuzzi F (ed) Proceedings of the 8th international conference on cold fusion, vol 141, Lerici
Goodstein DL (1994) Whatever happened to cold fusion. The American Scholar, 63 (1994) 527. also in: Engineering and Science, autumn 1994
Currie LA (1968) Anal Chem 40:586
Leclerc JC, Cornu A (1989) Neutron activation analysis table. Heyden, London
Avino P, Capannesi G, Rosada A (2006) Toxicol Environ Chem 88:633
Rosada A, Santoro E, Sarto F, Violante V, Avino P (2009) In: Proceedings of the 15th international conference on condensed matter nuclear science, Rome, Italy, in press
Acknowledgments
The additional analysis performed to check the V content of the electrolytic solution have been provided by Dr. C. Zoani and Dr. G. Zappa (ICP-OES), whose collaboration is greatly acknowledged. The technical support by Dr. Lecci, Ing. E. Castagna and M. Sansovini is gratefully acknowledged.
Author information
Authors and Affiliations
Corresponding author
Rights and permissions
About this article
Cite this article
Avino, P., Santoro, E., Sarto, F. et al. Neutron activation analysis for investigating purity grade of copper, nickel and palladium thin films used in cold fusion experiments. J Radioanal Nucl Chem 290, 427–436 (2011). https://doi.org/10.1007/s10967-011-1296-3
Received:
Published:
Issue Date:
DOI: https://doi.org/10.1007/s10967-011-1296-3