Abstract
During the last two decades, sediments collected in different sources of water bodies of the Tehuantepec Basin, located in the southeast of the Mexican Pacific Coast, showed that concentrations of heavy metals may pose a risk to the environment and human health. The extractable organic matter, geoaccumulation index, and enrichment factors were quantified for arsenic, cadmium, copper, chromium, nickel, lead, vanadium, zinc, and the fine-grained sediment fraction. The non-parametric SiZer method was applied to assess the statistical significance of the reconstructed metal variation along time. This inference method appears to be particularly natural and well suited to temperature and other environmental reconstructions. In this approach, a collection of smooth of the reconstructed metal concentrations is considered simultaneously, and inferences about the significance of the metal trends can be made with respect to time. Hence, the database represents a consolidated set of available and validated water and sediment data of an urban industrialized area, which is very useful as case study site. The positive matrix factorization approach was used in identification and source apportionment of the anthropogenic heavy metals in the sediments. Regionally, metals and organic matter are depleted relative to crustal abundance in a range of 45–55 %, while there is an inorganic enrichment from lithogenous/anthropogenic sources of around 40 %. Only extractable organic matter, Pb, As, and Cd can be related with non-crustal sources, suggesting that additional input cannot be explained by local runoff or erosion processes.
Similar content being viewed by others
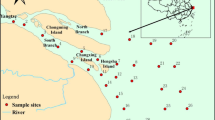
Explore related subjects
Discover the latest articles, news and stories from top researchers in related subjects.Avoid common mistakes on your manuscript.
Introduction
The significance of sediment contamination to the overall condition of the aquatic environment has long been recognized. Indeed, sediments represent a vast sink for contaminants that can serve as a reservoir of toxic compounds that continually threaten the health and viability of aquatic biota and have the potential to provide a starting point for entry into the aquatic food web (Kosmehl et al. 2008; Mai et al. 2003; Geffard et al. 2003).
Marine sediments contain metals derived from a variety of sources including (1) point sources associated with definable acute or long-term anthropogenic activities, such as urban and industrial discharges, and (2) non-point sources, such as atmospheric fallout of combustion-derived particles, or general surface runoff from the surrounding urban or industrial communities (Birch et al. 1996).
Sediment pollution sources can be addressed by different means including the geochemical index (I geo) or enrichment factors (EF), as well as receptor models. Enrichment factors consider normalization with conservative elements as an indication of contamination to discriminate natural variation from anthropogenic inputs (Pekey 2006; Zhou et al. 2007; Çevik et al. 2011; Ghrefat et al. 2011; Muñoz-Barbosa et al. 2012).
In the field of receptor modeling, when the sources are known and the compositions are either measured or otherwise available, the chemical mass balance approach would be the most appropriate (Chow and Watson 2002).
However, in most practical cases, no prior information is available; therefore, different models, including principal component analysis (PCA)/absolute principal component scores (Henry et al. 1984) and edge analysis (Henry 1997), have been applied to identify and establish the sources contributing to pollution in sediments (Peré-Trepat et al. 2007; Salau et al. 1997; Imamoglu and Christensen 2002; Sakurai 2003).
Customary methods such as factor analysis (FA) and PCA have provided useful results in investigations of a variety of environmental phenomena (Stanimirova et al. 1999; Simeonov et al. 2000; 2001; Sundqvist et al. 2010).
Nevertheless, PCA and FA present serious drawbacks because of the possibility that negative amounts will be present in almost all factors, and the rotational indeterminacy of the solution means that multiple valid solutions can be found (Mooibroek et al. 2007). The PCA method is incapable of handling missing and below-detection-limit data often observed in environmental measurements.
Paatero and Tapper (1994) developed positive matrix factorization (PMF), which overcomes the problems of prior methods by including strict non-negativity constraints to obtain more physically explainable factors (Paatero et al. 2002; Huang and Conte 2009; Comero et al. 2011). Moreover, the PMF technique includes error estimates of observed values, allowing the user to handle extreme values by using the estimates as weighting parameter. Accordingly, this method enables non-representative data to be used in the analysis by reducing their importance through appropriate weighting of the uncertainties and skewed distributions rather than normalization (Sofowote et al. 2008).
The Tehuantepec Gulf is located on the Pacific Coast of the Isthmus of Tehuantepec. The economic development in the region has been mostly based on oil industry activities and has included major transformations such as the construction of the Trans-isthmus highway, the industrial harbor of Salina Cruz, and the oil refinery.
Salina Cruz is a medium-sized town situated on the Pacific Coast of the Isthmus of Tehuantepec (16.2° N, 95.2° W). The city, with an official population of 76,000 inhabitants, is the southern terminal of the railroad that crosses the Isthmus of Tehuantepec and also serves as a southern port primarily for the products of one of Mexico's largest oil refineries with an installed capacity of 330,000 barrels of crude oil per day (Secretarìa de Energía 2009).
The Salina Cruz region is a very productive and dynamic system that supports a great variety of commercial fisheries (including shrimp and tuna) but also has experienced fast growth rates in urbanization and industrialization since the 1950s (Ruiz-Fernández et al. 2004, 2009).
We have studied the environmental impact caused by the economic development in the inner shelf off the Salina Cruz harbor and coastal areas at the Tehuantepec Gulf. Trace metals (Cr, Cu, Fe, Pb, Ni, V, and Zn) and total aromatic hydrocarbons in surface sediment samples collected from 1982 to 2002 indicated that Cu, Ni, and Pb showed concentrations in the range of possible toxicity effects in 20 to 30 % of cases (González-Macías et al. 2006, 2009).
Later in February 2008, sediment samples collected at the Bay showed the presence of Cd, Hg, and As, the first two at concentrations above the threshold effect level. The concentrations of Pb, Ni, and Cu increased almost two times the previous detected levels, which is not the case for V (Schifter et al. 2011).
In the present study, sediment samples from three continental water bodies of the Tehuantepec Basin (TB) were collected, and PMF analysis was performed to identify possible sources of metals to the Bay. Water systems are related to different land uses in the TB and thus represent different apportionment sources to the Tehuantepec Gulf that could help explain the cause of environmental problems detected in previous studies.
Methods
Study area
The studied area is located at the North side of the Tehuantepec Gulf in the Mexican Pacific Ocean (16º06′–16º11′ N and 95º15′–95º07′ W). In the area, high-energy oceanographic conditions are observed, which generate dispersal of the different inputs.
The TB is an ecological microsystem with high evaporation and dilution rates from fluvial runoff and highly productive lagoon systems. Continental mountain chains present deep fluvial canyons exposed to erosive processes that play an important role in the formation of coastal plains, lagoons, and littoral sedimentary environments.
Drainage basins are dendritic, and 40 % of the soils are subjected to erosion throughout the drainage basins. The main surface current pattern runs east to west and is characterized by two seasonal climatic conditions, rainy (May to September) and dry windy (October to April) (Chelton et al. 2000).
The TB presents hydrogeographical and orographic conditions that divide the region into three systems: Zanjon–Ventosa, Tehuantepec River, and estuary–lagoon systems, as displayed in Fig. 1. The systems are separated by terrain elevations or breakthroughs (represented by dotted lines in Fig. 1) that form microbasins, each one influenced by sources derived from a variety of socioeconomic activities.
The Gulf of Tehuantepec receives continental runoff from the Ventosa estuary–Zanjon stream system. The Zanjon stream runs perpendicular to the coast, along the refinery facilities.
The Tehuantepec River is 240 km long with a basin area of 10,090 km2 and mean annual surface flux of 950 × 106 m3. The Tehuantepec coast covers most of the coastal plain of the Gulf and discharges an annual volume of 3,624 × 106 m3 of natural waters (Ruiz-Fernández et al. 2004, 2009).
The Tehuantepec River discharges to the bay approximately 1,400 million m3 year-1 of water, while the La Ventosa estuarine system only discharges water during the rainy seasons; during the rest of the year, the exchange with the Bay is not constant.
The estuary–lagoon system consists of small estuaries and streams: Igú, San Pedro and Los Perros streams, and Xadani and Piedra estuaries that drain to the Laguna Superior and eventually to the Gulf of Tehuantepec through the Laguna Inferior. The Laguna Superior is an irregular basin 20.5 km wide, 33 km long, and about 350 km2 in area with an annual runoff of 1,119 × 106 m3.
Sampling and sediment analysis
A total of 283 samples of the top 5 to 10 cm of sediment were collected from three continental water bodies of the TB from December 1995 to August 2002 using a Smith–McIntyre grab sampler. Table 1 shows the polygonal geographical limits of the sampling locations.
Table 2 shows the number of samples from each water body of the TB systems, as well as the variables considered, their detection limits, and the number of samples below the detection limits.
Samples were stored at 4 °C prior to processing in the laboratory, and all sampling equipment and storage containers were acid-washed before being used. Sediment grain size was estimated by a combination of wet sieving (2 mm–63 μm) and pipette analysis (<63 μm). The total extractable organic matter (EOM) was quantified by infrared spectrometry in a Perkin Elmer UNICAM Model SP-2000, based on the United States Environmental Protection Agency (USEPA) Method 418.1 (United States Environmental Protection Agency USEPA 1978, 1996).
Calibration was performed every ten set of samples using a multi-elemental standard solution (SPEX CertiPrep. Inc. and Perkin-Elmer Corporation PE-Pure standards both traceable to the National Institute of Standards and Technology) via the standard curve approach. From spiked samples, the absolute recoveries of the reference standard for metals ranged from 85 to 90 %, while they ranged from 70 to 110 % for EOM.
Geoaccumulation index (I geo) and EF
Differentiating the metals originating from human activity and those from natural weathering is an essential part of geochemical studies. One such technique largely applied is “normalization,” where metal concentrations were normalized to a textural or compositional characteristic of sediments.
Since Al, Fe, and grain size all tend to covary, the use of a single normalizer can often represent several underlying geochemical relationships. First, Pearson coefficients were used to check relationships between a normalizer and contaminants of concern, and in addition to normalizing metals, we use the geometric mean of fine-grained fractions and EOM for each water body, considering them as conservative.
An EF was calculated for each metal, using the following equation, according to Taylor (1964):
EFs close to unity indicate a crust source; those < 1.0 suggest possible mobilization or depletion of metals (Szefer et al. 1996), and those > 1.0 indicate that the element is of anthropogenic origin. EFs > 10 are considered to be from non-crusted sources (Nolting et al. 1999).
I geo for metals was calculated using Muller’s (1969) expression:
where (M)i is the measured concentration of the element in the environment and (M)r is the average concentration of the metals in crustal earth.
Based on Muller (1969), the contamination level may be classified according to its intensity using a scale ranging from 1 (none) to 6 (very strong). The metal abundance reference values used were from Taylor (1964) and correspond to the average composition of continental crust as follows: V 135, Cr 100, Ni 75, Cu 55, Zn 70, As 1.8, Cd 0.2, and Pb 12.5 (μg g-1).
For EOM and fine-grained materials, we used the following geometric mean values from each water body: estuaries (EOM 177.95 μg g-1, fines 27.01 %), lagoons (EOM 117.31 μg g-1, fines 18.89 %), Tehuantepec (EOM 369.52 μg g-1, fines 3.21 %), Ventosa (EOM 421.57 μg g-1, fines 27.46 %), and Zanjon (EOM 862.74 μg g-1, fines 24.44 %).
Positive matrix factorization
The mathematical foundation of the PMF has been extensively described (Paatero and Tapper 1994; Paatero et al. 2002; 2005). PMF is a factor analysis method that employs individual weighting of matrix elements. PMF solves the equation:
In this equation, X is the matrix of measured values, G and F are the factor matrices to be determined, and E is the matrix of residuals, the unexplained part of X. In PMF, the solution is a weighted least squares fit, where the known standard deviations for each value of X are used to determine the weights of the residuals in matrix E.
The objective of PMF is to minimize the sum of the weighted residuals. PMF uses information from all samples by weighting the squares of the residuals with the reciprocals of the squares of the standard deviations of the data values.
In environmental pollution problems, one row of X would consist of the concentrations of all chemical species in one sample, and one column of X would be the concentration of one species for each of the samples. One row of the computed F matrix would be the source profile for one source, and the corresponding column of G would be the amount of this source in each individual sample.
The required input matrices for PMF were X, the measured values, and Xstd-dev, the standard deviations (uncertainties) of the measured values. PMF requires that all values and uncertainties be positive values; therefore, missing data and zero values must be omitted or replaced with appropriate substitute values.
Data matrix pretreatment
Missing concentrations were replaced by the geometric mean of the concentrations, and their accompanying uncertainties were set at four times of this geometric mean concentration (Kim and Hopke 2005). In cases were a whole period of data was not available, the non-parametric SiZer method (Chaudhuri and Marron 1999) was applied to assess the statistical significance of the reconstructed metal variation along time.
Smoothing methods and SiZer are useful statistical tools for discovering statistically significant structure in data. Based on scale space ideas originally developed in the computer vision literature, SiZer (significant ZERO crossing of the derivatives) is a graphical device used to assess which observed features are “really there” and which are just spurious sampling artifacts (Park et al. 2004).
Most of the variables were heavily skewed according to the Kolmogorov–Smirnov test; therefore, the data matrices were transformed using ln(x + 1) to improve normality and reduce the effect of differences in measurement units.
Central tendency analysis of the original variables was performed on a regional basis and for each water body in the TB. Extreme values were kept to appraise the quality of sediments. The literature survey revealed that several approaches have been used to handle values below the method detection limit (MDL).
Based on the studies of Kim and Hopke (2005) and Polissar et al. (1998; 2001), the measured concentrations below the MDL values were replaced by half of the MDL values, and their uncertainties were set at 5/6 of the MDL values. Statistical treatment of the data was accomplished using standard statistical software (Statistica 1998).
Substituted values had an impact on the PMF results. In our databases, the percentage of substituted values varied depending on the chemical species; the largest substitutions are for Cd (54 %) and V (61 %). Following this example, cadmium was classified as “weak,” and thus, the PMF model assigned three times the provided uncertainty and lowered its importance into further calculations.
Another influence of substituted values on PMF was noted in the Q parameter: smaller Q-values (49–51 % smaller) than the theoretical Q were obtained; nevertheless, authors such as Chueinta et al. (2000), Polissar et al. (2001), and Song et al. (2001) report a reasonable deviation of the Q-value from the Q-theoretical for matrices containing missing and/or below-detection-limit data.
Usually, the obtained Q-value can be smaller than the theoretical, and this is an acceptable result. For instance, Polissar et al. (2001) obtained a Q of 5,367 for an 11-factor solution in Vermont, whereas the theoretical Q was 13,351. Initial runs of PMF were conducted using two to ten factors in order to determine the optimum number of factors, which was between four and five according to the Q-value goodness-of-fit criterion. Both Q true and Q robust were 50 % smaller than the Q theoretical.
Chemical species were classified as strong, weak, or very weak according to the signal-to-noise ratio (S/N) range value proposed by Reff et al. (2007). Once the optimal number of factors was found, results were tested for residual analysis and bootstrap model results (Chung et al. 1996) to ensure that fitting parameters were met.
Results and discussion
Physical and chemical characterization of the sediment
Table 3 shows grain size, extractable organic matter, and metal content values of the input dataset. Higher mean values primarily correspond to the Zanjon fine fractions. The As and Cd values are higher in Ventosa, while those of Cr are higher in estuaries. In general, the river had the lowest concentrations, which suggests that this behavior probably is related to its physical dynamics.
Geoaccumulation index and enrichment factors of the sediment
The upper graph in Fig. 2 summarizes the calculated I geo and EF using EOM and a grain fraction <63 μm as reference elements for the total period of study. The upper graph shows the I geo for all metals and organic matter analyzed in the sediments. An I geo higher than 6 indicates that the metal concentration is 100 times greater than would be expected in the crust.
The widest concentration range was observed for Cd (none to very strong pollution). Indexes for arsenic ranged from none to strongly polluted, while Pb and EOM showed concentration levels ranging from none to moderately to strong pollution. The geoaccumulation indexes for the rest of the metals indicated none.
EFs were calculated by applying two different variables as crustal references to distinguish inorganic (fine fractions) from organic (EOM) sediment enrichments, which are included in the middle and bottom graphs of Fig. 2, respectively.
Metals and organic matter were depleted relative to crustal abundance in a range of 45–55 %, while there was inorganic enrichment from lithogenous/anthropogenic sources of around 40 %. Only 5 % values of Ni, Pb, and EOM had EF above 10, which indicates a non-crustal source, suggesting that additional input cannot be explained by local runoff or erosion processes.
The bottom of Fig. 2 indicates that organic enrichment is dominated by lithogenous/anthropogenic sources, with less intensive depletion with respect to crustal abundance than inorganic enrichment. Cd, Cr, and Pb present an organic enrichment that cannot be related to local processes such as municipal, domestic, or industrial discharges.
Figure 3 presents the I geo index for each water system for metals with a high accumulation range. The Ventosa–Zanjon system consistently had the widest pollution range for all metals, while the Tehuantepec River had the lowest. Cd and arsenic appeared to occur throughout the region.
Enrichment factors are shown in Fig. 4. The Tehuantepec River had higher depletion processes influenced by both organic and inorganic factors. Lead had the highest enrichment factors and was primarily derived by inorganic sources not related to crustal accumulation processes.
The enrichment of Pb in lagoons was related to the presence of organic matter. Lagoons showed higher enrichment factors from organic origin, and EOM and fine particles were related in lagoons. The dominant metal accumulation process was from lithogenous/anthropogenic sources.
Source apportionment estimations
Table 4 summarizes the PMF results and shows the percentage of each chemical species (EOM, As, Cd, Cu, Cr, Ni, Pb, V, Zn, and fine-grained fraction) in factors determined with the model. Correlation coefficients between the observed and estimated concentrations are also shown in Table 4. It should be noted that low correlation values were obtained for weak species (i.e., those showing low S/N values).
Zanjon–Ventosa system
The Zanjon–Ventosa system receives municipal discharges from Salina Cruz city as well as those derived from the oil processing activities. The first factor determined by the PMF model contains an abundant proportion of fine grains (25.84 %), indicating carry and deposition processes of suspended solids enhanced by a long residence time.
The second factor showed high proportions of Pb (29.08 %), As (10.31 %), and Cu (10.04 %) without V contribution. Lead is usually found in ore with zinc, silver, and copper and is extracted together with these metals. The major sources of Pb in natural waters include manufacturing processes and atmospheric deposition. Other sources include domestic wastewaters, sewage, and sewage sludge (Denton, et al. 1997).
The main anthropogenic activities that release As into the environment are mining, fossil fuel processing and combustion, wood preservation, pesticide production and application, and disposal and incineration of municipal and industrial wastes (Huang and Conte 2009), all of which occur close to the study area (Schifter, et al. 2011).
Cu is a moderately abundant heavy metal with a mean concentration in the lithosphere of about 39 μg g-1. Cu is an essential trace element for the growth of most aquatic organisms; however, it becomes toxic to aquatic organisms at levels as low as 10 μg g-1 (Hornberger et al. 2000). Cu is input into natural aquatic systems from various sources including mining, smelting, domestic and industrial wastewater, steam electrical production, incinerator emissions, and dumping of sewage sludge. Therefore, the factor is considered to reflect domestic wastewaters combined with incineration and industrial wastes.
The third factor contains elevated proportions of Zn (20.04 %), As (10.06 %), and V (25.14 %). Zn is a very common environmental contaminant that usually outranks all other metals considered in this study in terms of abundance. Major sources of Zn to the aquatic environment include the discharge of domestic wastewater, coal-burning power plants, and manufacturing processes involving metals, atmospheric fallout, and fertilizer and cement production (Denton et al. 2001).
More than 65 × 106 kg of V is added to the environment through natural sources (earth crust erosion and volcanic emissions) and approximately 200 × 106 kg by anthropogenic activities (Galloway et al. 1980; Hernandez and Rodriguez 2012). V can be found as metallic and organometallic complexes in crude oils and materials of fossil origin. Therefore, the factor is considered to reflect domestic and industrial wastewaters, and oil spills.
The fourth factor contained Cr (19.84 %), Ni (17.80 %), Zn (16.10 %), and Cu (14.04 %) as representative chemical species. Major coastal marine contributors of chromium are dominated by input from rivers, urban runoff, domestic and industrial wastewaters, and sewage sludge (Denton, et al. 1997), suggesting industrial waste discharges, atmospheric deposition, and crude oil processes associated with the refinery activities (Salazar-Coria, et al. 2010). Concisely, the factor must be associated within industrial processes related to oil transformations.
For the Zanjon–Ventosa system, Fig. 5 shows the normalized source contribution along the period of study obtained by PMF. It can be observed that the calculated source contribution remained relatively constant.
Tehuantepec River system
The Tehuantepec River collects a variety of wastes derived from cattle raising, agriculture by irrigation, mining operations (Fe Mn, Pb, Zn, and Au), domestic discharges, and open dumping of municipal solid wastes from Santo Domingo Town (42,000 inhabitants) that eventually drains directly into the Gulf.
The first factor showed a high proportion of EOM (20.26 %) combined with Cu (17.53 %) and Cr (17.22 %). According to López-Garrido and Bautista Galicia (2008), the river receives 131,264 × 106 m3 of residual waters, 90 % of them from agriculture. Therefore, the first factor was likely related to organic accumulation associated with discharges of agricultural wastewater, which is a common practice in the area.
The second factor was primarily composed of EOM (21.83 %), Zn (18.57 %), Cr (16.25 %), and Cd (11.80 %); these suggest urban and mining waste stream practices. Cadmium is a common impurity in zinc, lead, and copper ores, and it is most often isolated during the production of zinc.
The major sources of cadmium include metallurgical industries, municipal effluents, sewage sludge and mine wastes, fossil fuels, and some phosphorus containing fertilizers. In sediments, cadmium does not appear to be absorbed in colloidal materials, but organic matter appears to be the main sorption material for the metal (Finkelman 2005).
The third factor was dominated by organic matter (32.70 %), followed by Zn (16.87 %) and arsenic (12.61 %). These findings indicate that this factor was related to domestic discharges and cattle/farming activities, including the use of organic arsenical pesticides that are rapidly converted to inorganic forms of As in soils and readily mobilized (Whitmore et al. 2008).
The fourth factor contained mainly Ni (26.07 %) and Pb (15.92 %), which is related to mining activities. The main source of discharge of Ni to natural waters is municipal wastewater, followed by smelting and refining of non-ferrous metals (Pekey 2006; González-Fernández et al. 2011). Also, mine drainage effluents are known to be major contributors due to high concentrations of nickel found in the discharges (Finkelman 2005).
Figure 6 shows the distribution of the sources estimated by the PMF model from 1998 to 2002 for the Tehuantepec River system. The contribution of solid waste tended to increase during this period, while agricultural wastewater showed lower values in 2001–2002. These findings are probably the result of land use shifting from agricultural to urban. Moreover, the maximum concentrations of Cr, Pb, and Ni were observed in 2001–2002 together with the minimum values of EOM, which supports the importance of urban waste discharge along the river.
Estuary and lagoon system
The system is surrounded by plantations (corn, sugar cane, and sorghum) and mining areas (Fe, Mn, Pb, Zn, Au, and Ag). Additionally, domestic and commercial fishing activities including boat maintenance take place in the Superior Lagoon. Urban discharges of Juchitán (75,000 inhabitants) are received through Igú and San Pedro streams.
The first factor showed a high importance of the fine-grained fraction (20.38 %), Zn (15.61 %), Cr (12.85 %), and Cu (11.87 %). Nowadays, Cu is the main component of anti-fouling paints used in the area, with boat traffic considered as a major anthropogenic source of Cu in the coastal aquatic environment (Karlsson et al. 2010). The factor is related to erosion, urban discharges, and boat maintenance.
The second factor was composed of an important proportion of EOM (28.56 %) combined with Pb (18.74 %). This factor indicates organic inputs and mining activities. It is important to note that the estuary–lagoon system is a mining zone; therefore, the presence of Pb might be associated with this activity. Therefore, the factor is related to mining and organic accumulation.
The third factor was associated with a high proportion of V and EOM. The high value of V is associated with oil spills that occur in the region. The pentavalent form of V is most soluble and is the primary agent of transport in surface water (Moore 1991). V is also soluble from stabilized oil ash waste in seawater (Breslin and Duedall 1988).
The fourth factor was rich in Cr (27.86 %) and Ni (14.42 %). Therefore, the factor is considered to reflect municipal and industrial wastewaters, and mining activities.
Figure 7 depicts the calculated source contribution for the estuary–lagoon system from December 1995 to August 2002. Oil spills were the most important source for the 1995–1999 samples, but were less important in 2002. Lately, municipal wastes and mining have dominated the contribution.
Conclusion
Regionally, metals and organic matter in the sediments are depleted relative to their crustal abundance in a range of 45–55 %, while there is an inorganic enrichment from lithogenous/anthropogenic sources of around 40 %. Only EOM, Pb, As, and Cd can be correlated with non-crustal sources, suggesting that additional input cannot be explained by local runoff or erosion processes.
Accordingly, species distributions obtained with PMF analysis explain that EOM accounted for nearly 40 % of the organic inputs from runoff in estuaries and lagoons, while the most important sources in Tehuantepec River were domestic discharges and animal waste from farms and livestock/poultry and dairy production operations.
In the Zanjon and Ventosa system, EOM is not related to a particular source. The presence of Pb, as it was identified in the estuaries and lagoons, and Tehuantepec River systems, was strongly related to mining activities as it was identified in the estuaries and lagoons, and Tehuantepec River systems.
In the Zanjon and Ventosa system, Pb was related to oil refinery activities. Arsenic was primarily derived from the regional use of agrochemicals and pesticides. Cd in the Tehuantepec River originated from urban and mining wastes.
Analysis of sediment data from the Tehuantepec Basin made it possible to extract latent factors responsible for the data structure. The Tehuantepec River is directly impacted by municipal discharges, location of temporary crops, and domestic livestock. The Zanjon stream and Ventosa estuary are the two water bodies most impacted by pollutants because they receive effluent from the Tehuantepec River and the refinery is located in their downstream region.
The system of lagoons and estuaries is situated in a higher elevation area, which is less influenced by anthropogenic activities. Nevertheless, these systems receive effluent from the Los Perros River, which has an intense flow rate; therefore, it is subject to intensive boat traffic and fishing.
Overall, the results of PMF indicate specific sources of metal contamination in the water bodies of the TB. Impacts by mining, petroleum refining, and use of agrochemicals distinguish each water body; however, it is important to specifically characterize each source to correlate and accurately quantify the inlets to the aquatic ecosystems in the TB.
References
Birch, G. F., Evenden, D., & Teutsch, M. E. (1996). Dominance of point source in heavy metal distributions in sediments of a major Sydney estuary (Australia). Environmental Geology, 28, 169–174.
Breslin, V. T., & Duedall, I. W. (1988). Vanadium release from stabilized oil ash waste in seawater. Environmental Science and Technology, 22, 1166–1170.
Çevik, F., Lugal-Göksu, M. Z., Deric, O. B., & Fındık, Ö. (2011). An assessment of metal pollution in surface sediments of Seyhan Dam by using enrichment factor, geoaccumulation index and statistical analyses. Environmental Monitoring and Assessment, 152, 309–317.
Chaudhuri, P., & Marron, J. S. (1999). SiZer for exploration of structure in curves. Journal of the American Statistical Association, 94, 807–823.
Chelton, D. F., Freilich, M. H., & Esbensen, S. K. (2000). Satellite observations of the wind jets off the Pacific Coast of Central America. Part II: regional relationships and dynamical considerations. Monthly Weather Review, 128, 2019–2043.
Chow, J. C., & Watson, J. G. (2002). Review of PM2.5 and PM10 apportionment for fossil fuel combustion and other sources by the chemical mass balance receptor model. Energy and Fuels, 16, 222–260.
Chueinta, W., & Hopke, P. K., & Paatero, P. (2000). Investigation of sources of atmospheric aerosol at urban and suburban residential areas in Thailand by positive matrix factorization. Atmospheric Environment, 34, 3319–3329.
Chung, H. Y., Lee, K. W., & Koo, J. Y. (1996). A note on bootstrap model selection criterion. Statistic Probability Letters, 26, 35–41.
Comero, A., Locoro, G., Free, G., Vaccaro, S., De Capitani, L., & Gawlik, B. M. (2011). Characterization of Alpine lake sediments using multivariate statistical techniques. Chemometrics and Intelligent Laboratory Systems, 107, 24–30.
Denton, G. R. W., Wood, H. R., Concepcion, L. P., Siegrist, H. G., Eflin, V. S., Narcis, D. K., & Pangelinan, G. T. (1997). Analysis of in-place contaminants in marine sediments from four harbor locations on Guam: a pilot study. Mangilao: Water and Environmental Research Institute of the Western Pacific, Technical Report No. 87, University of Guam.
Denton, G. R. W., Bearden, B. G., Concepcion, L. P., Siegrist, H. G., Vann, D. T., & Wood, H. R. (2001). Contaminant assessment of surface sediments from Tanapag Lagoon, Saipan. Mangilao: Water and Environmental Research Institute of the Western Pacific, Technical Report No. 93, University of Guam.
Finkelman, R. B. (2005). Sources and health effects of metals and trace elements in our environment: an overview. In T. A. Moore, A. Black, J. A. Centeno, J. S. Harding, & D. A. Trumm (Eds.), Metal contaminants in New Zealand (pp. 25–46). Christchurch: Resolution Press.
Galloway, J. N., Eisenreich, S. J., & Scott, B. C. (1980). Toxic substances in atmospheric deposition: a review and assessment. National Atmospheric Deposition Program, Report, NC-141.
Geffard, O., Geffard, A., His, E., & Budzinski, H. (2003). Assessment of the bioavailability and toxicity of sediment associated polycyclic aromatic hydrocarbons and heavy metals applied to Crassostrea gigas embryos and larvae. Marine Pollution Bulletin, 46, 481–490.
Ghrefat, H. A., Rukah, Y. A., & Rosen, M. A. (2011). Application of geoaccumulation index and enrichment factor for assessing metal contamination in the sediments of Kafrain Dam, Jordan. Environmental Monitoring and Assessment, 178, 95–109.
González-Fernández, D., Garrido-Pérez, M. C., Nebot-Sanz, E., & Sales-Márquez, D. (2011). Source and fate of heavy metals in marine sediments from a semi-enclosed deep embayment subjected to severe anthropogenic activities. Water Air Soil Pollution, 221, 191–202.
González-Macías, C., Schifter, I., Lluch-Cota, D. B., Méndez-Rodríguez, L., & Hernández-Vázquez, S. (2006). Distribution, enrichment and accumulation of heavy metals in coastal sediments of Salina Cruz Bay, México. Environmental Monitoring and Assessment, 118, 211–230.
González-Macías, C., Schifter, I., Lluch-Cota, D. B. M., Rodríguez, L., & Hernández-Vázquez, S. (2009). Assessment of benthic changes during 20 years of monitoring of the Mexican Salina Cruz Bay. Environmental Monitoring and Assessment, 149, 113–132.
Henry, R. C., Lewis, C. W., Hopke, P. K., & Williamson, H. J. (1984). Review of receptor model fundamentals. Atmospheric Environment A, 18, 1507–1515.
Henry, R. C. (1997). History and fundamentals of multivariate air quality receptor models. Chemometrics and Intelligent Laboratory Systems, 37, 37–42.
Hernandez, H., & Rodriguez, R. (2012). Geochemical evidence for the origin of vanadium in an urban environment. Environmental Monitoring and Assessment, 184, 5327–5342.
Hornberger, M. I., Luoma, S. N., Cain, D. J., Parchaso, F., Brown, C. L., Bouse, R. M., Wellise, C., & Thompson, J. K. (2000). Linkage of bioaccumulation and biological effects to changes in pollutant loads in South San Francisco Bay. Environmental Science and Technology, 12, 2401–2409.
Huang, S., & Conte, M. H. (2009). Source/process apportionment of major and trace elements in sinking particles in the Sargasso Sea. Geochimica et Cosmochimica Acta, 73, 65–90.
Imamoglu, I., & Christensen, E. R. (2002). PCB sources, transformations, and contributions in recent Fox River, Wisconsin, sediments determined from receptor modeling. Water Research, 36, 3449–3462.
Karlsson, J., Ytreberg, E., & Eklund, B. (2010). Toxicity of anti-fouling paints for use on ships and leisure boats to non-target organisms representing three trophic levels. Environmental Pollution, 158, 681–687.
Kim, E., & Hopke, P. K. (2005). Identification of fine particle sources in mid-Atlantic US area. Water Air and Soil Pollution, 168, 391–421.
Kosmehl, T., Hallare, A. V., Braunbeck, T., & Hollert, H. (2008). DNA damage induced by genotoxicants in zebrafish (Danio rerio) embryos after contact exposure to freeze-dried sediment and sediment extracts from Laguna Lake (the Philippines) as measured by the comet assay. Mutation Research, 650, 1–14.
López-Garrido, P.A., Bautista Galicia, N. (2008). Diagnóstico de la contaminación del agua en el estado de Oaxaca. Pan American Health Organization, Virtual Library of Sustainable Development and Environmental Health. http://www.bvsde.paho.org/bvsaidis/saneab/mexicona/R-0157.pdf.
Mai, B., Qi, S., Zeng, E. Y., Yang, Q., Zhang, G., Fu, J., Sheng, G., & Wang, Z. (2003). Distribution of polycyclic aromatic hydrocarbons in the coastal region off Macao, China: assessment of input sources and transport pathways using compositional analysis. Environmental Science and Technology, 37, 4855–486.
Mooibroek, D., Hoogerbrugge, R., & Bloemen, H.J.Th. (2007). Implementation of source apportionment using positive matrix factorization. Application of the Palookaville exercise. National Institute for Public Health and the Environment, RIVM Report 863001006/2007. The Netherlands.
Moore, J. W. (1991). Inorganic contaminants of surface water: Research and monitoring priorities. New York: Springer.
Muñoz-Barbosa, A., Gutiérrez-Galindo, E. A., Daesslé, L. W., Orozco-Borbón, M. V., & Segovia-Zavala, J. A. (2012). Relationship between metal enrichments and a biological adverse effects index in sediments from Todos Santos Bay, northwest coast of Baja California, México. Marine Pollution Bulletin, 64, 405–409.
Muller, G. (1969). Index of geo-accumulation in sediments of the Rhine River. Geojournal, 2, 108–118.
Nolting, R. F., Ramkema, A., & Everaats, J. M. (1999). The geochemistry of Cu, Cd, Zn, Ni and Pb in sediment cores from the continental slope of the Banc d'Arguin (Mauritania). Continental Shelf Research, 19, 665–691.
Paatero, P., & Tapper, U. (1994). Positive matrix factorization: a non-negative factor model with optimal utilization of error estimates of data values. Environmetrics, 5, 111–126.
Paatero, P., Hopkeb, P. K., Song, X., & Ramadan, Z. (2002). Understanding and controlling rotations in factor analytic models. Chemometrics and Intelligent Laboratory Systems, 60, 253–264.
Paatero, P., Hopkeb, P. K., Begumb, B. A., & Biswasc, S. K. (2005). A graphical diagnostic method for assessing the rotation in factor analytical models of atmospheric pollution. Atmospheric Environment, 39, 193–201.
Park, C., Marron, J. S., & Rondonotti, V. (2004). Dependent SiZer: goodness of fit tests for time series models. Journal of Applied Statistics, 31, 999–1017.
Pekey, H. (2006). The distribution and sources of heavy metals in Izmit Bay surface sediments affected by a polluted stream. Marine Pollution Bulletin, 52, 1197–1208.
Peré-Trepat, E., Ginebreda, A., & Tauler, R. (2007). Comparison of different multiway methods for the analysis of geographical metal distributions in fish, sediments, and river waters in Catalonia. Chemometrics and Intelligent Laboratory Systems, 88, 69–83.
Polissar, A. V., Hopke, P. K., Paatero, P., Malm, W. C., & Sisler, J. F. (1998). Atmospheric aerosol over Alaska 2. Elemental composition and sources, Journal of Geophysical Research, 103, 19045–19057.
Polissar, A. V., Hopke, P. K., & Poirot, R. L. (2001). Atmospheric aerosol over Vermont: chemical composition and sources. Environmental Science and Technology, 35, 4604–4621.
Reff, A., Eberly, S. I., & Bhave, P. V. (2007). Receptor modeling of ambient particulate matter data using positive matrix factorization: review of existing methods. Journal of the Air and Waste Management Association, 57, 146–154.
Ruiz-Fernández, A. C., Páez-Osuna, F., Machain-Castillo, M. L., & Arellano-Torres, E. (2004). 210Pb geochronology and trace metal fluxes (Cd, Cu and Pb) in the Gulf of Tehuantepec, South Pacific of Mexico. Journal of Environmental Radioactivity, 76, 161–175.
Ruiz-Fernández, A. C., Hillaire-Marcel, C., de Vernal, A., Machain-Castillo, M. L., Vásquez, L., Ghaleb, B., Aspiazu-Fabián, J. A., & Páez-Osuna, F. (2009). Changes of coastal sedimentation in the Gulf of Tehuantepec, South Pacific Mexico, over the last 100 years from short-lived radionuclide measurements. Estuarine, Coastal and Shelf Science, 82, 525–536.
Sakurai, T. (2003). Dioxins in aquatic sediment and soil in the Kanto region of Japan: major sources and their contributions. Environmental Science and Technology, 37, 3133–3140.
Salau, J. S. I., Tauler, R., Bayona, J. M., & Tolosa, I. (1997). Input characterization of sedimentary organic contaminants and molecular markers in the northwestern Mediterranean Sea by exploratory data analysis. Environmental Science and Technology, 31, 3482–3490.
Salazar-Coria, L., Schifter, I., & González-Macias, C. (2010). Weighing the evidence of ecological risk from PAHs contamination in the estuarine environment of Salina Cruz, Bay, Mexico. Environmental Monitoring and Assessment, 162, 387–406.
Schifter, I., González-Macias, C., Salazar-Coria, L., & González-Lozano, C. (2011). Pollution in estuarine and bay sediments at a refinery complex located on the Mexican Pacific Ocean. International Journal of Environmental Studies, 68, 83–106.
Secretarìa de Energìa (2009). Oil products outlook 2007–16. Available at http://www.sener.gob.mx/res/PE_y_DT/pub/Propect%20Petroliferos%20ult.pdf
Simeonov, V., Massart, D. L., Andreev, G., & Tsakovski, S. (2000). Assessment of metal pollution based on multivariate statistical modeling of “hot spot” sediments from the Black Sea. Chemosphere, 41, 1411–1417.
Simeonov, V., Stanimirova, I., & Tsakovski, S. (2001). Multivariate statistical interpretation of coastal sediment monitoring data. Fresenius Journal Analytical Chemistry, 370, 719–722.
Sofowote, U. M., McCarry, B. E., & Marvin, C. H. (2008). Source apportionment of PAH in Hamilton Harbour suspended sediments: comparison of two factor analysis methods. Environmental Science and Technology, 42, 6007–6014.
Song, X. H., Polissar, S. V., & Hopke, P. K. (2001). Sources of fine particle compositions in the northeastern US. Atmospheric Environment, 35, 5277–5286.
Stanimirova, I., Tsakovski, S., & Simenov, V. (1999). Multivariate statistical analysis of coastal sediment data. Fresenius Journal Analytical Chemistry, 365, 489–493.
Statistica. (1998). Statistica for Windows (volume I). General conventions and statistics I (2nd ed.). Tulsa: StatSoft, Inc.
Sundqvist, K. L., Tysklin, T. M., Geladi, P., Hopke, P. K., & Wiberg, K. (2010). PCDD/F source apportionment in the Baltic Sea using positive matrix factorization. Environmental Science and Technology, 44, 1690–1697.
Szefer, P., Glosby, G. P., Szefer, K., Penopkowiak, J., & Kaliszan, R. (1996). Heavy metal pollution in surficial sediments from the Southern Baltic Sea off Poland. Journal Environmental Science Health, 31, 2723–2754.
Taylor, S. R. (1964). Abundance of chemical elements in the continental crust; a new table. Geochimica Cosmochimica Acta, 28, 1273–1285.
United States Environmental Protection Agency (USEPA). (1978). Test method for evaluating total recoverable petroleum hydrocarbon, method 418.1 (spectrophotometric, infrared). Washington, DC: U.S. Government Printing Office.
United States Environmental Protection Agency (USEPA). (1996). Method 8440, total recoverable petroleum hydrocarbon by infrared spectrophotometry (3rd ed.). Washington, DC: Revision-0. U.S. Government Printing Office. SW-846.
Whitmore, T. J., Riedinger-Whitmore, M. A., Smoak, J. M., Kolasa, K. V., Goddard, E. A., & Bindler, R. (2008). Arsenic contamination of lake sediments in Florida: evidence of herbicide mobility from watershed soils. Journal of Paleolimnology, 40, 869–884.
Zhou, F., Guo, H., & Liu, L. (2007). Quantitative identification and source apportionment of anthropogenic heavy metals in marine sediment of Hong Kong. Environmental Geology, 53, 295–305.
Author information
Authors and Affiliations
Corresponding author
Rights and permissions
About this article
Cite this article
González-Macías, C., Sánchez-Reyna, G., Salazar-Coria, L. et al. Application of the positive matrix factorization approach to identify heavy metal sources in sediments. A case study on the Mexican Pacific Coast. Environ Monit Assess 186, 307–324 (2014). https://doi.org/10.1007/s10661-013-3375-0
Received:
Accepted:
Published:
Issue Date:
DOI: https://doi.org/10.1007/s10661-013-3375-0