Abstract
The bighead (Hypothalmichthys nobilis) and silver carp (H. molitrix) have invaded much of the Mississippi River. It is unclear how reproduction in northern impounded pools of the Upper Mississippi River System (UMRS) compares to unimpounded (open) southern reaches. During spring through summer 2005 and 2006 and once in spring 2007, we quantified larval and juvenile production in the pooled and open UMRS. We then simulated population dynamics in pools as a function of apparent reproductive success. Larvae occurred during about 2 weeks each spring. Peak density and apparent spawn duration were greater in the open reach. Larval production peaked when discharge was high plus rising and water temperatures reached 18°C. Most juveniles (>97%) occurred in the open reach. Low flow during drought years in the pools may limit reproductive success. The simulation demonstrated that, by treating dams as barriers to invasion from the lower open river (i.e., a source), climatic conditions may interact with flow in pools to limit populations by creating an isolated sink.
Similar content being viewed by others
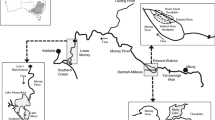
Avoid common mistakes on your manuscript.
Introduction
During the past half century, the number of fish species introduced both intentionally and unintentionally in the United States has increased dramatically (Fuller et al. 1999; Rahel 2000). As with all invasive species (Kolar and Lodge 2001), some pose a threat to aquatic ecosystems by competing with natives for food, spawning areas, and other limited resources, thus reducing density of native fish species (Laird and Page 1996). Two Asian carp species, the bighead carp (Hypothalmichthys nobilis) and silver carp (H. molitrix), have invaded much of the Mississippi River basin in central North America (Chick and Pegg 2001). As bighead and silver carp increase in population size and range, they may outcompete native fishes (Tucker et al. 1996; Laird and Page 1996; Sampson 1999).
Although initially appearing in the southern, unimpounded portion of the Upper Mississippi River System (UMRS), adult bighead and silver carp now occur in northern reaches that have been impounded (i.e., pooled) to allow navigation. This includes the Illinois River, which is connected to the currently uninvaded Lake Michigan (Kolar et al. 2005). Juvenile bighead and silver carp have been found in the lower and middle unpooled reaches (Burr et al. 1996; Tucker et al. 1996) and in the southernmost pools of the UMRS (Koel et al. 2000; Garvey et al. 2003a). However, it is unclear to what extent Asian carp are able to successfully reproduce in navigation pools of the UMRS relative to lower, unimpounded reaches. This disparity may be due, in part, to how characteristic differences between the reaches affect reproduction of the bighead and silver carp.
The ability for bighead and silver carp to reproduce with surviving offspring is fundamental to their establishment and dispersal in the UMRS and may depend on patterns of flow and water level. Silver and bighead carp reproduce similarly. In their native Asian range, spawning occurs during spring through early summer (Jennings 1988; Kolar et al. 2005). Spawning appears to be timed to increased discharge and rising water levels where water velocity increases past 0.7 m/s and flooded backwater areas become available as nursery habitats for drifting larvae (Nikolsky 1963; Verigin et al. 1978; Schrank et al. 2001). Spawning of Asian carp occurs in large rivers at the surface of the channel behind islands or gravel bars (Huet 1970; Jennings 1988; Costa-Pierce 1992; Kolar et al. 2005). Confluences of large tributaries are likely spawning areas (Huet 1970). Larvae may require a contiguous river of at least 100 km for recruitment (Gorbach and Krykhtin 1980).
Patterns of flow and water levels differ markedly between the unimpounded and impounded reaches of the UMRS, with potentially important effects on Asian carp reproduction. The unimpounded reach extending from St. Louis, Missouri to the Gulf of Mexico has been greatly altered but still maintains a spring rise in water level and discharge. The pooled reaches north of St. Louis have complex patterns of flow and water level (Fig. 1). In the middle of each pool, water level is kept constant by managers. With increases in river discharge, water is passed through lower-reach dam gates at a higher rate in each pool than in the upper reach. Thus, within each pool, water level increases at the upstream section, remains relatively constant at the middle, and decreases downstream. During low flow (typically late spring through summer), all gates are closed to keep pools impounded; each pool becomes more lotic upstream and lentic downstream (Garvey et al. 2003a).
Open river and Pools 20–26 of the Upper Mississippi River System (UMRS). Pool numbers are associated with the downstream lock and dam (L&D; black bars). Larval and juvenile Asian carp were sampled in the open river and Pools 26, 24, 22, and 20 during 2005, 2006, and one date in 2007. Larval sites (grey symbols) were located in the upstream and downstream section of the pools about 1.5 km from L&Ds, and near Chester, Illinois in the open river. Juvenile sites were located in the downstream section of UMRS pools and near Chester, Illinois in the open river
During a drought year, adult Asian carp did not appear to reproduce in the lower impounded Illinois River (DeGrandchamp et al. 2007), perhaps due to a lack of proper flow-related conditions. We hypothesized that the relatively unnatural flow regimes and perhaps impeded flow due to dams in the pooled UMRS, particularly during dry springs, might reduce Asian carp reproduction relative to unpooled southern reaches. Thus, we quantified larval densities and survival to the juvenile stage in four UMRS pools and the “open” river of the UMRS (Fig. 1). With this information, we conducted a computer simulation to determine under what conditions might dry spring conditions reduce reproductive success and perhaps population abundance in the pools, if pools became permanently isolated (e.g., via a species-selective barrier) from the unpooled southern UMRS. In other words, at this large spatial scale, we assessed whether the upper reaches of the UMRS might act as an occasional sink in a metapopulation sense (see Schlosser 1998 for example with beaver dams) and whether management might facilitate this ecological mechanism.
Methods
Larval data collection
Larval densities were quantified in 2005 and 2006 at four pools and in the open river. Pooled sites were located in Pool 20 (river kilometer; RK 586-554), Pool 22 (RK 521-484), Pool 24 (RK 484-441), and Pool 26 (RK 388-323) (Fig. 1). The open river was sampled at RK 175-161 (Fig. 1). In 2005, each pool contained two sites: one main channel site about 1.5 km below the upstream lock and dam, and another main channel site about 1.5 km above the downstream dam. The open river contained one main channel site at RK 175. In 2006, all the same main channel sites were sampled, but side channel sites for larval sampling were added. In the pools, side channel sites were located within 2 km of the downstream dam. The side channel site in the open river was located at RK 161 (Fig. 1).
Larvae were sampled weekly from mid-May through July during both years with the exception of 2005 where sampling in the open reach began at the end of June; larvae were sampled bi-weekly through August. In addition, larvae were sampled on May 24th and 25th, 2007 in the open river, Pool 26, and Pool 24 side-channel sites. All larval samples were collected using a 1 × 2 m neuston net with 500 μm mesh. A General Oceanics flow meter (model 2030R) was attached to the neuston net frame to quantify water volume. Samples were gathered by towing upstream at the surface for 4 min twice, with each considered a replicate. Ichthyoplankton samples were rinsed through a 500 μm sieve and preserved immediately in 95% ethanol.
Water temperature (°C) and water velocity (m/s) were measured at each site per sample date. Downstream and upstream water velocities in pools were combined and compared to those in the open river using one-way ANOVA. Pair-wise comparisons were Bonferroni corrected. Velocities in pools were combined to compare between downstream and upstream sections using a t-test. Daily discharge (m3/s) and stage elevation (m) at Locks and Dams 19-26 and at Chester, IL (RK 175) were provided by the St. Louis and Rock Island Districts of the U.S. Army Corps of Engineers. Discharge values at each site from May 15th to August 28th were related to the other sites using Pearsons linear correlation (2005 and 2006 separate).
Larval samples were sub-sampled by at least 25% using a Folsom plankton splitter. Meristic and morphometric characteristics were used to identify larval Asian carps to genus (Soin and Sukhanova 1972; Murty et al. 1986; Tweb et al. 1990; Chapman 2006). Voucher specimens were used to verify identification (D. Snyder, Colorado State University, Larval Fish Laboratory, Fort Collins). Larval Asian carp were counted and converted to density (larvae/m3). Average larval Asian carp density was calculated for each site per sample date. Densities from side and main channel sites in 2006 were included in the same average. Larval densities were compared among sites. When peak larval Asian carp densities occurred between sites during the same week, densities were compared using a t-test. Pair-wise comparisons were Bonferroni corrected. A G-test was used to compare the number of weeks larvae occurred at sites; expected values in the UMR pools were set to the number of weeks larvae occurred in the open river (Sokal and Rohlf 1995).
Lapilli otoliths were removed from 10 to 15 randomly selected larval Asian carp from each site per date for aging; otoliths were removed from all fish in samples with fewer than 10 larvae. Otoliths were mounted in a drop of mineral oil on microscope slides and viewed under a compound microscope at 100× magnification. Two independent readers counted daily rings. Otoliths with ring counts that differed more than 10% between readers were discarded. Ages were averaged between the two readers. Hatch dates were estimated by subtracting the ring count from the date of capture. Birth dates were estimated by subtracting 1 day from the hatch date (Schrank et al. 2001). Frequency distributions of birth dates were compared to spatial and temporal variation of environmental factors such as water temperature, discharge, and water level. A Kolmogorov–Smirnov test compared spawn duration among the reaches.
Juvenile data collection
Each of the pools (RK 325, 444, 489, and 557) and the open river (RK 161) contained a backwater site for juvenile sampling. The open river site was typically disconnected, characterized by muddy benthos with maximum depths ranging from 1 to 2 m. Pool backwater sites were contiguous backwaters or island side channels characterized by muddy benthos and maximum depths ranging from 1 to 2 m.
Juveniles were sampled bi-weekly from mid-July through the beginning of October in 2005 except in the open river where sampling began at the end of September. In 2006, juveniles were sampled bi-weekly in mid-July and weekly from the beginning of August to the beginning of September. Juveniles were sampled from each backwater site using a 3.66-m bag seine with 5-mm mesh. At each site, three, 20-m transects were performed perpendicular to shore. Fish were preserved in 95% ethanol.
Juvenile Asian carp were identified to species using Pflieger (1997). Abundance data from the three hauls at each site were averaged for the number of juvenile Asian carp per seine haul for that site and sample date. Average number of juvenile Asian carp per seine haul was compared among sites.
Lapilli otoliths were removed from 10 to 20 randomly selected juvenile Asian carp from each site per date for aging; otoliths were removed from all fish in samples with fewer than 10 juveniles. Otoliths were mounted in thermoplastic cement on a microscope slide and sanded lightly using 1,000 grit sandpaper. Otoliths were viewed under a compound microscope at 100× magnification, and two independent readers counted daily rings. Otoliths with ring counts that differed more than 10% between readers were discarded. Ages were averaged between the two readers. The methods used to estimate hatch dates and birth dates were used for juveniles. Birth dates of juvenile Asian carp were compared with those of larvae to infer recruitment to the juvenile stage.
Simulation modeling
To assess how variable reproductive success of Asian carp might translate to population persistence in the pools (i.e., could pools be sinks?), we built a simple age-structured model, with recruitment of young to the population depending on the distribution of dry versus wet springs (i.e., expected months of low water in spring). Although the model parameters were largely heuristic, the maximum age (age 6) and mortality rates (30% per year) for each simulated population were derived from empirical data (e.g., Williamson and Garvey 2005; DeGrandchamp 2006). Each model population started with an age structure of 1,000,000 age-0, 100,000 age-1, 10,000 age-2, 1,000 age-3, 100 age-4, 100 age-5, and 10 age-6 cohorts. During each simulated year, if conditions were favorable, recruit “success” added an additional 1,000,000 age-0 individuals to the population. Density declined annually by 30% within each cohort (see Williamson and Garvey 2005) and cohorts only persisted until age 6. Based on our experience with water-level variation in the UMRS, we assumed that the number of “dry” months during the spawning season in pools averaged 3 months with a Poisson distribution. Therefore, dry periods of ≤3 months are common and longer periods are uncommon. We then explored how the duration of dry periods affected the persistence of populations by varying recruitment success as a function of the extent of randomly generated dry periods. We ran 100, 100-year simulations for populations that were drought-tolerant (≥3 months of low discharge caused recruitment failure; i.e., new age-0s = 0), intermediate (≥2 months caused failure), and drought-prone (≥1 months caused failure).
Results
Larvae
Spatial variation
One-hundred ninety and 392 samples were collected in 2005 and 2006. Of these samples, only 25 contained larval Asian carp. Larval Asian carp occurred in the open river and the downstream sections of Pools 26, 24, and 22 (Table 1). No larval Asian carp occurred in upstream sections of any pools or both sites in Pool 20. Larval Asian carp density in the open river and downstream Pool 26 comprised 100% of total larval carp sampled in 2005 and 99.9% in 2006 (Table 1). Larval Asian carp density in the open river was six times greater than in Pool 26 in 2005 and nine times greater in 2006 (Table 1). Peak density in the open river and Pool 26 in 2006 occurred during the same week, and density during the peak was greater in the open river than in Pool 26 (t = 2.50, P = 0.05, df = 6; Table 1). During the single sampling week in 2007, larval Asian carp occurred in all three side channel sites sampled (open river, Pool 26, and Pool 24) (Table 1). Overall, density at sites differed in 2007 (F = 40.23, P = 0.007, df = 2, 3). Larval density between the open river and Pool 24 did not differ (t = 0.768, P = 0.522, df = 2), but both had higher density than Pool 26 (t = 8.37–9.85, P = 0.014–0.010, df = 2).
Temporal variation
Larval Asian carp occurred during 8 of the 24 weeks sampled in 2005 and 2006 (Table 1). Larvae occurred more often in the open river than in the pools (G = 9.503, P < 0.025, df = 3). In 2005, the majority of larval Asian carp density in the open river occurred >2 months later than the peak density in Pool 26. However, a low density of larval Asian carp occurred during our first sampling in the open river (June 30th), and larvae may have occurred before sampling commenced that year (Table 1). In 2006, larval Asian carp occurred the first 2 weeks of June in the open river and Pool 26. Larvae continued to occur in open river samples 1 week later than in Pool 26. In contrast to 2005, no larvae occurred in August 2006 (Table 1).
Spawn duration/cohort trends
Sixteen larval Asian carp were aged in 2005 (with none discarded due to disagreement between readers) and 72 in 2006 (plus four discarded). Spawning duration of surviving larvae in the open river and Pool 26 was similar in 2005 (Fig. 2): larval Asian carp were spawned between 2 and 3 days (Table 2). In 2006, successful spawning duration was longer in the open river than in Pool 26 (Ksa = 2.09, P < 0.001). Larval Asian carp occurring in the open river and Pool 26 were spawned during 19 and 4 days, respectively (Fig. 2, Table 2). The extended spawning duration in the open river was, in part, a result of two closely spawned cohorts. Only one cohort occurred in Pool 26. Spawning producing the first larval cohort in the open river was 9 days longer than the corresponding cohort in Pool 26.
Back-calculated birth dates of larval Asian carp occurring in the open river and Pool 26 (Pool 26) of the Upper Mississippi River System in 2005 and 2006 and water conditions. Samples were collected in the time frame illustrated except for dates prior to the dashed vertical line. Stage elevations in Pool 26 are represented by a solid line for the upstream section of the pool, a dotted line for the middle of the pool, and a segmented line for the downstream section of the pool. The arrow on the upper left hand panel indicates a date when one larval fish caught in the open river in July was estimated to hatch
Abiotic conditions
Water temperatures during both years reached 18°C by the last week of May and stayed below 30°C during the entire season (except for the last week in July and the first week in August) (Fig. 2). Larvae occurred within the range of suitable water temperatures (Table 1). Discharge among all sites was highly correlated (r = 0.91–0.99, P < 0.001) and larval production occurred during periods of high or increasing discharge after water temperatures reached 18°C (Fig. 2).
In 2005, back-calculated birth dates of the larvae occurring in Pool 26 coincided with rising discharge of 504 m3/s between June 4th and 10th after water temperatures reached 18°C on May 24th. When late summer spawning occurred in the open river in 2005, larval Asians again appeared with rising discharge and water level (Fig. 2). Birth dates of larvae occurring in the open river coincided with an increase in discharge of 960 m3/s between August 19th and 26th after water temperatures declined from 32°C on July 25th to 27°C on August 26th (Fig. 2).
In 2006, larval Asian carp in both the open river and Pool 26 occurred in the late spring/early summer. While the overall trend in discharge was decreasing in both the open river and Pool 26, back-calculated birth dates of larvae in the coincided with small increases in discharge (Fig. 2). Separate cohorts in the open river occurred in association with two increases in discharge: one of 690 m3/s between June 1st and 4th, and another of 360 m3/s between June 11th and 14th after water temperatures reached 18°C on May 29th (Fig. 2). A single cohort in Pool 26 occurred after discharge increased by 122 m3/s between May 31st and June 2nd after water temperatures reached 18°C on May 29th (Fig. 2). During 2005 and 2006, no surviving larval Asian carp were spawned below a discharge of 2,972 m3/s or a gage height below 5.98 m (upstream discharge and gage height values used in pools).
Water velocities when larval Asian carp occurred were within the range of purported suitable spawning conditions (Table 2). Water velocities among all sites differed (F = 14.65, P < 0.001, df = 4, 206). Mean velocity in the open river (1.30 m/s; n = 23) was higher than in pools (t = 3.173–8.408, P < 0.05, df = 67–69) and never dropped below 0.60 m/s. In the pools, mean pool velocity generally increased from the downstream to upstream pools: Pool 26 had the lowest mean velocity (0.48 m/s; n = 48) and Pool 20 had the highest (0.83 m/s; n = 48). Mean downstream water velocity in the pools was lower (0.52 m/s) than upstream (0.78 m/s; t = 4.79, P < 0.0001, df = 186).
Juveniles
Forty-two juvenile samples were collected in 2005, and 59 samples were collected in 2006. Of these samples, 24 contained juvenile Asian carp (Table 3). Juvenile Asian carp occurred in the open river and Pool 26. Juveniles in the open river comprised 100% of total Asian carp caught in 2005 and 97% in 2006. Juveniles did not occur in Pools 24, 22, and 20 in either year.
Twenty-eight juvenile Asian carp were aged in 2005 (excluding three discarded due to disagreement between readers) and 36 in 2006 (excluding five discarded). Mean estimated birth dates for larval and juvenile Asian carp overlapped within 2–3 days (Figs. 3, 4). In 2005, juveniles occurring in the open river were spawned as late as August 31st while larvae occurring in the open river were not spawned past August 23rd (Fig. 3). However, larval sampling in the open river ceased on August 26th in 2005, and larval production may have continued after this date. In 2006, ages of 75% of juveniles occurring in the open river overlapped with ages of larvae occurring in the open river (Fig. 4). A small cohort of Asian carp produced between June 28th and July 3rd appeared in juvenile samples but not larval samples. In Pool 26, 25% of juveniles collected were within the age range of larvae collected; the remainder were 1–3 days older (Fig. 4).
Simulations
Simulations demonstrated that the response of Asian carp reproduction to the duration of dry periods during spawning (and hence low velocities, discharge, and water levels) may affect their persistence in the pools. Assuming that adults do not spawn or larvae do not survive during relatively short drought periods (≥1-month, drought prone), then populations may fluctuate widely (Fig. 5) and go extinct between 10 and 60 years (Fig. 6). Extinction is less likely under the intermediate (≥2 months needed to stop reproduction) scenario (Figs. 5, 6). Under the drought tolerant (drought must equal or exceed the average expected duration) scenario, population density fluctuates (Fig. 5) but extinction is unlikely (Fig. 6).
Example of results from one of 100, 100-year simulations per modeling scenario in which Asian carp population density varied as a function of variable recruitment in pools of the Upper Mississippi River System. Successful recruitment depended on the relative duration of a dry spring through summer spawning period (i.e., affecting water level, discharge, and other reproductive cues): drought tolerant = Asian carp would reproduce if dry period was less than 3 months; intermediate = reproduce if dry period <2 months; drought prone = reproduce when dry period <1 month. The average expectation was 3 months of dry conditions, with events distributed among years as a Poisson
Summary of extinction times for 100 simulated Asian carp populations during 100 years depending on variable recruitment in pools of the Upper Mississippi River System (i.e., maximum of 100 observations per panel). Successful recruitment depended on the relative duration of a dry spring through summer spawning period (i.e., affecting water level, discharge, and other reproductive cues): drought tolerant = Asian carp would reproduce if dry period was less than 3 months; intermediate = reproduce if dry period <2 months; drought prone = reproduce when dry period <1 month. The average expectation was 3 months of dry conditions, with events distributed among years as a Poisson
Discussion
Successful reproduction and survival of non-indigenous organisms is a key component to their establishment and subsequent dispersal within an ecosystem (Colautti and MacIssac 2004). The success of invaders needs to be viewed in a spatially explicit context to determine how rapidly they may spread through ecosystems (Lodge et al. 1998, Garvey et al. 2003b). In other words, if areas of high survival and reproductive success are contiguous, then invaders may spread rapidly. In contrast, sinks can act as barriers for dispersal.
Only a handful of studies have focused on reproductive capabilities of Asian carp in natural waters of the United States. Spatial and temporal trends in larval production have been described in the unimpounded sections of the Missouri River (Schrank et al. 2001), and reproductive status and larval densities have been quantified in the lower Illinois River (DeGrandchamp et al. 2007), but no studies have quantified reproductive ability in the pools of the UMRS relative to the open river. Given current concerns of the ability of Asian carp to establish and disperse within the pools of the UMRS and adjacent waterways (i.e. the Illinois River and the Great Lakes), special attention should be given to spatial components of their reproductive ability and cues for successful spawning.
As the literature suggests (Nikolsky 1963; Verigin et al. 1978; Jennings 1988; Costa-Pierce 1992; Schrank et al. 2001), larval production peaked in spring when discharge and water level were high or rising. Within these periods of high water, back-calculated birth dates coincided with even small increases in discharge (as little as 122 m3/s) after water temperatures reached a suitable range. Schrank et al. (2001) also reported larval production in the Missouri River in association with similar magnitudes of discharge increase (about 100 m3/s). Thus, even small increases in discharge may cue Asian carp spawning provided that water levels are high enough and water temperatures are within the suitable range.
Considerable spatial variation of larval production occurred, with most larval production occurring in the open river. While discharge among all sites was correlated, differences in water level regimes in the open river versus water level management in the UMRS pools may affect larval production. In the open river, changes in water level were consistent throughout the reach insofar as an increase in water level upstream generally coincided with an increase in water level downstream. This likely provided cues for movement to spawning areas and facilitated reproduction (DeGrandchamp et al. 2007).
In contrast to the open river, frequently incongruent water levels in pools may have reduced reproductive success. For instance, DeGrandchamp et al. (2007) reported limited spawning (as observed by high fecundity and GSI values, reabsorption of eggs, and low larval production) in the Illinois River in 2005 and suggested that it may have been linked to declining river flow associated with the dry spawning season. In the pools, regulation at the dams reduces water velocity by interrupting flow during spring and summer, particularly in dry years, such as 2005 and 2006, when less water was allowed to pass through gates at the dams. While water velocities at the upstream section of the pools tend to be adequate early in the spawning season, water velocities at the downstream section are often lower than the suitable limit and may contribute to low larval production in the pools during dry years. Larval Asian carp occurring in downstream sections of the pools in 2005 and 2006 may have drifted from upstream reaches and became entrained at the lower dams.
In 2007, our limited sampling suggested a different scenario in the pooled UMRS because a substantial density of larvae occurred in Pool 24. In 2007, a stronger spring flood pulse occurred (i.e., higher discharge and open river conditions) relative to the two previous years. Peak discharge at the upstream section of Pool 24 during 2007 was 976 m3/s greater than in 2005 and 1326 m3/s greater than in 2006. Additionally, gates at lock and dam 24 were open 52 days between mid-March and mid-May in 2007. In contrast the gates were only open for 6 and 15 days in April 2005 and 2006, respectively. The higher discharge and open gates in the spring of 2007 may have cued movement of adult Asian carp into Pool 24, and thus caused adult spawning and produced larvae.
Recruitment to juvenile stage
Characteristic differences in river conditions between the pools and the open river contributed to variation in larval production. However, these differences may have less of an effect on recruitment to the juvenile stage. Reaches that produced relatively high densities of larvae (i.e., the open river and Pool 26) contained juveniles from the same cohort. Juvenile birth dates that did not overlap with larval birth dates were probably a result of larvae that were present as eggs (which we did not identify) rather than larvae in tows. Our results suggest that year-class strength may depend more on adult production of larvae rather than survival to the juvenile stage. However, over-winter survival of juveniles was not quantified, and differences among reaches may contribute to recruitment to age-1 (see Garvey et al. 1998). This may be particularly important for the few larvae produced in late summer; it is questionable that resulting late-spawned juveniles would reach sizes by fall that ensure adequate energy reserves and winter survival (Garvey et al. 2002).
Implications
During 2005 and 2006, water level management at the UMRS dams within the range of this study apparently limited larval production of Asian carp and subsequent recruitment to the juvenile stage. This implies that during dry spawning seasons, managers can anticipate poor year-classes in the pools under current water level management practices. Asian carp appear to have particular spawning requirements that are limited to a relatively short period each spring (or late summer). If these conditions are not met, adults may not reproduce and simulations suggest that the sensitivity of the populations to these dry events relative to the climatic frequency of dry weather at pools will affect the persistence of the two invaders. The same trend of consistently low larval production during dry spawning seasons cannot be anticipated in the open river; substantial larval production occurred in the open river (especially in 2006) despite a dry spring. So while a series of poor Asian carp year-classes may occur in the pools, the recruits in the open river may replenish populations in lower pools given their propensity for movement (see DeGrandchamp et al. 2008).
A combination of commercial harvest and species-selective barriers may help control the Asian carp populations in the pools. Promotion of commercial harvest in the UMRS following a series of dry years and poor year-classes would reduce resident populations. This would require the development of new markets and incentives for harvesters (Conover et al. 2007). Emplacing physical or behavioral barriers at dams (as described in Conover et al. 2007) to reduce the movement of adults from the open river to the UMRS pools would limit the Asian carps’ ability to replenish these purported sink areas.
By comparing larval Asian carp production in the open river and pools and then inferring recruitment to the juvenile stage, we have begun to show that viewing invasive species in a spatial context might hold promise for limited their spread and persistence in novel or even currently occupied ecosystems. By targeting the mechanism of population growth (i.e., reproduction), managers can influence the establishment and subsequent dispersal of these and other invasive species.
References
Burr BM, Eisenhour DJ, Cook KM, Taylor CA, Seegert GL, Sauer RW et al (1996) Nonnative fishes in Illinois waters: what do the records reveal? Trans Ill State Acad Sci 89(1/2):73–91
Chapman DC (2006) Early development of four cyprinids native to the Yangtze River, China. U.S. Geological Survey Data Series 239
Chick JH, Pegg MA (2001) Invasive carp in the Mississippi River Basin. Science 292(5525):2250–2251. doi:10.1126/science.292.5525.2250
Colautti RI, MacIssac HJ (2004) A neutral terminology to define ‘invasive’ species. Divers Distrib 10:135–141. doi:10.1111/j.1366-9516.2004.00061.x
Conover G, Simmonds R, Whalen M (eds) (2007) Management and control plan for bighead, black, grass, and silver carps in the United States. Asian Carp Working Group. Aquatic Nuisance Species Task Force, Washington, DC, 223 pp
Costa-Pierce BA (1992) Review of the spawning requirements and feeding ecology of silver carp (Hypophthalmichthys molitrix) and reevaluation of its use in fisheries and aquaculture. Rev Aquat Sci 6(3/4):257–273
DeGrandchamp KA (2006) Habitat selection and movement of bighead carp and silver carp in the lower Illinois River. Thesis, Southern Illinois University, Carbondale, IL
DeGrandchamp KL, Garvey JE, Csoboth LA (2007) Linking adult reproduction and larval density of invasive carp in a large river. Trans Am Fish Soc 136:1327–1334. doi:10.1577/T06-233.1
DeGrandchamp KL, Garvey JE, Colombo RE (2008) Habitat selection and dispersal of invasive Asian carps in a large river. Trans Am Fish Soc 137:33–44. doi:10.1577/T06-116.1
Fuller PL, Nico LG, Williams JD (1999) Nonindigenous fishes introduced into inland waters of the United States. American Fisheries Society, Special Publication 27, Bethesda, MD
Garvey JE, Wright RA, Stein RA (1998) Overwinter growth and survival of age-0 largemouth bass: revisiting the role of body size. Can J Fish Aqua Sci 55:2414–2424
Garvey JE, Herra TP, Leggett WC (2002) Protracted reproduction in sunfish: the temporal dimension in fish recruitment revisited. Ecol Appl 12(1):194–205. doi:10.1890/1051-0761(2002)012[0194:PRISTT]2.0.CO;2
Garvey JE, Dugger BD, Whiles MR, Adams SR, Flinn MB, Burr BM et al (2003a) Responses of fish, waterbirds, invertebrates, vegetation, and water quality to environmental pool management: Mississippi River Pool 25. U.S. Army Corps of Engineers, St. Louis District, Final report
Garvey JE, Rettig JE, Stein RA, Lodge DM, Klosiewski SP (2003b) Scale-dependent associations among fish predation, littoral habitat, and distributions of crayfish species. Ecology 84(12):3339–3348. doi:10.1890/02-0444
Gorbach EI, Krykhtin ML (1980) Maturation rate of the white amur Ctenopharyngodon idella and silver carp Hypophthalmichthys molitrix in the Amur River. J Ichthyol 21(5):835–843
Huet M (1970) Textbook of fish culture: breeding and cultivation of fish. Fishing News Limited, London
Jennings DP (1988) Bighead carp (Hypophthalmichthys nobilis): a biological synopsis. U.S. Fish and Wildlife Service. Biol Reprod 88(29)
Koel TM, Irons KS, Ratcliff E (2000) Asian carp invasion of the Upper Mississippi River System. Long term resource monitoring, program status report (PSR 2000–2005)
Kolar CS, Lodge DM (2001) Progress in invasion biology: predicting invaders. Trends Ecol Evol 16:199–204
Kolar KS, Chapman DC, Courtenay WR Jr, Housel CM, Williams JD, Jennings DP (2005) Asian carps of the genus Hypophthalmichthys (Pisces, Cyprinidae)—a biological synopsis and environmental risk assessment. U.S. Fish and Wildlife Service
Laird CA, Page LM (1996) Non-native fishes inhabiting the streams and lakes of Illinois. Ill Nat Hist Surv Bull 35(1):1–51
Lodge DM et al (1998) Predicting impact of freshwater exotic species on native biodiversity: challenges in spatial scaling. Aust J Ecol 23(1):53–67. doi:10.1111/j.1442-9993.1998.tb00705.x
Murty DS, Sukmaran KK, Reddy PVGK, Dey RK (1986) Observations on the life history of silver carp Hypophthalmichthys molitrix (Valenciennes). J Inland Fish Soc India 18:4–14
Nikolsky GV (1963) The ecology of fishes. Academic Press, London, 353 pp
Pflieger WL (1997) The fishes of Missouri, 2nd edn. Missouri Department of Conservation, Jefferson City
Rahel FJ (2000) Homogenization of fish faunas across the United States. Science 288:854–856. doi:10.1126/science.288.5467.854
Sampson SJ (1999) Dietary overlap between two Asian carp and three native filter feeding fishes of the Illinois and Mississippi Rivers. Thesis, Iowa State University, Ames, IA
Schlosser IJ (1998) Fish recruitment, dispersal, and trophic interactions in a heterogeneous lotic environment. Oecologia 113(2):260–268. doi:10.1007/s004420050377
Schrank S, Braaten PJ, Guy CS (2001) Spatiotemporal variation in density of larval bighead carp in the Lower Missouri River. Trans Am Fish Soc 130:809–814. doi:10.1577/1548-8659(2001)130<0809:SVIDOL>2.0.CO;2
Soin SG, Sukhanova AI (1972) Comparative morphological analysis of the development of the grass carp, the black carp, the silver carp and the bighead (Cyprinidae). J Ichthyol 12(1):61–71
Sokal RR, Rohlf FJ (1995) Biometry, 3rd edn. Freeman, New York
Tucker JK, Cronin FA, Hrabik RA, Petersen MD, Herzog DP (1996) The bighead carp (Hypophthalmichthys nobilis) in the Mississippi River. J Freshwat Ecol 11(2):241–243
Tweb A, Ahmed A, Baroi P, Mustafa G (1990) Studies on the development of the silver carp, Hypophthalmichthys molitrix (Val.). Bangladesh J Zool 18(2):139–145
Verigin BV, Makeyeva AP, Zaki Mokhamed MI (1978) Natural spawning of the silver carp, Hypophthalmichthys molitrix, the bighead carp Aristichthys nobilis, and the grass carp, Ctenopharyngodon idella, in the Syr-Dar’ya River. J Ichthyol 18(1):143–146
Williamson CJ, Garvey JE (2005) Growth, fecundity, and diets of newly established silver carp in the middle Mississippi River. Trans Am Fish Soc 134:1423–1430. doi:10.1577/T04-106.1
Acknowledgments
We thank the many staff and students of the Fisheries and Illinois Aquaculture Center for their assistance in the field and laboratory. Ed Heist and Greg Whitledge provided useful comments. This research was supported by the Aquatic Nuisance Species Program through the U.S. Army Corps of Engineers.
Author information
Authors and Affiliations
Corresponding author
Rights and permissions
About this article
Cite this article
Lohmeyer, A.M., Garvey, J.E. Placing the North American invasion of Asian carp in a spatially explicit context. Biol Invasions 11, 905–916 (2009). https://doi.org/10.1007/s10530-008-9303-5
Received:
Accepted:
Published:
Issue Date:
DOI: https://doi.org/10.1007/s10530-008-9303-5