Abstract
The Predation Danger Hypothesis suggests that shorebirds adjust aspects of their annual schedules to limit the amount of time they are exposed to the danger posed by the presence of Peregrine Falcons (Falco peregrinus) and other raptors. For example, Pacific Dunlin (Calidris alpina pacifica) remain near their breeding grounds on the Yukon-Kuskokwim Delta, Alaska to molt, and migrate southward after peak Peregrine Falcon migration has passed. This extended residence in the north may enable them to increase the time they devote to reproductive activities and enhancing their reproductive output, either by increasing the length of bi-parental brood care or by producing more clutches. In this paper, I describe the breeding ecology of Pacific Dunlin and relate my results to their migration strategy. Pacific Dunlin females deserted their broods, on average, at 5 days of age, leaving the remainder of the 19-day brood care period to their mates. This resembles the parental care patterns of other Calidris species. However, Pacific Dunlin exhibited a high propensity for replacing first clutches that failed (75%), and 17% of individuals who successfully hatched their first nest deserted their broods and went on to produce a clutch with a new mate (i.e., double brood). These rates are high compared to other subspecies of Dunlin suggesting that Pacific Dunlin take advantage of their extended time on the breeding grounds to increase their reproductive output.
Zusammenfassung
Die „Predation Danger”-Hypothese legt nahe, dass Küstenvögel bestimmte Aspekte ihres „Jahreskalenders” so ausrichten, dass sie die Zeit, die sie unter direkter Bedrohung durch Wanderfalken (Falco peregrinus) und andere Raubvögel stehen, möglichst klein halten. So bleiben zum Beispiel die Alpenstrandläufer (Calidris alpina pacifica) über die Mauser nahe ihrer Brutgebiete im Yukon-Kuskokwim-Delta, Alaska, und ziehen erst nach Süden, wenn der größte Pulk der ziehenden Wanderfalken durchgezogen ist. Dieses längere Verbleiben im Norden ermöglicht es ihnen vermutlich, mehr Zeit in die Reproduktionsaktivitäten zu stecken und damit ihren Bruterfolg zu vergrößern, entweder durch eine längere Brutpflege durch beide Elternteile, oder durch eine größere Anzahl Gelege. In dieser Arbeit beschreibe ich die Brut-Ökologie des Alpenstrandläufers und setze meine Ergebnisse in Beziehung zu ihrer Zug-Strategie. Die Weibchen des Alpenstrandläufers verlassen ihre Brut im Schnitt nach fünf Tagen und überlassen die restlichen 19 Tage Brutpflege ihren Partnern, was den Brutpflege-Gewohnheiten anderer Calidris-Arten ähnlich ist. Die Alpenstrandläufer jedoch zeigen eine starke Neigung, Erst-Gelege mit geringem Bruterfolg (75%) zu ersetzen; 17% der Individuen, die das Erst-Gelege erfolgreich ausgebrütet hatten, verließen ihre Brut und produzierten ein zweites Gelege mit einem neuen Partner (i.e., doppelte Brut). Diese Raten sind hoch im Vergleich mit anderen Strandläufer-Unterarten und lassen vermuten, dass Alpenstrandläufer ihre längere Verweildauer im Brutgebiet dazu nutzen, die Zahl ihrer Nachkommen zu vergrößern.
Similar content being viewed by others
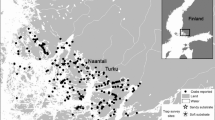
Explore related subjects
Discover the latest articles, news and stories from top researchers in related subjects.Avoid common mistakes on your manuscript.
Introduction
Southward-migrating Peregrine Falcons (Falco peregrinus) and other raptor species form a component of the predator landscape on the migration flyways of North America with special relevance to the migration of prey species (Lank et al. 2003; Ydenberg et al. 2007). The southward propagation of the wave of migratory raptors has a specific phenology and geography (Worcester and Ydenberg 2008), and may have a strong influence on the migration and molt of shorebirds. In the annual schedule of birds, these periods represent times of extra vulnerability to predation because their flight performance is impaired by the high wing loading caused by large fuel loads (Kullberg et al. 2000; Burns and Ydenberg 2002) or reduced aerodynamics due to the loss of flight feathers (Swaddle and Witter 1997). The Predation Danger Hypothesis proposes that adult shorebirds may adjust the timing of their molt and southward migration to minimize their exposure to migrating raptors during these periods (Lank et al. 2003). This can be achieved by either (1) leaving the breeding grounds early, migrating to their wintering (or staging sites) and molting before the arrival of the falcons or (2) delaying their departure and molt on or near the breeding areas and migrating after the falcons.
Shorebirds that migrate after the falcons not only benefit from increasing their likelihood of surviving the migration but they could also use their extended time on the breeding grounds to increase their reproductive output. They might do this by investing more time in parental care to enhance their offsprings’ probability of survival, lay more replacement clutches if they experience nest failure, and/or they may attempt to carry out additional breeding attempts (i.e., double brood). Not all northern breeding shorebirds are capable of adopting a migrate-late strategy. For example, at some sites, weather conditions become too harsh in late summer/early autumn to make it profitable for birds, particularly small ones, to remain to molt; while in other cases birds may need to migrate at rather specific times to take advantage of particular ephemeral conditions (e.g., prevailing winds, flooding of salt flats) that would be unavailable to them later in the year.
The Pacific Dunlin (Calidris alpina pacifica) has a typical Calidris parental care pattern with bi-parental incubation and asymmetrical male-biased brood care (Borowik and McLennan 1999); however, they are one of the few Calidris sandpipers that adopt a migrate-late strategy (Cramp and Simmons 1983; Poole 2009). They nest throughout the coastal regions of southwest Alaska (del Hoyo et al. 1996) and, following breeding, they move to nearby coastlines to molt, then in October and November migrate to their wintering grounds along the Pacific Coast from Canada to Mexico (Jamieson 2009). In this paper, I describe the breeding ecology of the Pacific Dunlin on the Yukon-Kuskokwim Delta, Alaska, with particular focus on brood care duration and second clutch production.
Methods
I studied the nesting ecology of Pacific Dunlin near Kanaryarmiut Field Station, Yukon Delta National Wildlife Refuge, Alaska (61°22′N, 165°07′W) from late April to late July 2004–2006. Pacific Dunlin nested on graminoid-dominated lowland wet meadows interlaced with small ponds and rivers. I measured Dunlin breeding phenology over an area totalling 0.58 km2, which was surveyed every 1–3 days. Every individual Dunlin observed was noted. All nesting territories defended by males were plotted and searched systematically for nests. Each nest was visited daily until the clutch was complete (i.e., four eggs or contained the same number of eggs for three consecutive days). The date the last egg was laid was defined as day zero of the incubation period (referred to as the ‘incubation date’), and the hatching date was estimated by adding 22 days to this date, which is the average incubation duration of Dunlin (Holmes 1966; this study). If a clutch was complete when found, I used the flotation method to estimate its incubation date (Liebezeit et al. 2007). If the eggs of a clutch floated at 90°, the eggs were examined every 3 days for signs of hatching and the incubation date was back-calculated from the hatch date. There were numerous nests that were depredated prior to hatch and, in many of these cases, I was unable to assign incubation dates.
Once the clutch was complete, I trapped and banded both parents. Each bird was fitted with a metal leg-band and a unique combination of three color bands. The sex of each adult was determined by culmen length (females ≥39.8 mm, males ≤37.7 mm; Page 1974) and when possible, confirmed with observations of mating behavior.
I checked the nests every 3 days for incubation activity, and daily as the estimated hatch date approached. If a nest was depredated, the predation date was assumed to have occurred at the midpoint between the date the nest was found depredated and the prior visit. If I suspected a nest had been abandoned, I repositioned one of the eggs and checked the nest the following day (incubating shorebirds position eggs with the narrow ends inward, resembling a four-leaf clover). If the egg had been returned to its proper position I presumed the nest was still being incubated; otherwise I recorded it as abandoned. The date of abandonment was assumed to have occurred midway between the last day a parent was observed incubating and the subsequent visit.
Dunlin are capable of renesting (i.e., laying a replacement clutch after the failure of a previous nest; Soikkeli 1967; Jönsson 1991; Naves et al. 2008). However, after nest failure, an individual does not necessarily re-pair with their mate of the previous nest to renest. Tracking the renesting activities of females is more difficult than males, because they are not as tightly attached to a territory. Therefore, if a female did not re-pair with her previous mate, I could not ascertain if she had terminated breeding for the year or had renested outside the study area. For this reason, I only report renesting activities of males because they remained associated with their territories throughout the breeding season regardless if they had an active nest. I am confident that I was able to document most, if not all, of their renesting activities.
After hatch, I visited broods at least once a day and recorded which parent(s) was providing brood care. I defined brood care as leading chicks to safe feeding areas, brooding, and protecting them from predators and getting lost. I was often unable to ascertain how many chicks were present due to their secretive nature and the height of the surrounding vegetation. A parent was considered to have deserted if it was not observed with its brood for three consecutive days. In some cases, the brood was depredated before either parent deserted. A brood was considered depredated if I was unable to locate it for three consecutive days before it reached 19 days of age. No broods reappeared after a 3-day absence and there were no broods discovered from previously unknown nests.
Dunlin are able to double brood (i.e., after deserting their first brood, individuals paired with new mates and produced second clutches; Heldt 1966; Soikkeli 1967). The proportion of individuals that double brooded was determined by dividing the number of known double brooders by the total number of first nests that successfully hatched.
Hatching and reproductive success were calculated using the Mayfield method (Mayfield 1975; Manolis et al. 2000). Hatching success is the probability a clutch hatches, and reproductive success is the probability a clutch survives incubation with ≥1 chick of the resulting brood surviving to fledge. A brood was considered fledged if ≥1 chick survived 19 days.
I tested which factors affected maternal care duration using linear mixed models with the following variables—dependent variable: log10 of maternal care duration; fixed independent variables: year, hatch period, nest type (i.e., whether the nest was the first nest of a single or double brooding female or a replacement nest); random independent variable: female. The maternal care duration data were log-transformed because they did not meet the assumption of normality (Shapiro–Wilk tests, P < 0.001, following transformation P = 0.054). I divided the hatching season into three periods (6–19 June, 20–30 June, 1–17 July). The first period is when double brooding individuals hatch their first nests, the second period represents the time when individuals whose nests fail may lay replacement nests, and the third period represents the time when individuals no longer replace lost nests. Post hoc comparisons were made between these periods using Tukey’s honestly significant differences tests.
A logistic regression was used to examine whether there was a significant effect of date on the likelihood a failed nest would be replaced. I used Pearson’s correlation to test for a relationship between day of incubation that a nest was lost and how long it took the female to lay a replacement clutch. I conducted two-sample t tests to determine whether there was a significant difference between the number of days it took a familiar pair, compared to a newly formed pair, to lay a replacement clutch and to compare the brood care duration of double brooding and non-double brooding females. I used the Chi-square statistic to investigate whether the rate of double brooding varied between years.
All statistical analyses were conducted with SYSTAT 12. Critical alpha was set at 0.05. All means are presented with ± 95% confidence intervals.
Results
General breeding ecology
I documented 30 nesting territories in 2004, 37 in 2005, and 39 in 2006 at the study site, corresponding to a density of 52–67 nests/km2. I found a total of 148 Dunlin nests on these territories over three summers (Table 1), for a mean of 1.4 nesting attempts per territory per year. The average laying season length was 40 days. The commencement of the laying period varied by 16 days between years (13–29 May), but the cessation of laying varied by only 6 days (25 June–1 July; Fig. 1).
Annual chronology of Pacific Dunlin (Calidris alpina pacifica) nests laid on the Yukon-Kuskokwim Delta, Alaska. Incubation date is the date that the last egg of a clutch is laid. Sample sizes do not necessarily match those listed in Table 1 because some nests were depredated before an incubation date could be estimated
Overall hatching and reproductive success varied between years: hatching success was highest in 2006 (59%) and lowest in 2004 (41%), while reproductive success was highest in 2006 (45%) and lowest in 2005 (20%). In all years and for all nests combined, hatching success and reproductive success were 47 and 29%, respectively (Table 1). The probability that a clutch hatched or produced a fledgling varied throughout the season (Fig. 2). The general pattern was that hatching and reproductive success increased during the first 3 weeks of laying, peaked in late May and early June, followed by a general decline.
The seasonal dynamics of mean hatching and reproductive success of Pacific Dunlin breeding on the Yukon-Kuskokwim Delta. Hatching success, represented by the black circles and solid line, is the Mayfield estimate of how many clutches successfully hatched while reproductive success, represented by the gray circles and dashed line, is the Mayfield estimate of how many clutches produced at ≥1 fledgling
I determined the timing of female desertion for 60 broods over the 3 years of study. In all but three cases, females deserted prior to their mates. The amount of brood care provided by deserting females varied greatly, with some deserting the day of hatch while others remained with the brood for 12 days (Fig. 3). On average, females spent 5.0 ± 1.1 days attending their broods. When examining which factors influenced when a female deserted, there was no significant effect of year (F 2,11 = 3.006, P = 0.091), nor whether the nest was the first nest of a single or double brooding female or a replacement nest (F 2,11 = 2.994, P = 0.092). The random variable, female identity was uninfluential (SE = 0.20) compared to the model intercept (SE = 0.74). The period during which the nest hatched had a significant effect on the amount of care a female provided (F 2,11 = 13.317, P = 0.001). Females whose nests hatched in the earliest part of the season provided 3.7 ± 1.5 days (n = 19), in mid-season females provided 5.8 ± 1.3 days (n = 25), and towards the end of the season females remained with their broods for 2.5 ± 0.8 days (n = 13). Post hoc comparisons showed that the amount of care provided late in the season was significantly less than the amount of care provided during the other two periods (early P = 0.014, mid P = 0.001). The duration of care provided during the early and middle parts of the season did not significantly differ (P = 0.303).
The amount of time female Pacific Dunlin dedicated to brood care relative to the hatch date of their broods. Circles signify individuals that deserted their broods into the care of their mates and were not observed for the rest of the season, triangles females that deserted and went on to double brood, and squares females that were deserted by their mates. Symbols are colored according to year of collection (white 2004, gray 2005, and black 2006)
Renesting
I found a total of 43 replacement nests. In 2004, 12 first replacement nests and 2 second replacement nests were found. In 2005, 21 first and 2 second replacement nests were found. In 2006, only 6 replacement nests were found, none of which were second replacement nests. In all years combined, first replacement nest hatching success was 40% and reproductive success was 12% (Table 1). The hatching and reproductive success of the 4 second replacement nests was 56 and 26%, respectively (Table 1).
There was a significant seasonal decline in the probability that a failed clutch would be replaced (odds ratio = 0.828, \( \chi_{1}^{2} \) = 44.222, n = 74, P < 0.001; Fig. 4). Males whose nests failed after 16 June never produced a replacement clutch, although they often remained on their territories displaying until the end of the laying period (i.e., the end of June).
In Pacific Dunlin breeding on the Yukon-Kuskokwim Delta, the probability that a nest would be replaced after failing was significantly negatively affected by the date it was lost. Each point represents an individual nest and the curved line represents logistic regression curve of logit (p) = 30.828–0.189 × date of failure, P < 0.001
In 31 cases, I identified the female(s) of the failed nest and replacement nest. When the female remained with her original mate to renest, the average interval between the date the first clutch failed and the initiation date of the replacement nest was 9.3 ± 0.9 days (n = 17; range 7–14 days). There was a positive correlation between the day of incubation on which the first clutch was lost and the time lapsed until completion of the second clutch (r = 0.526, P = 0.030; Fig. 5). If a male renested with a new partner the average time between the failure date of his original clutch and the initiation date of the replacement clutch was 15.1 ± 3.2 days (n = 14; range 8–31 days). This was significantly longer than the renesting interval of pairs that remained together (t 29 = 3.732, P = 0.001).
There was a significant positive correlation between how far along in incubation a clutch is lost and the number of days the pair takes to lay a new clutch for Pacific Dunlin nesting on the Yukon-Kuskokwim Delta, Alaska. The data point furthest to the right represents a pair that lost their 2-day-old brood and renested. They were the only pair to renest after the loss of a brood
Double brooding
Among adults that deserted their first broods, 17% attempted to double brood on the study site: six females (two of which did so in two consecutive years) and one male (who attempted to do so in two consecutive years). There was variation between years in the degree of double brooding (2004: 21%; 2005: 33%; 2006: 8%), however these differences were not significant (χ 2 = 5.991, df = 2, P = 0.146). Details of the individuals that double brooded are listed in Table 2. Hatching and reproductive success of nests that were a result of double brooding was 33 ± 5 and 9 ± 4%, respectively.
Females that went on to double brood provided, on average, 2.6 ± 1.0 days of brood care for their original brood (n = 8; range 0–4 days). In contrast, females in the rest of the population provided care for an average of 5.4 ± 1.3 days (n = 52; range 0–19 days). This difference was not statistically significant (t 58 = 1.656, P = 0.103).
There were no cases in which a pair produced a second clutch after successfully fledging their first brood, nor were there cases in which an individual went on to double brood after deserting a clutch that was the result of renesting.
Discussion
Despite the fact that prolonged maternal brood care can enhance reproductive output in shorebirds (Székely and Williams 1995), female Pacific Dunlin breeding on the Yukon-Kuskokwim Delta do not use the extra time in the north provided by molting prior to migration to extend brood care duration. The average amount of time a female Dunlin devoted to a brood’s care was 5.0 ± 1.1 days, well within the range of female brood care duration of other species of Calidris sandpipers that adopt a migrate-early strategy (e.g., Least Sandpipers C. minutilla 6 days, Miller 1985; Semipalmated Sandpipers C. pusilla 6 days, Gratto-Trevor 1991; Western Sandpipers C. mauri 7.1 days, Ruthrauff et al. 2009).
Instead, Pacific Dunlin apparently take advantage of their prolonged residence time on the breeding grounds to extend the laying season by investing in more nesting attempts. Their average laying period was 40 days. This is longer than the laying periods of other Dunlin subspecies (C. a. arcticola 33 days, Holmes 1966; C. a. schinzii 34 days, Soikkeli 1967; C. a. centralis 20 days, Schekkerman et al. 2004). It is also longer than the laying period of sympatrically nesting Western Sandpipers (\( {\bar{\text{x}}} \) = 32 days; Jamieson 2009). This extended laying season allowed Pacific Dunlin to renest at a high frequency. I documented the failure of 52 first nests, of which at least 39 were replaced (i.e., 75%; Table 1). This is high compared to other subspecies of Dunlin. For example, in a 4-year study in northern Alaska of the 158 nests with ≥1 adult marked, only five cases of Dunlin renesting were detected (C. a. arcticola; Naves et al. 2008) and a Finnish population of Dunlin had a renesting rate of 41% (C. a. schinzii; Soikkeli 1967, 1970).
The probability that a failed nest was replaced decreased as the season progressed. Although the males that failed remained until the end of the egg laying period, many of the females who failed late in the season left and move to the coast (Gill and Handel 1990) rather than attempt to renest. I suggest that this decrease in renesting probability late in the season is due to late clutches having a very low likelihood of succeeding. When males did renest, approximately half of them remained with their original mate (55%). In these cases, the average time lag between nest failure and initiation of the replacement clutch was 9 days, while in newly formed pairs the average male’s time lag was 15 days. In northern latitudes where the time available for breeding can be limiting, this may result in males that are unable to maintain the pair bond with their original mate sustaining a substantial cost.
The previous pairing history of the two parents was not the only factor that influenced how much time passed between the first and second clutch. The age of the lost clutch also had a significant effect. The further along in incubation a pair was, the longer it took the female to lay a replacement clutch. During the peak laying period, gonad size is at a maximum but decreases throughout the remainder of the season (Holmes 1966), suggesting that the later in incubation a clutch is lost the more gonadal recession has occurred and thus more time is needed for the gonads to return to functional size.
Previous works reported that North American Dunlin did not double brood (i.e., C. a. arcticola, C. a. hudsonia, and C. a. pacifica; Holmes 1966, 1971; Warnock and Gill 1996); however, I discovered that Pacific Dunlin double brooded at a rather high frequency; a minimum 17% of individuals that successfully hatched their first brood and deserted went on to double brood. Double brooding has been reported in European Dunlin; however, it does not appear to be a regular breeding strategy (Heldt 1966; Soikkeli 1967). For example, Soikkeli (1967) found only 4% of Dunlin breeding in Finland (C. a. schinzii) that successfully hatched their first nest and deserted went on to double brood. Moreover, to my knowledge, Pacific Dunlin are the only Dunlin population where a male has been observed double brooding. Again, I suggest that the high propensity for double brooding is due to the extended breeding season which is a result of their migration strategy.
Females that double brooded were amongst the first to lay every year and provided on average only 2.6 days of brood care. The amount of care provided by the other females was on average 5.4 days, after which they left the breeding grounds presumably to go to the coast to stage and begin molt (Gill and Handel 1990), although undoubtedly some double brooded outside the study area and were not detected. This early desertion by the double brooding females may lead to a lower survivorship of the first brood (Székely and Williams 1995); however, the overall increase in lifetime reproductive output resulting from the laying of a second clutch likely outweighs such costs.
The amount of maternal brood care varied significantly throughout the season; at the beginning and end of the breeding season, females provided little care compared to the mid-season. A similar pattern was also observed in parental care of female Kentish Plovers (Charadrius alexandrinus; Székely and Williams 1994). These plovers have a similar mating-parental care strategy as Dunlin nesting on Yukon-Kuskokwim Delta (Székely and Lessells 1993). I suggest that, early in the season, females desert so that they can seek additional mating opportunities; however, by mid-season, they would be unable to manage another breeding attempt in the time remaining in the breeding season, and therefore try to maximize their fitness by extending their current parental care. As the season progresses, the costs of providing care may begin to outweigh the benefits, resulting in reduced care towards the end of the season. This may be due to either an increase in predation risk to the female herself on the breeding grounds and/or a decrease in the value of the brood due to their lower fledging probability. Females may also desert so that they may avoid overlapping their breeding and molting activities (a concession that late breeding Pacific Dunlin males often make; unpublished data).
In summary, Pacific Dunlin nesting on the Yukon-Kuskokwim Delta have an unusually long breeding season for an arctic shorebird species. They take advantage of this long season to enhance their reproductive output, not by prolonging brood care but by increasing their number of nesting attempts. They renest and double brood at very high frequencies, 75 and 17%, respectively. I suggest that this long laying season is a result of the Dunlin remaining in the north to molt and migrating in fall in order to avoid migrating with Peregrine Falcons. Data from Dunlin nesting in Finland further support this hypothesis. These Dunlin, C. a. schinzii, breed at a similar latitude as Pacific Dunlin (61°N) but they leave the breeding grounds early and migrate before the passage of southward migrating falcons (Jamieson 2009). Compared to Pacific Dunlin, they have a short laying period (34 days), low renesting frequency (41%), and low prevalence of double brooding (n = 3; Soikkeli 1967), thus further supporting the hypothesis that predation mitigating migration strategies can influence breeding investment.
Naturally, I am not suggesting that predation pressure on the post-breeding migration is the only, or even the strongest, factor influencing the scheduling of breeding in birds. Other factors that can have a strong influence on the timing of breeding include food availability (Meltofte et al. 2007; Wegge et al. 2010), climatic conditions (Love et al. 2010), predation pressure on the breeding grounds (Smith et al. 2010), and the physiological condition of the adults (Bêty et al. 2003; Ninni et al. 2004). I am merely suggesting that in the future researchers consider the possible impact that migration danger can have on events during the breeding season.
References
Bêty J, Gauthier G, Giroux JF (2003) Body condition, migration, and the timing of reproduction in Snow Geese: A test of the condition-dependent model of optimal clutch size. Am Nat 162:110–121
Borowik OA, McLennan DA (1999) Phylogenetic patterns of parental care in Calidridine sandpipers. Auk 116:783–799
Burns JG, Ydenberg R (2002) The effects of wing loading and gender on the escape flights of least sandpipers (Calidris minutilla) and western sandpipers (Calidris mauri). Behav Ecol Sociobiol 52:128–136
Cramp S, Simmons KEL (1983) The birds of the Handbook of the birds of Europe, the Middle East and North Africa: the birds of the western Palearctic. Oxford University Press, Oxford
del Hoyo J, Elliot A, Sagatal J (1996) Handbook of the birds of the world, vol. 3: Hoatzins to Auks. Lynx, Barcelona
Gill RE, Handel CM (1990) The importance of subarctic intertidal habitats to shorebirds: A study of the central Yukon-Kuskokwim Delta, Alaska. Condor 92:709–725
Gratto-Trevor CL (1991) Parental care in the semipalmated sandpiper Calidris pusilla: brood desertion by females. Ibis 133:394–399
Heldt R (1966) Zur Brutbiologie des Alpenstrandläufers, Calidris alpina schinzii. Corax 1:173–188
Holmes RT (1966) Breeding ecology and annual cycle adaptations of the red-backed sandpiper (Calidris alpina) in northern Alaska. Condor 68:3–46
Holmes RT (1971) Latitudinal differences in the breeding and molt schedules of Alaskan red-backed sandpipers (Calidris alpina). Condor 73:93–99
Jamieson SE (2009) Cross-seasonal factors affecting breeding investment by female Pacific Dunlins. PhD dissertation, Simon Fraser University
Jönsson PE (1991) Reproduction and survival in a declining population of the southern dunlin Calidris alpina schinzii. Wader Study Group Bull 61:56–68
Kullberg C, Jakobsson S, Fransson T (2000) High migratory fuel loads impair predator evasion in sedge warblers. Auk 117:1034–1038
Lank DB, Butler RW, Ireland J, Ydenberg R (2003) Effects of predation danger on migration strategies of sandpipers. Oikos 103:303–319
Liebezeit JR, Smith PA, Lanctot RB, Schekkerman H, Tulp I, Kendall SJ, Tracy DM, Rodrigues RJ, Meltofte H, Robinson JA, Gratto-Trevor CL, McCaffery BJ, Morse JA, Zack SW (2007) Assessing the development of shorebird eggs using the flotation method: species-specific and generalized regression models. Condor 109:32–47
Love OP, Gilchrist HG, Descamp S, Semeniuk CAD, Bêty J (2010) Pre-laying climatic cues can time reproduction to optimally match offspring and ice conditions in an Arctic marine bird. Oecologia 164:277–286
Manolis JC, Andersen DE, Cuthbert FJ (2000) Uncertain nest fates in songbird studies and variation in Mayfield estimation. Auk 117:615–626
Mayfield HF (1975) Suggestions for calculating nest success. Wilson Bull 87:456–466
Meltofte H, Høye TT, Schmidt NM, Forchhammer MC (2007) Differences in food abundances cause inter-annual variation in the breeding phenology of High Arctic waders. Polar Biol 30:601–606
Miller EH (1985) Parental behavior in the least sandpiper (Calidris minutilla). Can J Zool 63:1593–1601
Naves LC, Lanctot RB, Taylor AR, Coutsoubos NP (2008) How often do Arctic shorebirds lay replacement clutches? Wader Study Group Bull 115:2–9
Ninni P, De Lope F, Saino N, Haussy C, Møller AP (2004) Antioxidants and condition-dependence of arrival date in a migratory passerine. Oikos 105:55–64
Page G (1974) Age, sex, molt and migration of dunlin at Bolinas Lagoon. West Birds 5:1–12
Poole A (2009) The Birds of North America Online. Cornell Laboratory of Ornithology. http://bna.birds.cornell.edu/bna/ Accessed 01 September 2009
Ruthrauff DR, Keller JN, Rizzolo DJ (2009) Ecological factors regulating brood attendance patterns of the western sandpipers Calidris mauri. Ibis 151:523–534
Schekkerman H, Tulp I, Calf KM, de Leeuw JJ (2004) Studies on breeding shorebirds at Medusa Bay, Taimyr, in summer 2002. Alterra report 922, Wageningen
Smith PA, Gilchrist HG, Forbes MR, Martin JL, Allard K (2010) Inter-annual variation in the breeding chronology of arctic shorebirds: Effects of weather, snow melt and predators. J Avian Biol 41:292–304
Soikkeli M (1967) Breeding cycle and population dynamics in the dunlin (Calidris alpina). Ann Zool Fenn 4:258–298
Soikkeli M (1970) Mortality and reproductive rates in a Finnish population of dunlin Calidris alpina. Ornis Fenn 47:149–158
Swaddle JP, Witter MS (1997) The effects of molt on the flight performance, body mass, and behaviour of European starlings (Sturnus vulgaris): an experimental approach. Can J Zool 75:1135–1146
Székely T, Lessells CM (1993) Mate change by Kentish povers Charadrius alexandrinus. Ornis Scand 24:317–322
Székely T, Williams TD (1994) Factors affecting timing of brood desertion by female Kentish plovers Charadrius alexandrinus. Behaviour 130:17–28
Székely T, Williams TD (1995) Costs and benefits of brood desertion in female Kentish plovers, Charadrius alexandrinus. Behav Ecol Sociobiol 37:155–166
Warnock ND, Gill RE (1996) Dunlin. In: Poole A, Gill F (eds) The Birds of North America, No. 203. Birds of North America, Inc., Ithaca
Wegge P, Vesterås T, Rolstad J (2010) Does timing of breeding and subsequent hatching in boreal forest grouse match the phenology of insect food for chicks? Ann Zool Fenn 47:251–260
Worcester R, Ydenberg R (2008) Cross-continental patterns in the timing of southward peregrine falcon migration in North America. J Raptor Res 42:13–19
Ydenberg R, Butler RW, Lank DB (2007) Effects of predator landscapes on the evolutionary ecology of routing, timing and molt by long-distance migrants. J Avian Biol 38:523–529
Acknowledgments
This research was supported by the Centre for Wildlife Ecology at Simon Fraser University, Environment Canada, Natural Sciences and Engineering Research Council (NSERC), US Fish and Wildlife Service, and the Northern Scientific Training Program. I would like to thank Catherine Dale, Émilie Germaine, Jean-Francoise Lamarre, Casper van Leeuwen, Sarah Lovibond, Olivier Meyer, and Erica van Rooij for their assistance in the field. Comments by Dov Lank, Heather Major, Kyle Morrison, Joe Nocera, Erica Nol, Tony Williams, and Ron Ydenberg greatly improved earlier drafts of this manuscript. This study complied with the current American and Canadian laws and permits were granted by Simon Fraser University, the State of Alaska, and the US Fish and Wildlife Service.
Author information
Authors and Affiliations
Corresponding author
Additional information
Communicated by P. H. Becker.
Rights and permissions
About this article
Cite this article
Jamieson, S.E. Pacific Dunlin Calidris alpina pacifica show a high propensity for second clutch production. J Ornithol 152, 1013–1021 (2011). https://doi.org/10.1007/s10336-011-0691-4
Received:
Revised:
Accepted:
Published:
Issue Date:
DOI: https://doi.org/10.1007/s10336-011-0691-4