Abstract
Nitrogen (N) has been suggested as a determinant of seed production especially in species with high seed N content. Assuming that seed yield was determined as the balance between N demand and supply for seed production, we studied the effect of N fertilization after flowering on soybean (Glycine max L. Merr.) yield. Seed N concentration was nearly constant irrespective of N fertilization, indicating that seed production was proportional to the amount of N available for seed growth. N demand for seed production was analyzed as the product of seed number, the rate of N filling in individual seeds, and the length of the reproductive period. N fertilization increased seed number and the reproductive period, but did not influence the N filling rate. Seed number was positively correlated with dry mass productivity after flowering. Three N sources were distinguished: mineral N uptake, symbiotic N2 fixation and N remobilization from vegetative body. N fertilization increased N uptake and N remobilization, but lowered N2 fixation. We concluded that N availability in the reproductive period determined seed yield directly through increasing N supply for seed growth and indirectly through increasing seed N demand with enhanced plant dry mass productivity.
Similar content being viewed by others
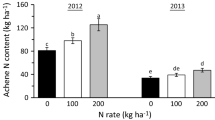
Explore related subjects
Discover the latest articles, news and stories from top researchers in related subjects.Avoid common mistakes on your manuscript.
Introduction
Seed production is closely related to plant ecological fitness and crop productivity. It is crucial for success of the species in the natural environment and for food production in agricultural systems. Nitrogen (N) has been suggested as a determinant of seed production especially in species with high seed N content (Sinclair and de Wit 1975; Henry et al. 1992; Lawlor 2002; Sinclair and Vadez 2002; Hirose et al. 2005). This is because N is more limiting than carbon in producing seeds with high N content. Kinugasa et al. (2003) grew an annual herb Xanthium canadense at elevated CO2 and found no increase in seed yield despite the increase in carbon availability with elevated CO2. Seed production in annual species was enhanced by elevated CO2 only when N allocation to seeds increased (Miyagi et al. 2007). Thus seed yield is restricted by the amount of N allocated to seed, with seed N concentration being more or less constant within species.
Soybean (Glycine max L. Merr.) requires a large amount of N for seed production (Sinclair and de Wit 1975), and hence its yield may be sensitive to N fertilization after flowering. However, positive effect of N fertilization after flowering on soybean yield was not always found in published studies (Salvagiotti et al. 2008). This may imply that factors other than N supply contribute to the determination of seed N and dry mass yield. Hirose et al. (2005) presented a simple model that described seed yield as a function of N demand and supply in seed production. The model, assuming constant seed N concentration, determined seed yield by the N when seed N demand exceeds the plant potential to supply N for seed, and predicted changes in seed yield in response to the environment. Here, we hypothesize that seed yield is constrained by factors that determine N demand as well as N supply for seed production.
N demand for seed may be determined by the product of three factors: seed number, the rate of N filling in individual seeds, and the length of the reproductive period. N fertilization may not stimulate the rate of N filling in individual seeds when sink activities are limited (Lhuillier-Soundele et al. 1999a). However, it may increase the number of pods and seeds due to enhanced availability of photosynthate (Egli and Zen-wen 1991; Board and Tan 1995; Jiang and Egli 1995) and extend the reproductive period due to delay in leaf senescence (Sinclair and de Wit 1975, 1976). There are three N sources for seed production in soybean: mineral N uptake from soil, symbiotic fixation of atmospheric N2 and N remobilization from vegetative body. N fertilization may increase mineral N uptake but lower symbiotic N2 fixation because high soil nitrate levels inhibit N2 fixation (Hunt and Layzell 1993; Voisin et al. 2002; Fujikake et al. 2002; McKenzie et al. 2003). It may also suppress N remobilization from vegetative body if external N supply meets N demand for seed production (Sunarpi and Anderson 1997; Imsande and Schmidt 1998).
In this paper, we address the factors that restrict seed yield. Assuming constant seed N concentration, we expect that seed production is determined by the amount of N allocated to seeds (Sinclair and Vadez 2002; Hirose et al. 2005). We analyze the response of soybean yield to N fertilization after flowering as a consequence of changes in N demand and supply in seed production. If N fertilization do not increase seed yield, it will result from reduction in N2 fixation and/or N remobilization from vegetative body. If N fertilization increase seed yield, it will result from increased mineral N uptake that outweighs reduction in N2 fixation. Enhanced N supply will be associated with the increase in either seed number, N filling rate, or length of the reproductive period.
Materials and methods
Experimental design
Nodulating soybean (Glycine max L. Merr. cv. Enrei) and its non-nodulating mutant (cv. En1282) were employed for the experiment (Francisco and Akao 1993). The latter was used as a control to estimate N2 fixation in the former. Both have a determinate flowering habit (Oikawa et al. 2010). Seeds of both lines were sown on 8 July 2005 on moistened paper in plastic dishes. Radicles emerged in a day after sowing. Germinated seeds were transplanted each in a pot (4.0 L volume) filled with well-washed Tottori dune sand inoculated with Bradyrhizobium japonicum. The number of plants prepared for the experiment was 113 and 92 for cv. Enrei and cv. En1282, respectively. Pots were placed in a greenhouse in the Arid Land Research Center, Tottori University, Japan (35°32′N and 134°13′E). Mean monthly temperatures in the greenhouse were 28.7°C in July, 29.4°C in August, 25.7°C in September and 19.4°C in October.
Plants were grown with weekly N additions. Plants received uniform N fertilization until flowering (2.94 mgN week−1). After flowering, plants were divided into three groups with different N fertilization rates: one with N equal to that before flowering (MN, 2.94 mgN week−1), one with N ten times of MN (HN, 29.4 mgN week−1) and one with one tenth of MN (LN, 0.294 mgN week−1). Standard nutrient solution followed Epstein (1972): N 16 (NO3 −:NH4 + = 7:1), P 2, K 6, Ca 4, S 1, and Mg 1 mmol L−1. N in the solution had a mean δ15N value of 12.0 ± 0.5‰ in excess against atmospheric air. 15/8 strength of this solution was prepared for HN. Solutions for MN and LN were prepared from the HN solution replacing NH4 + with Na+ and NO3 − with Cl−. Pots received 70 ml of the solution every week from 1 week after germination. Plants received tap water as needed during the experiment. Pots were rotated periodically to minimize differences in growth conditions among plants.
Measurements
The date of flowering was recorded when the first flower appeared and the date of death when all leaves dropped and the stem turned brown. Plants were harvested at about 3-week intervals, and at seed maturation (plant death). Harvests after flowering corresponded to R1 (beginning of blooming), R4 (full pod), R6 (full seed) and R8 (full maturity). Four plants were sampled per treatment at each harvest. Harvested plants were separated into leaves, stems, roots, dead leaves, dead petioles, nodules, and reproductive structures (pods and seeds). The number of nodules was counted. Leaf area was determined with a leaf area meter (AAL-410, Hayashi Denko, Japan). Dry mass of plant parts was determined after oven drying at 80°C for more than 3 days. Samples were ground in a mill and their N content and δ15N values were measured by an elemental analyzer (Flash EA1112, Thermo Electron, Milan, Italy) coupled to an isotope ratio mass spectrometry (IRMS) via ConFlo III interface (Delta Plus XP, Thermo Fisher Scientific, Bremen, Germany).
The percentage N derived from symbiotic N2 fixation (Ndfa) in the whole plant of nodulating soybean (Enrei) was calculated every harvest with the following equation:
where δ15Na, δ15Nb and δ15Nc represent δ15N value in non-nodulating soybean (cv. En1282), nodulating cv. Enrei and soybean grown with N only from symbiotic fixation. In this study, −1.7‰ was used for δ15Nc value in soybean (Yoneyama et al. 1986, 2000).
The model
If seed yield (Y) is limited by the amount of N allocated to seeds, it is determined as the seed N (Y N) divided by seed N concentration ([Nse]):
Considering N sources for soybean, the following equation can be obtained:
where U N, F N and R N represent seed N supplied from mineral N uptake, N2 fixation and N remobilization from vegetative body, respectively. Assuming leaves as the main source of N remobilization and all remobilized N is allocated to seed (Egli et al. 1978; Zeiher et al. 1982), R N was calculated as the reduction in leaf N (the sum of N in living and dead leaves) during the reproductive period. Efficiency of N remobilization was calculated as a quotient of remobilized N to the foliage N at the first harvest after flowering (R4) when foliage N reached the maximum. The sum of U N and F N, i.e. newly acquired N, was obtained by subtracting R N from Y N. We assumed that the ratio of U N to F N was the same as that of mineral N uptake to N fixation in plant N acquisition. N demand in seed production was analyzed as:
where S, A N and D represent seed number, the rate of N filling in individual seeds, and the length of the reproductive period, respectively.
We calculated model variables with the assumption that the rank order of individuals in terms of total dry mass did not change with plant growth. Statistical analyses were conducted with JMP software (JMP 7, SAS Institute Inc., USA). To test the effects of N treatments on variables, a post hoc test (Tukey–Kramer method) was used after applying ANOVA.
Results
N fertilization did not influence seed N concentration ([Nse], Fig. 1a), supporting the assumption that seed has a constant N concentration. N fertilization, however, significantly increased seed N yield (the amount of N in seed at the end of the growth period, Y N, Fig. 1b). Seed dry mass yield (Y) thus increased with N fertilization (Eq. 2, Fig. 1c).
a Seed N concentration ([Nse]), b seed N (Y N) and c seed dry mass yield (Y) of soybean grown with different N fertilization after flowering: LN (0.294 mgN week−1), MN (2.94 mgN week−1) and HN (29.4 mgN week−1). Different alphabets above columns indicate statistically significant difference (Tukey–Kramer method, P < 0.05). Error bars indicate ±1 SE (n = 4)
Figure 2 shows contributions of three N sources to Y N (Eq. 3). The amount of mineral N taken up from soil (U N) increased with increasing N levels, although the difference between LN and MN was not significant (Fig. 2a). U N accounted for Y N by 4.3, 17.4 and 77.7% at LN, MN and HN, respectively. N2 fixation (F N) was significantly smaller at HN than the other two N treatments (Fig. 2b). The fraction of Y N derived from F N was 87.9, 74.5 and 5.8% at LN, MN and HN, respectively. Remobilized N from vegetative body (R N) was largest at HN (Fig. 2c). R N accounted for 7.7, 8.1 and 16.5% of Y N at LN, MN and HN, respectively.
Sources of seed N (Y N): a seed N supplied from mineral N uptake (U N), b symbiotic N2 fixation (F N) and c remobilization from vegetative body (R N). Open, grey and black columns represent LN, MN and HN treatments, respectively. Different alphabets above columns indicate statistically significant difference (Tukey–Kramer method, P < 0.05). Error bars indicate ±1 SE (n = 4)
The rate of N2 fixation per plant in the reproductive period was significantly larger in LN and MN than HN plants (Table 1). The number of nodules averaged over the reproductive period was not different between N treatments. The rate of N2 fixation per nodule, i.e. nodule activity, decreased at high N fertilization. Efficiency of N remobilization was not significantly different between N treatments, although the maximum foliage N was significantly larger at higher N fertilization (Table 2).
N demand in seed production was analyzed with Eq. 4. Seed number (S) was larger in HN than the other two treatments (Fig. 3a). S was positively correlated with the plant dry mass production in the early reproductive period (R1–R4) (P < 0.05, r 2 = 0.66, Fig. 4). N filling rates in individual seeds (A N) were not significantly different between N levels (Fig. 3b). The reproductive period (D) was 16 days longer in HN than the other two treatments (Fig. 3c). N in individual seeds (A N × D) increased with N fertilization, implying that dry mass of individual seeds increased as well.
a Seed number (S), b the rate of N filling in individual seeds (A N), and c the length of the reproductive period (D). Open, grey and black columns represent LN, MN and HN treatments, respectively. Different alphabets above columns indicate statistically significant difference (Tukey–Kramer method, P < 0.05). Error bars indicate ±1 SE (n = 4)
Discussion
N fertilization after flowering significantly increased plant growth and seed yield (Y) (Fig. 1). The positive response of Y to N fertilization after flowering has been reported in many studies (Afza et al. 1987; Wood et al. 1993; Hayati 1995; Wesley et al. 1998; Gan et al. 2002), though no response was also reported (Schmitt et al. 2001; Gutierrez-Boem et al. 2004; Barker and Sawyer 2005; Salvagiotti et al. 2008). The increase in Y was caused by the increase in seed N (Y N) with constant N concentration of seed ([Nse]) (Fig. 1). A meta-analysis of 61 soybean studies showed that the variation of [Nse] was considerably narrow: an average 6.34% with an interquartile range of 6–6.75% (Salvagiotti et al. 2008). Values of [Nse] in the present study were within this interquartile range. Constant [Nse] implies that seed production is proportional to N allocation to seeds (Sinclair and de Wit 1975; Sinclair and Vadez 2002; Kinugasa et al. 2003; Hirose et al. 2005; Miyagi et al. 2007).
N fertilization increased N uptake (U N) and remobilization (R N), but decreased N2 fixation (F N) (Fig. 2). Reduction in N2 fixation with N fertilization has been ascribed to inhibition of nodule formation and/or nitrogenase activity (Sprent et al. 1988; Lucinski et al. 2002) by high soil nitrate levels (Hunt and Layzell 1993; Voisin et al. 2002; Fujikake et al. 2002; McKenzie et al. 2003). As the number of nodules did not differ between N treatments (Table 1), the decrease in F N at HN should have been caused by the reduction in nitrogenase activity.
In contrast to our expectation, N fertilization increased the amount of N supplied from remobilization from vegetative body (R N) in both absolute and relative terms (Fig. 2c), though its contribution to Y N was relatively small. The increase in R N with N fertilization resulted from the increase in foliage N pool available for remobilization, because N remobilization efficiency did not change with N fertilization (Table 2). N allocation to vegetative structures continued after flowering in soybean (Pahalwan and Tripathi 1984; Warembourg and Fernandez 1985; Vessey et al. 1990). No reduction in the efficiency of leaf N remobilization with N fertilization has been reported in many studies (e.g. Huang et al. 2008; Bancel 2009; Norris and Reich 2009). A survey of more than 30 studies covering 60 species showed no response of leaf N remobilization efficiency to increased nutrient availability in about two-thirds of experiments (Aerts 1996).
The increase in Y N with N fertilization was caused mainly by the increase in seed number (S) (Fig. 3a). Soybean aborts 20–80% of flowers and immature pods (Van Schaik and Probst 1958; Wiebold et al. 1981; Heitholt et al. 1986). If the number of flowers was determined before different fertilization treatments, different number of seeds might reflect the number of aborted flowers and pods. The final pod and seed number was determined by the availability of photosynthates in the early reproductive period (from flowering to pod formation, R1–R5) (Board and Tan 1995, Jiang and Egli 1995). In the present study, S was positively correlated with dry mass productivity in the early reproductive period (R1–R4, Fig. 4). Larger dry mass productivity in HN plants is attributable to their larger canopy leaf area and higher leaf N (both more than 1.5 times larger than those in the other treatments).
It was suggested that the reproductive period (D) would extend with N fertilization due to delay in leaf senescence (Sinclair and de Wit 1975, 1976; Hirose et al. 2005). Several studies reported lengthening of D with N fertilization (Egli et al. 1985; Munier-Jolian and Duthion 1996; Egli 2004). In the present study, however, D lengthened at HN only, with no shortening at LN (Fig. 3c). Its contribution to the increase in Y N was relatively small when compared with that of S.
To our knowledge, changes in seed N filling rate (A N) with N fertilization have not been reported in soybean. In pea, however, A N increased with the amount of N available for seed growth until a maximum A N was reached due to source limitation (Lhuillier-Soundele et al. 1999a, b). A N of soybean did not significantly respond to N fertilization (Fig. 3b), indicating that N availability was large enough for seed production, where seed production might have been sink- rather than source-limited.
Seed N in individual seeds (A N × D) and consequently seed size increased significantly with increasing N fertilization. Actually seed size increased by 57% (LN vs. HN), while seed number by 117%. Soybean responded to different N availability by changing seed number more than seed size, which was in line with the general trend (Bradshaw 1965; Harper et al. 1970; Borras et al. 2004). High plasticity in seed number may allow for variable response to resource availability. Less variable seed size may also have been reinforced by agronomic selection (Sadras 2007).
Conclusions
Seed N concentration was nearly constant irrespective of N fertilization after flowering, and therefore seed production was proportional to the amount of N available for seed growth. N fertilization increased seed N and thus seed dry mass yield. The increase in seed N and dry mass yield was associated with the increase in seed number with a less variable seed size. The number of seeds was determined by dry mass productivity at the early stage in the reproductive period. N availability in the reproductive period determined seed yield directly through increasing N supply for seed growth and indirectly through increasing seed N demand with enhanced plant dry mass productivity.
References
Aerts R (1996) Nutrient resorption from senescing leaves of perennials: are there general patterns? J Ecol 84:597–608
Afza R, Hardarson G, Zapata F, Danso S (1987) Effects of delayed soil and foliar N fertilization on yield and N2 fixation of soybean. Plant Soil 97:361–368
Bancal P (2009) Decorrelating source and sink determinism of nitrogen remobilization during grain filling in wheat. Ann Bot 103:1315–1324
Barker DW, Sawyer JE (2005) Nitrogen application to soybean at early reproductive development. Agron J 97:615–619
Board JE, Tan Q (1995) Assimilatory capacity effects on soybean yield components and pod number. Crop Sci 35:846–851
Borras L, Slafer GA, Otegui ME (2004) Seed dry weight response to source-sink manipulations in wheat, maize and soybean: a quantitative reappraisal. Field Crop Res 86:131–146
Bradshaw AD (1965) Evolutionary significance of phenotypic plasticity in plants. Adv Genet 13:115–155
Egli DB (2004) Seed-fill duration and yield of grain crops. Adv Agron 83:243–279
Egli DB, Zhen-wen Y (1991) Crop growth rate and seeds per unit area in soybean. Crop Sci 31:439–442
Egli DB, Leggett JE, Duncan WG (1978) Influence of N stress on leaf senescence and N redistribution in soybeans. Agron J 70:43–47
Egli DB, Guffy RD, Meckel LW, Leggett JE (1985) The effect of source-sink alterations on soybean seed growth. Ann Bot 55:395–402
Epstein E (1972) Mineral nutrition of plants: principles and perspectives. Wiley, New York
Francisco PBJ, Akao S (1993) Autoregulation and nitrate inhibition of nodule formation in soybean cv. Enrei and its nodulation mutants. J Exp Bot 44:547–553
Fujikake H, Yashima H, Sato T, Ohtake N, Sueyoshi K, Ohyama T (2002) Rapid and reversible nitrate inhibition of nodule growth and N2 fixation activity in soybean (Glycine max (L.) Merr.). Soil Sci Plant Nutr 48:211–217
Gan Y, Stulen I, Posthumus F, van Keulen H, Kuiper P (2002) Effects of N management on growth, N2 fixation and yield of soybean. Nutr Cycl Agroecosyst 62:163–174
Gutiérrez-Boem FH, Scheiner JD, Rimski-Korsakov H, Lavado RS (2004) Late season nitrogen fertilization of soybeans: effects on leaf senescence, yield and environment. Nutr Cycl Agroecosyst 68:109–115
Harper JL, Lovell PH, Moore KG (1970) The shapes and sizes of seeds. Annu Rev Ecol Syst 1:327–356
Hayati R, Egli DB, Crafts-Brandner SJ (1995) Carbon and nitrogen supply during seed filling and leaf senescence in soybean. Crop Sci 35:1063–1069
Heitholt JJ, Egli DB, Leggett JE (1986) Characteristics of reproductive abortion in soybean. Crop Sci 26:589–595
Henry LT, Raper CDJ, Rideout JW (1992) Onset of and recovery from nitrogen stress during reproductive growth of soybean. Int J Plant Sci 153:178–185
Hirose T, Kinugasa T, Shitaka Y (2005) Time of flowering, costs of reproduction, and reproductive output in annuals. In: Reekie EG, Bazzaz FA (eds) Reproductive allocation in plants. Elsevier, San Diego, pp 159–188
Huang JY, Zhu XG, Yuan ZY, Song SH, Li X, Li LH (2008) Changes in nitrogen resorption traits of six temperate grassland species along a multi-level N addition gradient. Plant Soil 306:149–158
Hunt S, Layzell DB (1993) Gas-exchange of legume nodules and the regulation of nitrogenase activity. Annu Rev Plant Physiol Plant Mol Biol 44:483–511
Imsande J, Schmidt JM (1998) Effect of N source during soybean pod filling on nitrogen and sulfur assimilation and remobilization. Plant Soil 202:41–47
Jiang H, Egli DB (1995) Soybean seed number and crop growth rate during flowering. Agron J 87:264–267
Kinugasa T, Hikosaka K, Hirose T (2003) Reproductive allocation of an annual, Xanthium canadense, at an elevated carbon dioxide concentration. Oecologia 137:1–9
Lawlor DW (2002) Carbon and nitrogen assimilation in relation to yield: mechanisms are the key to understanding production systems. J Exp Bot 53:773–787
Lhuillier-Soundele A, Munier-Jolain N, Ney B (1999a) Influence of nitrogen availability on seed nitrogen accumulation in pea. Crop Sci 39:1741–1748
Lhuillier-Soundele A, Munier-Jolain NG, Ney B (1999b) Dependence of seed nitrogen concentration on plant nitrogen availability during the seed filling in pea. Eur J Agron 11:157–166
Lucinski R, Polcyn W, Ratajczak L (2002) Nitrate reduction and nitrogen fixation in symbiotic association Rhizobium-legumes. Acta Biochim Pol 49:537–546
McKenzie FR, Jacobs JL, Riffkin P, Kearney G, McCaskill M (2003) Long-term effects of multiple applications of nitrogen fertiliser on grazed dryland perennial ryegrass/white clover dairy pastures in southwest Victoria. 1. Nitrogen fixation by white clover. Aust J Agric Res 54:461–469
Miyagi KM, Kinugasa T, Hikosaka K, Hirose T (2007) Elevated CO2 concentration, nitrogen use, and seed production in annual plants. Glob Change Biol 13:2161–2170
Munier-Jolain NG, Ney B, Duthion C (1996) Termination of seed growth in relation to nitrogen content of vegetative parts in soybean plants. Eur J Agron 5:219–225
Norris M, Reich P (2009) Modest enhancement of nitrogen conservation via retranslocation in response to gradients in N supply and leaf N status. Plant Soil 316:193–204
Oikawa S, Miyagi KM, Hikosaka K, Okada M, Matsunami T, Kokubun M, Kinugasa T, Hirose T (2010) Interactions between elevated CO2 and N2-fixation determine soybean yield—a test using a non-nodulated mutant. Plant Soil 330:163–172
Pahalwan D, Tripathi R (1984) Nodulation, accumulation and redistribution of nitrogen in soybean (Glycine max (L.) Merrill) as influenced by seed inoculation and scheduling of irrigation. Plant Soil 81:235–246
Sadras VO (2007) Evolutionary aspects of the trade-off between seed size and number in crops. Field Crop Res 100:125–138
Salvagiotti F, Cassman KG, Specht JE, Walters DT, Weiss A, Dobermann A (2008) Nitrogen uptake, fixation and response to fertilizer N in soybeans: a review. Field Crop Res 108:1–13
Schmitt MA, Lamb JA, Randall GW, Orf JH, Rehm GW (2001) In-season fertilizer nitrogen applications for soybean in Minnesota. Agron J 93:983–988
Sinclair TR, de Wit CT (1975) Photosynthate and nitrogen requirement for seed production by various crops. Science 189:565–567
Sinclair TR, de Wit CT (1976) Analysis of the carbon and nitrogen limitations to soybean yield. Agron J 68:319–324
Sinclair TR, Vadez V (2002) Physiological traits for crop yield improvement in low N and P environments. Plant Soil 245:1–15
Sprent JI, Stephens JH, Rupela OP (1988) Environmental effects on nitrogen fixation. In: Summerfield RJ (ed) World crops: cool season food legumes. Kluwer, Dordrecht, pp 801–810
Sunarpi, Anderson JW (1997) Effect of nitrogen nutrition on remobilization of protein sulfur in the leaves of vegetative soybean and associated changes in soluble sulfur metabolites. Plant Physiol 115:1671–1680
van Schaik PH, Probst AH (1958) The inheritance of inflorescence type, peduncle length, flowers per node, and percent flower shedding in soybeans. Agron J 50:98–102
Vessey JK, Raper CD, Henry LT (1990) Cyclic variations in nitrogen uptake rate in soybean plants—uptake during reproductive growth. J Exp Bot 41:1579–1584
Voisin AS, Salon C, Munier-Jolain NG, Ney B (2002) Quantitative effects of soil nitrate, growth potential and phenology on symbiotic nitrogen fixation of pea (Pisum sativum L.). Plant Soil 243:31–42
Warembourg FR, Fernandez MP (1985) Distribution and remobilization of symbiotically fixed nitrogen in soybean (Glycine max). Physiol Plant 65:281–286
Wesley TL, Lamond RE, Martin VL, Duncan SR (1998) Effects of late-season nitrogen fertilizer on irrigated soybean yield and composition. J Prod Agric 11:331–336
Wiebold WJ, Ashley DA, Boerma HR (1981) Reproductive abscission levels and patterns for eleven determinate soybean cultivars. Agron J 73:43–46
Wood CW, Torbert HA, Weaver DB (1993) Nitrogen fertilizer effects on soybean growth, yield, and seed composition. J Prod Agric 6:354–360
Yoneyama T, Nakano H, Kuwahara M, Takahashi T, Kambayashi I, Ishizuka J (1986) Natural 15N abundance of field grown soybean grains harvested in various locations in Japan and estimate of the fractional contribution of nitrogen fixation. Soil Sci Plant Nut 32:443–450
Yoneyama T, Fujiwara H, Engelaar W (2000) Weather and nodule mediated variations in delta13C and delta15N values in field-grown soybean (Glycine max L.) with special interest in the analyses of xylem fluids. J Exp Bot 51:559–566
Zeiher C, Egli DB, Leggett JE, Reicosky DA (1982) Cultivar differences in N redistribution in soybeans. Agron J 74:375–379
Acknowledgments
We are grateful to Atsushi Suetsugu, Kensuke Kondo, Tomoki Shimizu, Itsuhiko Ueyama, Ping An, Atsushi Tsunekawa and other members in the Arid Land Research Center, Tottori University, for their advice and technical help in the experiment. We also thank Tokachi Federation of Agricultural Cooperatives for offering Rhizobium and Shoichiro Akao for permission to use En1282. This work was supported in part by Sumitomo Foundation [050933].
Author information
Authors and Affiliations
Corresponding author
Rights and permissions
About this article
Cite this article
Kinugasa, T., Sato, T., Oikawa, S. et al. Demand and supply of N in seed production of soybean (Glycine max) at different N fertilization levels after flowering. J Plant Res 125, 275–281 (2012). https://doi.org/10.1007/s10265-011-0439-5
Received:
Accepted:
Published:
Issue Date:
DOI: https://doi.org/10.1007/s10265-011-0439-5