Abstract
This work presents a detailed investigation of the genomic region surrounding the PRNP gene in a sample of patients diagnosed with fatal familial insomnia (FFI) from several European countries, notably Spain. The main focus of the study was to explore the origins of the chromosomes carrying the D178N mutation by designing a single-nucleotide polymorphism (SNP) haplotype around the PRNP gene. Haplotypes were constructed by genotyping six SNPs (rs2756271, rs13040327, rs6037932, rs13045348, rs6116474, and rs6116475) in 25 FFI patients from all over Spain. To augment the geographical scope of our study, 13 further FFI cases from Germany (9) and Italy (4) were also examined. Genotyping of SNPs in conjunction with the analysis of genealogical data for a group of FFI patients revealed the existence of two distinct haplotypes potentially associated with the D178N mutation. Of them, GCATTA-M proved to be the common haplotype of Spanish patients, whereas ACATTA-M was typical of the German cases. It is interesting to note that both haplotypes were identified in the Italian samples: GCATTA-M in a family from the Tuscany region and ACATTA-M in a family from the Veneto region. Our findings suggest the occurrence of two independent D178N-129M mutational events in Europe, preserved and transmitted from one generation to the next until nowadays. Likewise, results based on the analysis of SNP data indicate that previous hypotheses postulating that the D178N mutation had independent origins for each family and that its global distribution was determined by recurrent mutational events must be regarded with caution.
Similar content being viewed by others
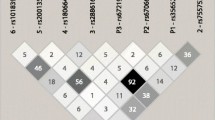
Avoid common mistakes on your manuscript.
Introduction
Fatal familial insomnia (FFI) is an autosomal dominant prion disease caused by a missense mutation at codon 178 of the prion protein gene (PRNP) [1] coupled with a methionine at the polymorphic site 129 of the same gene [2]. The D178N mutation consists of an A→G transition that gives place to an amino acid change (aspartic acid-178 is replaced by asparagine) in the sequence of the cellular prion protein (PrPC). It has been demonstrated that this amino acid substitution results in the formation of an insoluble, pathogenic prion protein (PrPres) that accumulates in the brain of the affected individuals, and the onset of the disease occurs when a critical amount of normally soluble PrPC is converted into the PrPres form [3]. The clinical presentation of the disease associated with the D178N-129M mutation of PRNP varies considerably from one patient to another, even among cases from the same family [4, 5]. Among FFI patients, methionine homozygous are usually characterized by a more rapid course of illness with a more sever insomnia, myoclonus, and prominent autonomic disturbances [6]. In contrast, methionine–valine (M/V) heterozygous individuals have a prolonged illness with presentation of ataxia and dysarthria, and tend to have more prominent cognitive impairment and seizures, while sleep disturbances and autonomic signs are less severe [7, 8].
The D178N point mutation has been linked to two distinct disorders, depending on the background genotype at codon 129 of the PRNP gene: FFI and familial Creutzfeldt–Jakob disease (CJD). A chromosome with the D178N mutation and valine at polymorphic codon 129 (D178N-129V) is associated with a typical CJD syndrome. As mentioned above, the D178N-129M chromosome is associated with a syndrome dominated by intractable insomnia [2]. However, although all of the FFI phenotypes feature a D178N-129M genotype, a number of genotypically identical patients have been reported to show clinical and neuropathological profiles compatible with CJD [4, 5, 9–11], suggesting that additional genetic and/or molecular factors might be involved in the development of these diseases.
The D178N mutation causing FFI is considered to be a rare mutation. Recently, in the framework of the standardized EUROCJD collaborative surveillance project, 66 cases of FFI were identified. This figure represents 1.5% (66/4,441) of all genetic transmissible spongiform encephalopathies (TSE) reported and 14.5% of genetic TSE [12]. Nevertheless, the fact that this neurodegenerative disorder features an adult onset, ranging from 20 to 61 years with an average of 50 [5, 8, 13], have undoubtedly influenced the preservation of the D178N mutation over time because the mutated individuals will have an offspring with a 50% probability of inheriting the mutation. Therefore, depending on the reproductive success of the members of the pedigrees carrying the D178N mutation, it will be possible to find a relatively high number of affected individuals in specific geographical regions [14].
According to updated information from the National Centre of Epidemiology (http://www.isciii.es/htdocs/centros/epidemiologia), there are approximately 35 FFI cases registered in Spain, of which about 46% have been reported by the Surveillance System of the Basque Country. The findings of a recent study by our research group suggest a founder effect of the D178N mutation in the Basque area as the most plausible explanation for the high number of FFI cases registered in this Spanish territory [15].
Previous investigations focusing on the founder effects of the PRNP gene have been performed by examining DNA microsatellites (short tandem repeats, STRs) [16, 17]. Thus, for instance, Dagvadorj et al. [17] who analyzed a set of STR loci around the PRNP gene in seven FFI families with different geographical origins, concluded that the distribution of the D178N mutation was determined by multiple independent mutational events that occurred in each of the studied pedigrees. However, it is known that microsatellites feature faster evolutionary rates than most other DNA molecular markers as a result of their comparatively high mutation rates, a phenomenon that might produce some bias when estimating divergence times [18] and establishing founder effects [19, 20]. Another characteristic of STRs susceptible of introducing bias is homoplasy, that is, two alleles identical by state will not necessarily share the same ancestry because the same allelic state can be obtained through different chains of mutational events [21].
To refine the findings emanating from investigations based only on STRs, specialists usually combine STRs with slowly evolving markers such as single-nucleotide polymorphisms (SNPs) or Alu insertions [18, 22, 23], characterized by low homoplasy and smaller mutation rates. Alternatively, many authors favor the analysis of haplotypes constructed from SNPs, a method that has become increasingly important for the study of founder mutations associated with human diseases [24–26].
This work presents a detailed investigation of the genomic region surrounding the PRNP gene in a sample of patients with a history of FFI from several European countries, notably Spain. We explored the origins of the chromosomes carrying the D178N-129M mutation by constructing a SNP haplotype around the PRNP gene. The haplotype consisted of six SNPs, all of which were genotyped in samples of FFI patients from Spain, Germany, and Italy, and in a control group of unrelated healthy individuals. To the best of our knowledge, this is the first study on the origin of FFI based on SNP markers.
Materials and methods
Sample selected for haplotyping
A sample of 25 Spanish individuals carrying the D178N-129M mutation of PRNP was collected to perform the present study. These cases were registered in the Transmissible Spongiform Encephalopathies Surveillance Systems from the Basque Country, Catalonia and Madrid (Fig. 1). The patients registered by the surveillance system of a given community do not necessarily stem from the same region. In this way, some affected individuals registered by the Basque Surveillance System had native ancestors from the North of Castile [15]. In addition, a control sample of 130 nonaffected individuals from the general population of Spain was included in the study as a reference group. Adherence to ethical guidelines was followed as stipulated by each of the institutions involved in the investigation. The study protocol was approved by the relevant ethics committees. Likewise, all patients signed the informed consent form before inclusion in the sample.
Geographic location (solid circles) of patients affected with FFI included in the study. Circle’s size is proportional to the number of FFI cases registered by the corresponding Surveillance System. Spanish regions are the Basque Country (BASQ), Madrid (MADR), and Catalonia (CATL). Other European samples included in the analysis were Germany (GERM) and the Italian regions of Tuscany (TUSC) and Veneto (VENT)
To broaden the geographical scope of the analysis, additional samples harboring the D178N-129M haplotype from Germany (N = 9) and Italy (N = 4) were also incorporated to our study. German samples were recorded in the country’s eastern region (mainly Saxony region), whereas Italian FFI cases belonged to two different genealogies: one from the Veneto region and the other one from the Tuscany region (Fig. 1).
The starting point of our investigation was the analysis of individual cases, a total of 38 FFI patients. During the course of the survey, we detected that 13 out of 25 Spanish cases had kinship relationships, which grouped into 5 independent genealogies. For the remaining Spanish cases, no common ancestor was known. Likewise, no kinship relationship was identified among German patients. In sum, 17 individual cases (13 from Spain and 4 from Italy) could be allocated to 7 different pedigrees: 5 Spanish and 2 Italian. Further information about the patients included in this study, such as age at onset, sex, duration of illness, and presenting clinical features, can be seen in an additional table (see the Electronic Supplementary Material).
Haplotype analysis
Six SNPs were selected for the haplotype construction: rs2756271, rs13040327, rs6037932, rs13045348, rs6116474, and rs6116475. These SNPs were chosen based on their chromosomal position (close to the D178N mutation) and on their allelic frequencies, according to the information compiled from publicly accessed databases with available genotyping data (GenBank database, NCBI). They are located on chromosome 20, between the PRNP gene and the MsP2 microsatellite [15, 27], outlining a genomic fragment of approximately 0.014 cM from rs2756271 to the PRNP178 mutation (Fig. 2). The SNP rs2756271 is situated at the PRNP 5′ extragenic region, at a distance of 0.014 cM of the polymorphism PRNP129, while rs13040327 is inserted in the PRNP promoter region, near the exon 1 [28] and around 0.013 cM of codon PRNP129. On the other hand, SNPs rs6037932, rs13045348, rs6116474, and rs6116475 are positioned in the intronic region, between exons 1 and 2 of PRNP. The distance from rs6037932 to codon 129 is 0.005 cM. The conversion of the intergenic distances was done based on the equivalence 1 cM equal to 106 base pairs. It is worth mentioning that SNPs rs13040327, rs2756271, rs6116474, and rs6116475 had been previously described and called 12533, 10870, 22814, and 22976, respectively, as can be seen in the reference U29185 of the NCBI (PRNP gene) [29]. The polymorphism described for PRNP codon 129 (PRNP129) was also considered in the analysis.
Genotyping of SNPs was performed by real-time PCR-based allelic discrimination assay with TaqMan MGB probes (Applied Biosystems), according to supplier’s conditions. In addition, conventional PCRs for each SNP were optimized for further sequence reactions to confirm the results of genotyping. In brief, 30 to 60 ng of DNA (obtained either from blood or buffy coat) were amplified in a final volume of 25 μl with 0.1 mM dNTP, 3 mM MgCl2 (1.5 mM for rs13040327), 0.2 μM of each primer, 0.5 U Taq polymerase (BioTaq, Bioline), 0.2 mg/ml BSA, and 1x Taq Buffer (Bioline). Further details regarding primer sequences and PCR conditions can be seen in Table 1.
The analysis of haplotype 178D/N-129M/V was performed by PCR followed by double restriction enzyme digestion. PCR amplifications were performed using 30 ng of DNA, 0.1 mM dNTP, 2 mM MgCl2, 0.2 μM of each primer, 0.5 U Taq Polymerase (BioTaq, Bioline), 1x Taq Buffer (Bioline), and 0.2 mg/ml BSA in a final volume of 25 μl. Primer sequences and PCR conditions are also detailed in Table 1. All PCR reactions were carried out in an iCycler Thermal Cycler (BioRad). Ten microliters of PCR product were first digested with 5 U/reaction of enzyme Tth111 I (New England Biolabs) in a final volume of 25 μl at 65°C for 3 h to genotype codon 178. Subsequently, 20 μl of the resultant product were digested with 0.1 U/reaction of enzyme HpyCH4IV (New England Biolabs) in a final volume of 25 μl, at 37°C for 3 h. Analysis of polymorphism 129 alone was performed under the conditions described above by digestion of 10 μl of PCR product with 0.1 U/reaction of enzyme HpyCH4IV. Results were electrophoresed in a 3% ethidium-bromide-stained agarose gel, and visualized under UV.
Anonymous, unrelated control samples from the Spanish general population were genotyped to ensure that all the SNPs involved and polymorphism PRNP129 were in Hardy–Weinberg equilibrium (HWE). HWE was tested using a modified version of the Markov chain random walk algorithm [30].
The existence of a common SNPs-PRNP129 haplotype in the group of FFI cases was deduced from parental and sibling relationships in those affected patients for which genealogical information was available. A maximum likelihood (ML) method, based on an expectation–maximization (EM) algorithm, was applied to estimate the haplotypic frequencies within the background population, considering gametic phase-unknown data (multilocus genotypic data). For this purpose, Arlequin v. 3.01 software [31] was utilized.
Results
The SNP genotyping data for the 38 European FFI cases examined (25 Spaniards, 9 Germans, and 4 Italians) are shown in Table 2. Due to obvious ethical reasons, only FFI-affected patients were analyzed (see Fig. 3). Logically, the lack of genotypic data for nonaffected relatives makes difficult the inference of SNP haplotypes associated with the D178N mutation. Thus, the identification of FFI cases with homozygous genotypes for all the SNP loci examined becomes a key starting point to further determine the haplotype(s) potentially linked to the D178N mutation. Bearing in mind that FFI is a genetic disorder inherited as an autosomal dominant trait, it should then be expected that the genetic markers transmitted along with the mutant allele are identical in affected individuals belonging to the same genealogy. In addition, because the SNPs selected to be analyzed are closely linked (<0.014 cM), probabilities of recombination within a genealogy are very low. In this way, once the SNP haplotype connected to the mutant allele has been identified in homozygous FFI patients, results can be reasonably extrapolated to the heterozygous patients of the same genetic lineage.
Pedigrees of Spanish cases of FFI. To protect the privacy of the patients’ living relatives, those family members in whose generation an FFI case was recorded are represented by white diamonds. The analyzed FFI cases are represented by solid rhombs. The black bar represents the haplotype constructed from six SNPs around the D178N mutation of the PRNP gene, following the order specified in the top right part of the figure
Out of 38 affected patients, 10 were homozygotes (S-01, S-05, S-15, I-01, I-03, and G-01 to G-05) for the whole set of SNPs selected for the haplotype construction (Table 2). From the thorough observation of the homozygous genotypes identified among the Spanish patients (S-01, S-05, and S-15), it seems apparent that GCATTA-M is the only haplotype that cosegregates with the D178N mutation. The availability of kinship information (genealogy data, Fig. 3) allowed establishing associations between the haplotype GCATTA-M and the D178N mutation in four further cases: S-02, S-03, and S-04 (relatives of S-01 and S-05, see pedigree A), and S-16 (relative of S-15, pedigree C). Genealogical data presented in pedigrees B and D also led to infer the existence of association between the cited haplotype (GCATTA-M) and the D178N mutation in some cases of heterozygous patients (S-06, S-07, S-17, and S-18). On the other hand, the kinship information available for S-13 and S-14 (siblings) did not help in establishing haplotype–mutant allele associations because the SNP rs6037932 was heterozygous in both cases (Table 2). As can be noted, all the Spanish cases examined so far showed a correlation between the GCATTA-M haplotype and the D178N mutation, irrespective of geographic origin in the Iberian Peninsula (see Fig. 1 and Table 2).
A number of FFI patients from Spain could not be allocated to any pedigree. Thus, for instance, it was not possible to accurately determine the haplotype connected to D178N in the patients S-08, S-09, S-10, S-11, and S-12 due to their heterozygous condition for the SNP rs2756271. Nonetheless, bearing in mind the common geographical origin of these patients (a small Basque rural community) with the cases grouped in pedigree A (S-01 to S-05), it seems very probable that they share a common SNP haplotype linked to the mutant allele.
The remaining Spanish FFI cases (S-19 to S-25) carried at least one heterozygous SNP marker, which, in conjunction with the lack of genealogy data, did not allow a reliable identification of the haplotype associated with the D178N mutation. Yet, neither these cases can be ruled out as carriers of GCATTA-M because their genotypes contain all the allelic variants whose combination gives place to the cited haplotype.
Unrelated healthy individuals (N = 130) from the Spanish general population were genotyped to test HWE in both the whole set of SNP loci examined and the polymorphism PRNP129. These molecular markers showed no deviation from HWE expectations. To exclude the possibility of the common haplotype being the more frequent in the general population, ML haplotype frequencies were estimated from the genotypic data by using an EM algorithm in the case of phase-unknown data. Estimates of haplotypic frequencies are shown in Table 3. The most frequent haplotype within the control group was by far ACATTA linked to PRNP129M (0.43), whereas the common haplotype identified in affected patients (GCATTA-M), despite being the second most abundant, reached a frequency of only 0.17. Results of a likelihood ratio test (G test) rendered highly significant differences (G = 22.90, df = 1, p < 0.001) for the frequencies of the GCATTA-M and ACATTA-M haplotypes between FFI patients and the control sample.
To determine how many common haplotypes there are in other European populations, several FFI cases from two additional Western European countries (four Italians and nine Germans) were also included in our study. It is interesting to note that we found that the SNP haplotype associated to the D178N mutation in two Italian cases from the Tuscany region (I-01 and I-02) was the same detected in the Spanish patients (GCATTA-M). The homozygous condition of I-01 and its genetic kinship with I-02 facilitated the inference of the common haplotype. On the other hand, the genotypic profile obtained for patient I-03 (Veneto region) points toward ACATTA-M as the common haplotype cosegregating with the D178N mutation. This haplotype inference can be extended to I-04, also from Veneto region, owing to the existence of kinship relationships between both patients. From these results, it is possible to deduce the existence of two distinct haplotypic combinations connected to the D178N mutation in Italy: one of them (GCATTA-M) is shared by Spanish and Italian patients, whereas a second haplotype (ACATTA-M) distinguishes from the previous one exclusively by the allelic variant of the SNP rs2756271.
Finally, we found that five (G-01 to G-05) out of nine German patients were homozygotes for all SNP markers. The homozygous condition of these patients allowed confirming the existence of a second common haplotype associated with the D178N mutation: ACATTA-M, coinciding with the Italian FFI cases from Veneto region. It was not possible to ascertain SNP haplotype–D178N mutation associations in the remaining German patients, owing mainly to the existence of at least one heterozygous SNP marker and also to the paucity of genealogical information.
Discussion
In this work, we have analyzed six SNPs in DNA samples from FFI patients and from individuals of the background population (control sample) to explore the origin of the chromosomes carrying the haplotype D178N-129M. Several features of the SNPs justify the selection of these molecular markers. Outstanding among them are their characteristic low mutation rates [32, 33], which make SNPs evolutionarily stable genetic loci (i.e., highly preserved from generation to generation), and therefore, particularly useful in founder effect studies. Specifically, the SNPs rs2756271, rs13040327, rs6037932, rs13045348, rs6116474, and rs6116475 were selected by considering proximity to the PRNP gene (around 0.014 cM), and their high polymorphism levels in the general population [29, NCBI data].
The PRNP gene has been the focus of several investigations aimed at establishing the founding events of the E200K [16, 34, 35] and D178N [15, 17] mutations, responsible for familial CJD and FFI, respectively. Studies centered on the founder effects of the D178N mutation have been carried out by means of the analysis of microsatellite loci (STRs). The mutation rates of microsatellites fluctuate depending on the nature of the specific STR [36]. Some recent works report mutation rate values of up to 1.5 × 10−3 per locus per generation for dinucleotidic STRs [37–39]. The elevated variability of the STR loci may, therefore, lead to an overestimation of the number of founder effects, especially when mutations are not recent. The results obtained upon the basis of STR data can then be seen as an estimate of the maximum number of “surviving” mutations in the population. On the contrary, SNP loci are characterized by mutation rates substantially lower with estimates fluctuating between 1.3–3.4 × 10−8 and 10−9 per site per generation [32, 33]. Because of this high evolutionary stability, SNP markers are increasingly important for the study of ancient mutational events associated with human diseases, either combined with STR loci or alone because SNP data may reduce the probability of overestimating the number of founder mutations [22–26, 37].
Specifically in the study on the D178N-129M mutation, Dagvadorj et al. [17] analyzed a set of autosomal microsatellites (STRs) and the SNP PRND/174 in a genomic region of around 3.85 cM in length. Seven kindreds, originating from Italy, US, UK, Austria, Ireland, and France, harboring the haplotype D178N-129M were included in the sample examined. These authors found different STR haplotypes associated to the D178N mutation for each one of the genealogies surveyed, thus concluding that the D178N mutation had been caused by recurrent mutational events (at least seven, according to the number of surveyed families), and therefore, had independent origins in each family. In explaining the inconsistency between the results obtained in the cited study and in the present survey, three main aspects must be taken into account: (1) the much wider geographical scope of the analysis by Dagvadorj et al. [17], which included families from six different countries from two continents, (2) the examination of cases rather than families in this work, and (3) the potential risk of introducing bias in the results by effect of the recombination processes because the microsatellites markers analyzed by those authors are distributed over a relatively long genomic region (3.85 cM).
The present analysis has revealed two distinct SNP haplotypic combinations potentially associated with the D178N-129M mutation in European FFI patients. Of them, GCATTA-M proved to be the common haplotype of the Spanish patients, whereas ACATTA-M was typical of the German FFI cases. In the Italian samples, on the other hand, both of these haplotypes were identified: GCATTA-M in a family from the Tuscany region and ACATTA-M in a family from the Veneto region. It is interesting to note that these two common haplotypes differed in just one SNP marker (rs2756271), whose allelic frequencies were the closest to 0.5 in Spanish general population (A = 0.43, G = 0.57). Some specialists have postulated a correlation between allelic frequencies and mutation age, suggesting that higher frequencies of the different allelic variants for the same SNP imply greater probabilities of an elevated mutation age [40]. In this way, being that these two common haplotypes are the most frequent (and therefore, presumably ancient) and considering also the wide geographical distribution of D178N, it is possible to infer a nonrecent origin for this mutation. The hypothetical antiquity of the mutation would be an additional argument in support of the use of SNPs to explore the origin of the D178N-129M chromosomes.
As previously mentioned, SNP data provide information about the minimal number of “successful” mutations in a given population. Thus, our findings suggest the occurrence of two independent D178N-129M mutational events in Europe, effectively preserved and transmitted between consecutive generations until nowadays. Likewise, analysis of the SNP haplotypes provides evidence indicating the prevalence of the founding events in GCATTA-M and ACATTA-M chromosomes.
Obviously, the results presented herein do not exclude the possibility that other less successful mutational events (i.e., not transmitted between consecutive generations) might have occurred. Furthermore, it is important to consider that a certain number of European FFI cases and of affected individuals misdiagnosed due to the rareness of the disease have not been analyzed in this work. In any case, bearing in mind that our sample involved a high proportion of the European FFI cases, on one hand, and the characteristics of the DNA markers utilized, on the other hand, we believe that the genetic information we provide on the origins of the D178N mutation suggesting the occurrence of two independent mutational events is reliable enough to a European scale. At the same time, findings derived from SNP haplotypic data indicate that the hypothesis postulating that the D178N mutation has independent origins for each family and that the global distribution of such mutation is determined by recurrent mutational events [17] must be regarded with extreme caution. Probably, the true number of founding events will be greater than two (minimal number estimated from SNPs) and less than seven (maximum number estimated from STRs). To clarify this issue, our findings remain open to future revisions with additional molecular DNA data from genomic regions closely linked to the target mutation.
References
Medori R, Tritschler HJ, LeBlanc A, Villare F, Manetto V, Chen HY, Xue R, Leal S, Montagna P, Cortelli P (1992) Fatal familial insomnia, a prion disease with a mutation at codon 178 of the prion protein gene. N Engl J Med 326:444–449
Goldfarb LG, Petersen RB, Tabaton M, Brown P, LeBlanc AC, Montagna P, Cortelli P, Julien J, Vital C, Pendelbury WW (1992) Fatal familial insomnia and familial Creutzfeldt–Jakob disease: disease phenotype determined by a DNA polymorphism. Science 258:806–808
Parchi P, Petersen RB, Chen SG, Autilio-Gambetti L, Capellari S, Monari L, Cortelli P, Montagna P, Lugaresi E, Gambetti P (1998) Molecular pathology of fatal familial insomnia. Brain Pathol 8:539–548
Zarranz JJ, Digon A, Atares B, Rodriguez-Martinez AB, Arce A, Carrera N, Fernandez-Manchola I, Fernandez-Martinez M, Fernandez-Maiztegui C, Forcadas I, Galdos L, Gomez-Esteban JC, Ibanez A, Lezcano E, Lopez dM, Marti-Masso JF, Mendibe MM, Urtasun M, Uterga JM, Saracibar N, Velasco F, de Pancorbo MM (2005) Phenotypic variability in familial prion diseases due to the D178N mutation. J Neurol Neurosurg Psychiatry 76:1491–1496
Zerr I, Giese A, Windl O, Kropp S, Schulz-Schaeffer W, Riedemann C, Skworc K, Bodemer M, Kretzschmar HA, Poser S (1998) Phenotypic variability in fatal familial insomnia (D178N-129M) genotype. Neurology 51:1398–1405
Goldfarb LG (2006) Genotype–phenotype relationships: fatal familial insomnia and Creutzfeldt–Jakob disease. Encyclopedia of Life Sciences, DOI 10.1038/npg.els.0006037
Gambetti P, Parchi P, Petersen RB, Chen SG, Lugaresi E (1995) Fatal familial insomnia and familial Creutzfeldt–Jakob disease: clinical, pathological and molecular features. Brain Pathol 5:43–51
Montagna P, Cortelli P, Avoni P, Tinuper P, Plazzi G, Gallassi R, Portaluppi F, Julien J, Vital C, Delisle MB, Gambetti P, Lugaresi E (1998) Clinical features of fatal familial insomnia: phenotypic variability in relation to a polymorphism at codon 129 of the prion protein gene. Brain Pathol 8:515–520
Johnson MD, Vnencak-Jones CL, McLean MJ (1998) Fatal familial insomnia: clinical and pathologic heterogeneity in genetic half brothers. Neurology 51:1715–1717
McLean CA, Storey E, Gardner RJ, Tannenberg AE, Cervenakova L, Brown P (1997) The D178N (cis-129M) “fatal familial insomnia” mutation associated with diverse clinicopathologic phenotypes in an Australian kindred. Neurology 49:552–558
Taniwaki Y, Hara H, Doh-Ura K, Murakami I, Tashiro H, Yamasaki T, Shigeto H, Arakawa K, Araki E, Yamada T, Iwaki T, Kira J (2000) Familial Creutzfeldt–Jakob disease with D178N-129M mutation of PRNP presenting as cerebellar ataxia without insomnia. J Neurol Neurosurg Psychiatry 68:388
Kovacs GG, Puopolo M, Ladogana A, Pocchiari M, Budka H, van Duijn C, Collins SJ, Boyd A, Giulivi A, Coulthart M, Delasnerie-Laupretre N, Brandel JP, Zerr I, Kretzschmar HA, Pedro-Cuesta J, Calero-Lara M, Glatzel M, Aguzzi A, Bishop M, Knight R, Belay G, Will R, Mitrova E (2005) Genetic prion disease: the EUROCJD experience. Hum Genet 118:166–174
Pocchiari M, Ladogana A, Petraroli R, Cardone F, D’Alessandro M (1998) Recent Italian FFI cases. Brain Pathol 8:564–566
Heinemann U, Krasnianski A, Meissner B, Varges D, Kallenberg K, Schulz-Schaeffer WJ, Steinhoff BJ, Grasbon-Frodl EM, Kretzschmar HA, Zerr I (2007) Creutzfeldt–Jakob disease in Germany: a prospective 12-year surveillance. Brain 130:1350–1359
Rodriguez-Martinez AB, Barreau C, Coupry I, Yague J, Sanchez-Valle R, Galdos-Alcelay L, Ibanez A, Digon A, Fernandez-Manchola I, Goizet C, Castro A, Cuevas N, Alvarez-Alvarez M, de Pancorbo MM, Arveiler B, Zarranz JJ (2005) Ancestral origins of the prion protein gene D178N mutation in the Basque Country. Hum Genet 117:61–69
Lee HS, Sambuughin N, Cervenakova L, Chapman J, Pocchiari M, Litvak S, Qi HY, Budka H, del Ser T, Furukawa H, Brown P, Gajdusek DC, Long JC, Korczyn AD, Goldfarb LG (1999) Ancestral origins and worldwide distribution of the PRNP 200K mutation causing familial Creutzfeldt–Jakob disease. Am J Hum Genet 64:1063–1070
Dagvadorj A, Petersen RB, Lee HS, Cervenakova L, Shatunov A, Budka H, Brown P, Gambetti P, Goldfarb LG (2002) Spontaneous mutations in the prion protein gene causing transmissible spongiform encephalopathy. Ann Neurol 52:355–359
Ramakrishnan U, Mountain JL (2004) Precision and accuracy of divergence time estimates from STR and SNPSTR variation. Mol Biol Evol 21:1960–1971
Campbell C, Mitui M, Eng L, Coutinho G, Thorstenson Y, Gatti RA (2003) ATM mutations on distinct SNP and STR haplotypes in ataxia–telangiectasia patients of differing ethnicities reveal ancestral founder effects. Human Mutat 21:80–85
Sharma D, Gupta M, Thelma BK (2003) FMR1 haplotype analyses among Indians: a weak founder effect and other findings. Hum Genet 112:262–271
Manica A, Prugnolle F, Balloux F (2005) Geography is a better determinant of human genetic differentiation than ethnicity. Hum Genet 118:366–371
Tishkoff SA, Pakstis AJ, Ruano G, Kidd KK (2000) The accuracy of statistical methods for estimation of haplotype frequencies: an example from the CD4 locus. Am J Hum Genet 67:518–522
Tishkoff SA, Pakstis AJ, Stoneking M, Kidd JR, Destro-Bisol G, Sanjantila A, Lu RB, Deinard AS, Sirugo G, Jenkins T, Kidd KK, Clark AG (2000) Short tandem-repeat polymorphism/alu haplotype variation at the PLAT locus: implications for modern human origins. Am J Hum Genet 67:901–925
Rademakers R, Baker M, Gass J, Adamson J, Huey ED, Momeni P, Spina S, Coppola G, Karydas AM, Stewart H, Johnson N, Hsiung GY, Kelley B, Kuntz K, Steinbart E, Wood EM, Yu CE, Josephs K, Sorenson E, Womack KB, Weintraub S, Pickering-Brown SM, Schofield PR, Brooks WS, Van Deerlin VM, Snowden J, Clark CM, Kertesz A, Boylan K, Ghetti B, Neary D, Schellenberg GD, Beach TG, Mesulam M, Mann D, Grafman J, Mackenzie IR, Feldman H, Bird T, Petersen R, Knopman D, Boeve B, Geschwind DH, Miller B, Wszolek Z, Lippa C, Bigio EH, Dickson D, Graff-Radford N, Hutton M (2007) Phenotypic variability associated with progranulin haploinsufficiency in patients with the common 1477C→T (Arg493X) mutation: an international initiative. Lancet Neurol 6:857–868
Scholefield J, Greenberg J (2007) A common SNP haplotype provides molecular proof of a founder effect of Huntington disease linking two South African populations. Eur J Hum Genet 15:590–595
Tsujikawa K, Tsujikawa M, Watanabe H, Maeda N, Inoue Y, Fujikado T, Tano Y (2007) Allelic homogeneity in Avellino corneal dystrophy due to a founder effect. J Hum Genet 52:92–97
Preuss S, Peischl T, Melchinger E, Geldermann H (2004) Numerous polymorphic microsatellites in the human prion gene complex (including PRNP, PRND and PRNT). Gene 329:197–203
McCormack JE, Baybutt HN, Everington D, Will RG, Ironside JW, Manson JC (2002) PRNP contains both intronic and upstream regulatory regions that may influence susceptibility to Creutzfeldt–Jakob Disease. Gene 288:139–146
Mead S, Mahal SP, Beck J, Campbell T, Farrall M, Fisher E, Collinge J (2001) Sporadic—but not variant—Creutzfeldt–Jakob disease is associated with polymorphisms upstream of PRNP exon 1. Am J Hum Genet 69:1225–1235
Guo SW, Thompson EA (1992) Performing the exact test of Hardy–Weinberg proportion for multiple alleles. Biometrics 48:361–372
Excoffier L, Laval G, Schneider S (2005) Arlequin ver. 3. 0: an integrated software package for population genetics data analysis. Evolutionary Bioinformatics Online 1:47–50
Whitfield LS, Sulston JE, Goodfellow PN (1995) Sequence variation of the human Y chromosome. Nature 378:379–380
Nachman MW, Crowell SL (2000) Estimate of the mutation rate per nucleotide in humans. Genetics 156:297–304
Miyakawa T, Inoue K, Iseki E, Kawanishi C, Sugiyama N, Onishi H, Yamada Y, Suzuki K, Iwabuchi K, Kosaka K (1998) Japanese Creutzfeldt–Jakob disease patients exhibiting high incidence of the E200K PRNP mutation and located in the basin of a river. Neurol Res 20:684–688
Colombo R (2000) Age and origin of the PRNP E200K mutation causing familial Creutzfeldt–Jacob disease in Libyan Jews. Am J Hum Genet 67:528–531
Ellegren H (2000) Heterogeneous mutation processes in human microsatellite DNA sequences. Nat Genet 24:400–402
Chakraborty R, Kimmel M, Stivers DN, Davison LJ, Deka R (1997) Relative mutation rates at di-, tri-, and tetranucleotide microsatellite loci. Proc Natl Acad Sci USA 94:1041–1046
Zhivotovsky LA (2001) Estimating divergence time with the use of microsatellite genetic distances: impacts of population growth and gene flow. Mol Biol Evol 18:700–709
Zhivotovsky LA, Rosenberg NA, Feldman MW (2003) Features of evolution and expansion of modern humans, inferred from genomewide microsatellite markers. Am J Hum Genet 72:1171–1186
Stoneking M (2001) Single nucleotide polymorphisms. From the evolutionary past.... Nature 409:821–822
Acknowledgments
The authors are grateful to Prof. P Montagna, P Cortelli, and Dr. P Avoni for providing the clinical data on the Italian case I-02. This study was funded by Research Projects EET-2002/05165 from Ministerio de Educación y Ciencia (Spain), GIU 05/51 from Universidad del País Vasco (UPV/EHU, Spain), and FIS 05/0912 from Fondo de Investigaciones Sanitarias (Spain). AB Rodríguez-Martínez was supported by a doctoral fellowship from the Basque Government (BFI02.1).
Author information
Authors and Affiliations
Corresponding author
Electronic supplementary material
Below is the link to the electronic supplementary material.
Table S1
Age of onset, sex, duration of illness, and clinical features of 38 European FFI cases (DOC 169 kb)
Rights and permissions
About this article
Cite this article
Rodríguez-Martínez, A.B., Alfonso-Sánchez, M.A., Peña, J.A. et al. Molecular evidence of founder effects of fatal familial insomnia through SNP haplotypes around the D178N mutation. Neurogenetics 9, 109–118 (2008). https://doi.org/10.1007/s10048-008-0120-x
Received:
Accepted:
Published:
Issue Date:
DOI: https://doi.org/10.1007/s10048-008-0120-x