Abstract
Attention problems are observed in attention-deficit/hyperactivity disorder (ADHD) and autism spectrum disorder (ASD). Most neuropsychological studies that compared both disorders focused on complex executive functions (EF), but missed to contrast basic attention functions, as well as ASD- and ADHD subtypes. The present study compared EF as well as basic attention functioning of children with the combined subtype (ADHD-C), the predominantly inattentive subtype (ADHD-I), and autism spectrum disorder without ADHD (ASD-) with typically developing controls (TD). Basic attention functions and EF profiles were analysed by testing the comprehensive attention function model of van Zomeren and Brouwer using profile analysis. Additionally, neurocognitive impairments in ASD- and ADHD were regressed on dimensional measures of attention- and hyperactive-impulsive symptoms across and within groups. ADHD-C revealed a strong impairment across measures of EF compared to ASD- and TD. The ADHD-C profile furthermore showed disorder specific impairments in interference control, whereas the ASD- profile showed a disorder specific impairment in basic attention component divided attention. Attention- and hyperactive-impulsive symptom severity did not predict neurocognitive impairments across- or within groups. Study findings thus support disorder and subtype specific attention/EF profiles, which refute the idea of a continuum of ADHD-I, ADHD-C, and ASD with increasing neurocognitive impairments.
Similar content being viewed by others
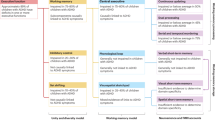
Avoid common mistakes on your manuscript.
Introduction
Autism spectrum disorder (ASD) as well as the predominantly inattentive and combined subtypes of attention-deficit/hyperactivity disorder (ADHD-I, ADHD-C) are early onset neuro-developmental disorders of childhood. ASD and ADHD share common genetic, but also disorder-specific genetic and environmental risk factors [1, 2]. In ASD, parent- and teacher ratings show a high rate of inattentive behaviour and attention problems [3,4,5], even if children with ASD do not fulfill diagnostic criteria for ADHD [6]. Thus, ASD, ADHD-I and ADHD-C are all characterized by high rates of clinically observed attention problems across diagnostic boundaries. Impairments in neurocognitive functions have been postulated to underlie inattentive behaviour and attention problems. Indeed, behavioural ratings of inattentive behaviour and attention problems predict neurocognitive impairments in children with ADHD [7,8,9]. Several studies have also shown neurocognitive impairments in children with ASD, but often studies included children with ASD and co-morbid ADHD [see for instance 10–12], and focused on executive functions (EF) rather than basic attention functions [13, 14]. Comparing neurocognitive profiles of basic attention functions in addition to EF, next to correlating inattentive and hyperactive-impulsive symptoms with shared and disorder-specific neurocognitive impairments, can therefore provide more insight into the specificity of neurocognitive impairments in ADHD-I, ADHD-C and ASD without ADHD (ASD-).
Various test batteries have been designed to measure neurocognitive functioning. To interpret performance on such tests correctly, several factors need to be considered. Attention and EF functions are defined as interdependent constructs, which implies that the quality of performance on a task specifically designed to measure a particular aspect of attention inevitably also depends on aspects of cognitive functioning; a problem referred to as task impurity [15]. Additionally, generalizing performance across tasks that target the same measurement objective (i.e. sustained attention or cognitive flexibility) may be compromised when such tasks differ in one or several important task parameters, such as task-demand, or stimulus-modality [16, 17]. Finally, even when the same test is used to measure performance, different- or additional methods to calculate outcome variables may lead to divergent interpretations of study results [18, 19].
Van Zomeren and Brouwer [20] postulated a multi-component attention model based on Posner and Rafal [21] that includes basic attention- as well as EF. The Tests of Attentional Performance battery (TAP) [20] was specifically designed to measure various basic attention- and EF components. In the model underlying TAP basic attention functions are subdivided into dimensions of attention intensity—and selectivity. Both dimensions are broken down into several attention subcomponents, which are described in more detail below. The “supervisory attention system” (SAS) [22] monitors dimensions of intensity and selectivity and comprises EF abilities flexibility and inhibition. To examine the neurocognitive profiles of basic attention functioning in addition to EF and to systematically address task impurities and differences in task designs and outcome variables across studies, the present study specifically focused on testing the multi-component model of van Zomeren and Brouwer [20] using the TAP.
Intensity of attention: alertness and sustained attention
The dimension attention intensity is composed of the attention components alertness and sustained attention. Alertness is further divided into two subcategories, tonic and phasic alertness. While tonic alertness refers to the state of wakefulness, which fluctuates mildly and slowly during the day, phasic alertness refers to the ability to immediately increase attention following an alerting stimulus. Some studies examined tonic and phasic alertness performance within each task, whereas other studies considered the difference in performance as main outcome. For both children with ADHD-C, ADHD-I, impairments in tonic as compared to phasic alertness have been reported. More specifically, when performance was collapsed within phasic alertness conditions, children with ADHD performed no different from TD [23, 24]. When tonic alertness was measured by the difference in performance between tonic and phasic conditions, alerting cues were more effective in increasing alertness for children with ADHD-C and ADHD-I as compared to typically developing controls (TD) [25, 26]. In contrast, children with ASD- seemed to benefit to the same extent as healthy controls from alerting cues [26, 27] and in addition did not show impairments across tonic and phasic alertness tasks [12].
Sustained attention comprises the capability to maintain a constant level of attention to one or more sources over a relatively long period of time [20]. Consequently, tasks that measure sustained attention are traditionally tasks that require maintaining the focus of attention over a longer time period [20]. Alternative ways to measure sustained attention is to study the differential decline over time in task performance during one [28] or across various tasks [29]. In studies using the continuous performance test (CPT) to study sustained attention, strong effects have been found for impairments in children with ADHD in overall task performance, and small to moderate effects for a decline of performance over time [30]. Equivalently, compared to children with ASD, children with ADHD showed impairments in overall task performance [11, 31] as well as a differential decline of performance over time [11]. Several studies that reported impaired sustained attention in children with ASD compared to TD did not control for co-morbid ADHD [10, 12].
Selectivity of attention: focused- and divided attention
The dimension selectivity of attention comprises attention components focused- and divided attention, which are considered to be most closely related to SAS components inhibition and flexibility [20]. Focused attention is defined as the ability to concentrate selectively on specific aspects of information while suppressing others. Focused attention abilities of children with ADHD and ASD were intact when simple task stimuli were used [12, 32]. In contrast, when visual complexity of these stimuli increased, children with ADHD and ASD showed impaired focused attention. More specifically, children with ADHD-C showed higher rates of omission errors [24] and children with ASD showed slower reaction times than TD [12]. None of these studies controlled for ADHD symptoms in ASD.
Divided attention refers to the ability to focus on two or multiple aspects in the environment at once. Tasks that measure divided attention require participants to process several aspects of information in parallel. Some tasks instruct participants to divide their attention between aspects of the environment periodically, reflecting a relatively more top-down controlled activity (e.g. Trail Making Test-B (TMT-B); Children’s Color Trails Test 2 (CCTT-2)). Other tasks require participants to divide their attention in response to target stimuli, reflecting a relatively more stimulus-driven activity (e.g. TAP-Divided Attention).
Across studies strong impairments for ASD- in top-down divided attention have been reported [14]. Impaired performance of top-down divided attention has been reported as well for children with ADHD [9, 33, 34], although not consistently [32], possibly due to subtype specific impairments for ADHD-C compared to ADHD-I [9]. As for stimulus-driven divided attention contrasting results have been reported for children with ADHD [24, 31, 35] as well as ASD- [31, 36]. Direct comparisons of stimulus-driven divided attention abilities between ASD- and ADHD did not reveal a differential impairment [31]. To date, no data on differential impairment of ADHD-C compared to ADHD-I in stimulus-driven attention is available, and no comparison to ASD- has been performed.
Supervisory attentional system (SAS)
The SAS comprises two cognitive functions, inhibition and flexibility, that monitor the basic attention functions [20]. Inhibition is the ability to withhold, stop or slow down behaviour. Tasks that measure inhibition can be broadly divided into tasks measuring interference control, the ability to stop an ongoing response, and impulsivity. Interference control tasks require participants to suppress an automatic or prepotent response. In tasks instructing participants to stop an ongoing response target stimuli are presented, occasionally followed by auditory or visual stop signals, (go-no-go tasks; the stop-signal paradigm, see [16] for an overview). Finally, impulsivity refers to commission errors during attention tasks [14, 32, 34].
For children with ADHD across studies medium effects have been reported for impairments in stopping an ongoing response [16] as well as impulsivity [32, 34]. Small to medium effects have been reported for an ADHD specific impairment in interference control [25, 37, 38], but findings were not consistent [10, 26]. Studies differentiating between ADHD-C and ADHD-I report a specific impairment of interference control for ADHD-C [9, 33]. Impairments in interference control have also been reported for ASD [10,11,12], but without accounting for the influence co-morbid ADHD symptoms. When the influence of co-morbid ADHD symptoms is controlled for, ASD- performance across various inhibition tasks has found to be unimpaired [14].
Cognitive flexibility implies a switch in the focus of attention on a perceptual, cognitive or response level in order to respond to the environment in different ways [20]. Tasks that measure cognitive flexibility include measures of spatial shifting of attention (re-orienting), cross-modality shifting, and (cognitive) set shifting. Furthermore, cross modality and set shifting tasks may differ in task instruction: in explicit flexibility tasks the participant is instructed to shift focus explicitly, in implicit flexibility tasks there is no such instruction (i.e. the participant needs to initiate the shift spontaneously in order to succeed).
Children with ADHD re-orient their attention comparable to TD [26, 39]. Only small effects for re-orienting impairments in children with ASD have been reported [40], and, accordingly, no differential impairment in re-orienting efficiency has been reported for ASD- as compared to ADHD [26]. Studies testing explicit flexibility task performance in ADHD reported divergent results [10, 24, 41]. For children with ASD a slower performance [12] as well as more errors compared to TD have been reported [10, 12]. Whether explicit flexibility is differentially impaired in ASD compared to ADHD is not clear, as the influence of co-morbid ADHD or subclinical ADHD-symptoms was not controlled, even in the studies directly comparing explicit flexibility task performance between ASD and ADHD [10, 41]. The influence of co-morbid ADHD in ASD on implicit flexibility abilities has been studied more frequently. That is, across studies children with ASD- showed specific impairments in implicit flexibility compared to TD [14], as well as compared to ADHD- [13].
Taken together, impairments in basic attention functions and EF for ADHD-C, ADHD-I, as well as ASD- were observed in comparison to TD. However, direct comparisons of ADHD-C, ADHD-I with ASD- are clearly needed to obtain more insight into the specificity of these impairments, i.e. ADHD-subtype specific impairments as well as ASD– specific impairments. The first aim of the present study was therefore to compare the basic attention and EF profiles of children with ADHD-C, ADHD-I, and ASD- based on the multi-component attention model of van Zomeren and Brouwer [20]. The second aim was to explore whether attention problems and hyperactive-impulsive behaviour across and within groups differentially predicted neurocognitive impairments in children with ASD- as compared to in ADHD-I and ADHD-C. First, we hypothesized that the attention profile of children with ADHD-C, ADHD-I and ASD- revealed an overall worse performance than TD across measures of basic attention function (i.e. tonic alertness, phasic alertness, focused attention, stimulus-driven divided attention) and both SAS components (interference control, impulsivity, re-orienting attention, explicit flexibility). We expected that the performance of children with ADHD-C, ADHD-I and ASD- across basic attention and SAS components significantly differed from TD. Furthermore, we expected that children with ADHD-C and ADHD-I showed relatively more impaired basic attention profiles compared to ASD-. In addition, we expected ADHD symptoms to predict neurocognitive impairments in ADHD-C and ADHD- as well as ASD-.
Methods
Participants
Four samples of children were included (ASD-, ADHD-I, ADHD-C, TD), aged 6.5–16.5 years. All subjects were male and had a full scale IQ above 75. The groups were matched for age. Average IQ was higher in TD than in ADHD-I and ADHD-C, F = 4.495, df = (3, 122), p < 0.01. Sample characteristics are presented in Table 1. Exclusion criteria for all groups were obsessive–compulsive and depressive disorder, epilepsy, fragile X syndrome, dyslexia, dyscalculia, visual or hearing impairments, premature birth and birth weight less than 2000 g. All children who received psychopharmacological treatment with stimulants were asked to withdraw medication 24 h (immediate release) respectively 48 h (extended release) prior to testing. No other medication was allowed. Children were recruited and tested in the University Hospital Departments of Child and Adolescent Psychiatry, Psychosomatics and Psychotherapy in Frankfurt and Homburg, Germany. The study was approved by the local ethical committees and was done in accordance with the 1964 Helsinki declaration and its later amendments or comparable ethical standards. Informed consent was obtained from all individual participants and their parents prior to inclusion in the study.
ASD group
Twenty-three children with ASD aged 6.8–16.5 years old (M = 10.0 years, SD = 2.2 years) with a mean IQ of 104.9 (SD = 13.0) participated. Nineteen were diagnosed with High Functioning Autism, 4 with Asperger’s Disorder, and one with pervasive developmental disorder—not otherwise specified. The diagnosis was made by an experienced clinical psychologist or psychiatrist using the gold standard instruments Autism Diagnostic Observation Schedule (ADOS [42] and autism diagnostic interview-revised (ADI-R [43]), and was based on DSM-IV-TR criteria [44]. Only children who met diagnostic criteria of ASD in both ADOS and ADI-R were included in this sample. Parents were additionally asked to complete the Child Behaviour Checklist (CBCL) [45]. ADHD-I or ADHD-C were excluded in the ASD group by standard clinical assessment and specific ADHD rating (see below).
ADHD-I group
In the ADHD-I group, 23 children aged 7.0–13.4 years old (M = 9.9, SD = 1.8) with an average IQ of 98.2 (SD = 12.2) were included. The diagnosis of ADHD-I was made using the structured Diagnostic Interview for Children and Adolescents, parent version (Kinder-DIPS [46]). On the basis of this interview, a German DSM-IV TR based diagnosis checklist, the DCL-ADHD [47] was completed to establish criteria for ADHD-I. Additionally, parents completed the CBCL. ASD was excluded by standard clinical assessment by an experienced clinical psychologist or psychiatrist as well as screening by the Social Communication Questionnaire [48].
ADHD-C group
Similarly to the diagnostic procedure of the ADHD-I group, the Kinder-DIPS and DCL-ADHD was administered to establish a diagnosis of ADHD-C. Parents also completed the CBCL. This sample included 58 children aged 6.5–15.3 years old (M = 9.7, SD = 1.9) with an average IQ of 102.3 (SD = 11.2). ASD was excluded by standard clinical assessment by an experienced clinical psychologist or psychiatrist as well as screening by the Social Communication Questionnaire [48].
TD group
Twenty-two TD children, aged 6.7–15.2 years (M = 9.8, SD = 2.3) with a mean IQ of 112.0 (SD = 13.0) were included. TD had no psychiatric history and showed T-scores below 67 on all subscales of the CBCL [45]. Means and SD all questionnaires scales and subscales are presented in Table 1.
Measures
All participants completed six subtests of the computerized test battery TAP (Tests of Attentional Performance) version 2.1 [49].
Intensity of attention: alertness and sustained attention
The alertness task consists of two parts. In the ‘tonic alertness’ condition, the participant is required to respond to a visual stimulus (‘X’ presented on the screen) by pressing a button. In the ‘phasic alertness’ condition, an acoustic stimulus (warning tone) appears prior to the presentation of the visual stimulus. The time span between the warning tone and the appearance of the stimulus was random (between 300 and 700 ms). The conditions are presented in an ABBA-design (A = tonic alertness, B = phasic alertness). Measures of tonic alertness comprised the mean reaction time (RT) and variability in reaction time (RTV; standard deviation of the mean) during the tonic alertness condition. Measures of phasic alertness were calculated as the difference in reaction time between the phasic- and tonic alertness condition.
Sustained attention Due to technical difficulties, we were not able to obtain sufficient measures of sustained attention for meaningful statistical analysis.
Selectivity of attention: focused- and divided attention
The go/no-go task requires the participant to select two patterns out of five by pressing the response button. During the task, one pattern at a time is presented in the center of the screen and the participant has to decide whether it refers to the two target stimuli. The dependent measures are RT, RTV, and omission errors (focused attention).
In the divided attention task a 4 × 4 matrix is displayed on the screen, in which crosses continuously change their position,. The participant has to press a button whenever the crosses form a square. Simultaneously an alternating sequence of low and high sounds is presented to the participant. In the case of inconsistency in this order, the participant has to press the button. Total amount of commission and omission errors, mean RT and RTV measures were calculated (divided attention).
Supervisory attentional system (SAS)
In the covert shift of attention task the participant has to focus a fixation point in the center of the screen. Then an arrow (= cue stimulus) appears that indicates the expected side of a cross (= target stimulus). For 80% of the trials this cue was valid. The participant is instructed to press the response button when the cross displays. RT, RTV and commission errors across both conditions were calculated (re-orienting of attention).
Commission errors of the go/no-go task (described above) were used to measure impulsivity.
In the incompatibility task the participant is instructed to fixate on a central point on the screen. During the task, arrows pointing either to the left or right appear on either the left or right side of the screen. The participant is asked to only react to the direction the arrow is pointing. During compatible trials, pointing direction and location of appearance are the same; during incompatible trials these parameters differ. RT, RTV, and incompatible false reactions were calculated (interference control).
In the flexibility task two buttons are allocated in the front of the participant, corresponding to the respectively left and right side of the computer screen. During the task, stimuli from two competing shape categories (angled and round) are presented simultaneously. The participant has to respond alternately to the location of the respectively angled or the round stimuli on the screen. RT, RTV and false reactions were calculated (explicit cognitive flexibility).
ADHD symptom severity
Severity of ADHD symptoms for all children were obtained using the Diagnostic Checklist for Attention Deficit Hyperactivity Disorder (DCL-ADHD) and the ADHD rating scale for parents (FBB-ADHD). The DCL-ADHD and the FBB-ADHD are disorder-specific questionnaires from the DISYPS-KJ based on DSM-IV-TR and ICD-10 criteria with each item measuring ADHD symptom severity on a 3-point scale [47]. The Inattention- and Hyperactivity/Impulsivity symptom domain scores show good factorial validity as well as internal consistency (Cronbach’s α respectively 0.88 and 0.84) [50]. The DCL-ADHD is the expert version of the FBB-ADHD and was rated by a trained clinician based on information from the Kinder-DIPS. For 11 children in the control group and 7 children in the ASD- group DCL-ADHD data was missing; thus FBB-ADHD data was recoded to obtain the same measures of ADHD symptom severity for all cases.
Procedure
All tasks were completed in a small and quiet room, and were administered in a fixed order (i.e. alertness task, flexibility task, divided attention task, Go/Nogo task, incompatibility task, covert shift of attention task). The participants were instructed verbally according to testing standard by the administrators. Additionally, a short introduction of the test procedure was presented on the monitor. The session lasted 60–70 min.
Statistics
Statistical analysis was carried out using IBM SPSS windows version 22 [51]. Profile analysis was used to compare neurocognitive attention- and EF profiles between groups. Group differences in the overall level of attention and EF functioning in terms of overall level of impairment (between groups effect) and group-specific impairment (within group/between group interaction effects) were tested using repeated measures. Separate analyses were conducted for basic attention functions and SAS components, as well as for reaction times, standard deviations and error rates. When groups performed significantly different across tasks (i.e. before correction for multiple comparisons), additional ANCOVAs were conducted to test for group-specific impairments. Group differences in phasic alertness were calculated separately, as phasic alertness performance was calculated by differences in mean RT between tonic and phasic alertness conditions. The effect of age on neurocognitive functioning was controlled for by including age as covariate in all models. To obtain an estimate of the influence of IQ on neurocognitive performance, sensitivity analysis was added by re-running models showing group differences by including IQ as additional covariate. False Discovery Rate (FDR) was used to account for multiple comparisons [52].
To explore the relation between attention- and hyperactivity/impulsivity symptom domain scores and neurocognitive impairments, symptom domain scores, clinical group, and interaction term for symptoms scores and clinical group were entered as predictors in linear regression models with measures of neurocognitive performance as dependent variables. Age was controlled for as covariate in the model.
Results
Results are presented across measures of basic attention- and SAS components (for all relevant parameters regarding basic attention components and SAS components see respectively Tables 2 and 3).
Differences in basic attention profiles between ADHD-C, ADHD-I, ASD- and TD
Children with ADHD-C, ADHD-I, ASD- and TD did not differ in the overall mean reaction time (RT) across measures of attention intensity (tonic alertness) and attention selectivity (divided attention, focused attention), F = 0.333, df = (3, 121), p = 0.801, pc = 0.801. Furthermore, no group differences were observed in mean RT for any of the basic attention functions, Pillai’s Trace = 0.219, F = 1.389, df = (6, 242), p = 0.219, pc = 0.270. The overall SD of RT across measures of attention intensity (phasic alertness), attention selectivity (divided attention, focused attention) was not different between groups, F = 1.340, df = (3, 121), p = 0.265, pc = 0.316 and groups also showed no specific differences in SD for any of the basic attention functions, Pillai’s Trace = 0.038, F = 0.771, df = (6, 242), p = 0.593, pc = 0.627. Groups did also not differ in the overall amount of false reactions across measures of attention selectivity (divided attention, selective attention), F = 1.050, df = (3, 121), p = 0.373, pc = 0.418, but marginal group differences were found for specific measures of attention selectivity (Fig. 1: within group/between group interaction effect: Pillai’s Trace = 0.103, F = 2.179, df = (6, 242), p = 0.046, pc = 0.077; including IQ: Pillai’s Trace = 0.122, F = 2.592, df = (6, 240), p = 0.019). More specifically, groups differed in the amount of omission errors as measure of divided attention, F = 3.752, df = (3, 121), p = 0.013, pc = 0.034, η 2 p = 0.085, but not in the amount of comission errors as a measure of divided attention, F = 0.926, df = (3, 121) p = 0.430, pc = 0.468, nor omission errors as a measure of focused attention, F = 1.217, df = (3, 121), p = 0.307, pc = 0.355. Children with ASD- made more omission errors compared to TD (p = 0.013, pc = 0.034), ADHD-C (p = 0.001, pc = 0.004), but only a trend for differences compared to ADHD-I (p = 0.050, pc = 0.079) was found. Finally, no group differences were found for the difference in mean RT between the phasic- and tonic alertness conditions, F = 0.418, df = (3, 121), p = 0.740, pc = 0.761).
To summarize, children with ADHD-C, ADHD-I, and ASD- did not show an overall worse performance than TD across measures of basic attention functions. In addition, no differential impairments for basic attention functions were found for the ADHD subtypes, but instead for ASD- compared to ADHD-C.
Differences in Supervisory Attention System (SAS) profiles between ADHD-C, ADHD-I, ASD- and TD
Children with ADHD-C, ADHD-I, ASD- and TD differed only marginally in the overall mean RT across measures of SAS components re-orienting attention, interference control and explicit flexibility (mean RT: F = 2.853, df = (3, 121), p = 0.040, pc = 0.070), but mean RT differed significantly between groups for specific SAS components (Fig. 2; within group/between group interaction effect: Pillai’s Trace = 0.127, F = 2.743, df = (6, 242), p = 0.013, pc = 0.034; including IQ: Pillai’s Trace = 0.124, F = 2.625, df = (6, 240), p = 0.017). When compared on the level of the specific tasks, however, neither group differences in mean RT for SAS components re-orienting attention, interference control and explicit flexibility reached statistical significance, F = 1.755, df = (3, 121), p = 0.159, pc = 0.203, F = 2.608, df = (3, 121), p = 0.055, pc = 0.081; F = 2.524, df = (3, 121), p = 0.061, pc = 0.087).
Groups did not differ in the overall SD across measures of SAS components re-orienting attention, interference control and explicit flexibility (F = 2.668, df = (3, 121), p = 0.051, pc = 0.079). In contrast, SD differed significantly between groups for specific SAS components (Fig. 3; within group/between group interaction effect: Pillai’s Trace = 0.129, F = 2.773, df = (6, 242), p = 0.013, pc = 0.034; including IQ: Pillai’s Trace = 0.126, F = 2.698, df = (6, 240), p = 0.015). Group differences for the SD of the RT measuring re-orienting attention (F = 3.206, df = (3, 121), p = 0.026, pc = 0.064), interference control (F = 3.117, df = (3, 121), p = 0.029, pc = 0.065, and explicit flexibility (F = 3.006, df = (3, 121), p = 0.033 pc = 0.066) did not withhold correction for multiple testing.
Groups significantly differed in the overall amount of false reactions across measures of SAS components re-orienting attention, impulsivity, interference control and explicit flexibility (Fig. 4; F = 8.704, df = (3, 121), p = 0.000, pc = 0.000, η 2 p = 0.177, also when IQ was included as covariate F = 7.143, df = (3, 121), p = 0.000). Children with ADHD-C showed a higher amount of false reactions across measures of SAS components than TD (p = 0.000, pc = 0.000) and ASD- (p = 0.002, pc = 0.007), but only a trend towards differences compared to ADHD-I (p = 0.03, pc = 0.065). Specific group effects were found for SAS-components re-orienting attention (F = 8.785, df = (3, 121), p = 0.000, pc = 0.000, η 2 p = 0.179), interference control (F = 5.532, df = (3, 121), p = 0.001, pc = 0.004, η 2 p = 0.120). Group differences for for explicit flexibility did not withold correction for multiple testing (F = 2.847, df = (3, 121), p = 0.040, pc = 0.070). Impulsivity (F = 2.125, df = (3, 121), p = 0.101, pc = 0.133) did not differ across groups (Fig. 4).
For SAS component re-orienting attention, ADHD-C specific impairments were found: children with ADHD-C made more comission errors compared to TD (p = 0.000, pc = 0.000), ASD- (p = 0.000, pc = 0.000), as well as ADHD-I (p = 0.000, pc = 0.000). In interference control children with ADHD-C differed significantly from TD (p = 0.000, pc = 0.000), but only a marginally significant difference to ADHD-I (p = 0.034 pc = 0.066) and no difference to ASD- (p = 0.077, pc = 0.106) was observed.
To summarize, the SAS profile of ADHD-C showed a relatively more severe overall level of impairments compared to ASD- and TD, characterized by a higher overall rate of false reactions, as well as SAS-specific impairments.
Dimensional ADHD symptom scores and impairments in basic attention and SAS components
To evaluate whether ADHD symptoms predict neurocognitive impairments in ADHD-C, ADHD-I as well as ASD-, neurocognitive impairments for ADHD or ASD- were regressed on dimensional symptom scores of the inattention- and hyperactivity/impulsivity domain, clinical group and an interaction term for clinial group and dimension sympom scores.
An ASD-specific impairment was found for basic attention component divided attention (omission errors). Neither dimensional inattention symptoms across groups or within ASD predicted measures of divided attention (inattention; βSD = 0.028, t(125) = 0.308, p = 0.768, inattention*group; βSD = − 0.145, t(125) = − 0.718, p = 0.474). Dimensional hyperactivity/impulsivity symptoms across groups or within ASD did also not predict measures of divided attention (hyperactivity/impulsivity; βSD = − 0.068, t(125) = − 0.747, p = 0.456; hyperactivity/impulsivity*group; βSD = − 0.245, t(125) = − 1.915 p = 0.057).
The highest effect-size for an ADHD-C specific was found for SAS component re-orienting attention (comission errors). Inattention did not predict comission errors as a measure of re-orienting attention (βSD = − 0.011, t(125) = − 0.088, p = 0.930), and the effect of inattention symptom severity on the rate of comission errors did not differ between groups (inattention*group; βSD = 0.073, t(125) = 0.188, p = 0.851. Differences in severity of hyperactivity/impulsivity symptoms neither predictedct the rate of comission errors as a measure of re-orienting attention across- or within a specfic group (hyperactivity/impulsivity; βSD = 0.148, t(125) = − 0.598, p = 0.551, hyperactivity/impulsivity*group; βSD = − 0.252, t(125) = − 0.560 p = 0.567).
Discussion
Our first study aim was to compare the basic attention and EF profiles of children with ADHD-C, ADHD-I, and ASD- by testing the comprehensive multicomponent attention model of van Zomeren and Brouwer [20]. Against our predictions, the attention profile of children with ADHD-C, ADHD-I and ASD- did not reveal an overall worse performance than TD across measures of basic attention functions (i.e. tonic alertness, phasic alertness, focused attention, stimulus-driven divided attention). However, across measures of SAS components (interference control, impulsivity, re-orienting attention, explicit flexibility), the profile of ADHD-C revealed a strong impairment compared to ASD- and TD, less so to ADHD-I. Furthermore, an ASD- specific impairment for the basic attention component divided attention was found, as well as ADHD-C specific impairments of SAS components interference control and re-orienting attention. Effects for specific impairments were medium- to large sized, and a large effect was found (i.e. η 2 p = 0.177) for the overall impairment of ADHD-C across SAS-components. Increased commission errors in different motor and cognitive inhibition tasks are a well replicated finding in children with ADHD-C [53, 54], and the results of the present study confirm these findings.
The specific impairments for ASD- in stimulus-driven divided attention is in line with previously reported ASD-specific impairments in impaired divided attention across modalities [31, 55], and the well replicated impaired visuo-spatial and visuo-motor processing abilities in ASD [56], as the divided attention task relies on a fast and correct allocation of a specific spatial position in the visual field. This divided attention deficit may also underlie reduced joint attention, which is a core deficit in ASD [57].
The specific impairments reported for ADHD-C in interference control are partly supported by previously reported small to medium effects [25, 37, 38], but see [10, 26]. Subtype-specific differences for ADHD-C in interference control compared to ADHD-I have been reported previously [9, 33]. In addition, specific impairments in interference control have been reported for ASD [10,11,12]. In contrast to previous studies, the present study controlled for co-morbid ADHD in ASD and differentiated between ADHD subtypes. Given the direction of the effects in the context of low power, our results therefore provide support for a differential impairment of interference control for ADHD-C. Finally, a large-sized ADHD-C specific impairment was found for re-orienting attention as measured by commission errors on a covert attention task, which is not supported by the literature [26, 39]. Since tasks were administered in a fixed order, with the covert attention task being the last task, the impaired performance may in fact reflect impaired performance in sustained attention rather than in re-orienting attention. ADHD specific impairments in sustained attention have been described by many studies, in children and adolescents [11, 30, 31, 58]. Taken together, findings suggest that attention impairments in ADHD-C are not limited to SAS-components, but are complemented by impairments in the basic attention component sustained attention.
Limitations of our study firstly concern the relatively simple computer-based tests that were applied to measure the various attention components and EF, which may affect generalisation to everyday situations. Next to the fixed administration order, another limitation was the small sample size and consequently low power, which did not allow testing for small and medium effects. Although power was sufficient to interpret group differences, as we have detected large effects, small to medium effect size differences between groups may have remained undetected and can thus not be excluded. In contrast, power was sufficient to detect a small to medium effect of a single regression component. Thus, the lack of association of dimensional measures of inattention and hyperactivity-impulsivity beyond group effects emphasizes the importance of specific basic attention and EF impairments in ADHD-C, ADHD-I and ASD-. Previous studies did find ADHD symptom severity to be associated with neurocognitive performance across ADHD and ASD samples [10, 59], and within ADHD [7,8,9] and ASD samples [60, 61]. Some of those studies used composite scores derived from multiple neurocognitive measures instead of single neurocognitive measures to control for the specific influence of each task/cognitive subdomain [9, 60]. In addition, the majority of these studies did not include group status in the analysis [7, 8, 59, 60]. The effects between ADHD symptom severity and neurocognitive performance may consequently not have extended beyond group status.
Another limitation is the IQ difference between TD and both ADHD groups. This reflects the typical IQ distribution in ADHD, which is often below TD samples [62] and therefore corroborates the classification of our groups. Furthermore, since results did not change when IQ was included as covariate, one can assume that the IQ differences did not influence study results. Finally, only male participants were included in the analysis. In future research girls should be brought into focus in order to analyse possible gender effects on attention performance in ASD and ADHD.
In summary, ADHD-C showed disorder specific impairments across SAS tasks and specific impairments in interference control. Impairments in basic attention functions were not found for both ADHD subtypes, but impairments for sustained attention in ADHD-C cannot be excluded due to limitations in study design. The attention/EF profile of ASD- showed impairments in the basic attention component divided attention. Overall, study findings support disorder and subtype specific attention profiles, which refute the idea of a continuum of ADHD-I, ADHD-C, and ASD with increasing cognitive impairments [6], at least in the area of the neuropsychology of attention. Based on twin studies, it has been reported that common genetic factors [63, 64] underlie the phenotypic overlap between ASD and ADHD [65]. Thus, the hypothesis that ASD and ADHD represent alternate expressions of an underlying continuum has been proposed, with ADHD as a less severely impaired subtype within the ASD spectrum [1]. Statistical approaches that allow to compare whether a particular disorder represent a discrete entity, or one end of a continuum, point towards ASD as a discrete entity, but ADHD as a continuous trait [66]. Across diagnostic borders of ADHD and ASD, however, support for an underlying continuum in terms of behavioral symptoms, as well as for the degree of (cognitive) impairment has been reported [6]. In the present study, the ADHD sample showed elevated parent reported social problems compared to TD, but not to ASD-, and the ASD- sample showed elevated parent reported attention problems compared to TD (see Table 1). In accordance with the overarching disorder hypothesis, ASD- should have shown the most severe impairments relative to ADHD-C and ADHD-I. In contrast, our results clearly demonstrated the ‘pure’ ADHD-C subtype to be most severely impaired on a neurocognitive level. Additionally, significantly higher CBCL total scores for ADHD-C compared to ASD- (see Table 1) indicate a higher overall level of psychiatric and behavioral symptoms for ADHD-C relative to ASD-. The disorder-specific impairments for ADHD-C and ASD- found in the present study furthermore support qualitative, rather than quantitative differences in neurocognitive profiles between disorders. Thus, even though our study did not statistically examine whether neurocognitive functions and symptom data across diagnostic borders of ADHD and ASD represent a discrete entity or a continuum, our results do argue against the idea of a continuum of ADHD-I, ADHD-C and ASD with increasing cognitive impairments in ASD. Accurate classifications of differential and overlapping impairments in attention functioning could support the search for shared and non-shared genetic mechanisms involved in the onset and susceptibility to develop ADHD or ASD-. Furthermore, comparing cognitive profiles between disorders in addition to research on ‘central deficits’ in ADHD and ASD- not only broadens our knowledge on the clinical manifestation of disorders, but may in addition lead to insights that may be directly applied in clinical practice.
References
Rommelse NNJ, Geurts HM, Franke B, Buitelaar JK, Hartman CA (2011) A review on cognitive and brain endophenotypes that may be common in autism spectrum disorder and attention-deficit/hyperactivity disorder and facilitate the search for pleiotropic genes. Neurosci Biobehav Rev 35:1363–1396. https://doi.org/10.1007/s00787-010-0092-x
Taurines R, Schwenck C, Westerwald E, Sachse M, Siniatchkin M, Freitag C (2012) ADHD and autism: differential diagnosis or overlapping traits? A selective review. ADHD Atten Def Hyp Disord 4:115–139. https://doi.org/10.1007/s12402-012-0086-2
Goldstein S, Schwebach AJ (2004) The comorbidity of pervasive developmental disorder and attention deficit hyperactivity disorder: results of a retrospective chart review. J Autism Dev Disord 34:329–339. https://doi.org/10.1023/B:JADD.0000029554.46570.68
Leyfer OT, Folstein SE, Bacalman S, Davis NO, Dinh E, Morgan J, Tager-Flusberg H, Lainhart JE (2006) Comorbid psychiatric disorders in children with autism: interview development and rates of disorders. J Autism Dev Disord 36:849–861. https://doi.org/10.1007/s10803-006-0123-0
Sinzig J, Walter D, Doepfner M (2009) Attention deficit/hyperactivity disorder in children and adolescents with autism spectrum disorder: symptom or syndrome? J Atten Disord 13:117–126. https://doi.org/10.1177/1087054708326261
van der Meer JM, Oerlemans AM, van Steijn DJ, Lappenschaar MG, de Sonneville LM, Buitelaar JK, Rommelse NN (2012) Are autism spectrum disorder and attention-deficit/hyperactivity disorder different manifestations of one overarching disorder? Cognitive and symptom evidence from a clinical and population-based sample. J Am Acad Child Adolesc Psychiatry 51:1160–1172. https://doi.org/10.1016/j.jaac.2012.08.024
Chhabildas N, Pennington BF, Willcut EG (2001) A comparison of the neuropsychological profiles of the DSM-IV subtypes of ADHD. J Abnorm Child Psychol 29:529–540
Huang-Pollock CL, Mikami AY, Pfiffner L, McBurnett K (2007) ADHD subtype differences in motivational responsivity but not inhibitory control: evidence from a reward-based variation of the stop signal paradigm. J Clin Child Adolesc Psychol 36:127–136. https://doi.org/10.1080/15374410701274124
Nikolas MA, Nigg JT (2013) Neuropsychological performance and attention-deficit hyperactivity disorder subtypes and symptom dimensions. Neuropsychology 27:107–120. https://doi.org/10.1037/a0030685
Corbett BA, Constantine LJ, Hendren R, Rocke D, Ozonoff S (2009) Examining executive functioning in children with autism spectrum disorder, attention deficit hyperactivity disorder and typical development. Psychiatr Res 166:210–222. https://doi.org/10.1016/j.psychres.2008.02.005
Johnson KA, Robertson IH, Kelly SP, Silk TJ, Barry E, Dáibhis A, Watchorn A, Keavy M, Fitzgerald M, Gallagher L, Gill M, Bellgrove MA (2007) Dissociation in performance of children with ADHD and high-functioning autism on a task of sustained attention. Neuropsychologia 45:2234–2245. https://doi.org/10.1016/j.neuropsychologia.2007.02.019
Noterdaeme M, Amorosa H, Mildenberger K, Sitter S, Minow F (2001) Evaluation of attention problems in children with autism and children with a specific language disorder. Eur Child Adolesc Psychiatr 10:58–66. https://doi.org/10.1007/s007870170048
Craig F, Margari F, Legrottaglie AR, Palumbi R, de Giambattista C, Margari L (2016) A review of executive function deficits in autism spectrum disorder and attention-deficit/hyperactivity disorder. Neuropsychiatr Dis Treat 12:1191–1202. https://doi.org/10.2147/NDT.S104620
Lai CLE, Lau Z, Lui SSY, Lok E, Tam V, Chan Q, Cheng KM, Lam SM, Cheung EFC (2017) Meta-analysis of neuropsychological measures of executive functioning in children and adolescents with high-functioning autism spectrum disorder. Autism Res 10:911–939. https://doi.org/10.1002/aur.1723
Miyake A, Friedman NP, Emerson MJ, Witzki AH, Howerter A, Wager TD (2000) The unity and diversity of executive functions and their contributions to complex “Frontal Lobe” tasks: a latent variable analysis. Cogn Psychol 41:49–100. https://doi.org/10.1006/cogp.1999.0734
Alderson RM, Rapport MD, Kofler MJ (2007) Attention-deficit/hyperactivity disorder and behavioral inhibition: a meta-analytic review of the stop-signal paradigm. J Abnorm Child Psychol 35:745–758. https://doi.org/10.1007/s10802-007-9131-6
Geurts HM, Corbett B, Solomon M (2009) The paradox of cognitive flexibility in autism. Trends Cogn Sci 13:74–82. https://doi.org/10.1016/j.tics.2008.11.006
Geurts HM, Grasman PPP, Verté S, Oosterlaan J, Roeyers H, van Kammen SM, Sergeant JA (2008) Intra individual variability in ADHD, autism spectrum disorders and Tourette’s syndrome. Neuropsychologia 46:3030–3041. https://doi.org/10.1016/j.neuropsychologia.2008.06.013
Lijffijt M, Kenemans JL, Verbaten MN, van Engeland H (2005) A meta-analytic review of stopping performance in attention-deficit/hyperactivity disorder: deficient inhibitory motor control? J Abnorm Child Psychol 114:216–222. https://doi.org/10.1037/0021-843X.114.2.216
van Zomeren AH, Brouwer WH (1994) Clinical neuropsychology of attention. Oxford University Press, New York
Posner MI, Walker JA, Friedrich FA, Rafal RD (1987) How do the parietal lobes direct covert attention? Neuropsychologia 25:135–145
Norman DA, Shallice T (1986) Attention to action: willed and automatic control of behaviour. In: Davidson RJ, Schwartz GE, Shapiro D (eds) Consciousness and self-regulation: advances in research and theory. Plenum Press, New York
Tucha L, Tucha O, Walitza S, Sontag TA, Laufkötter R, Linder M, Lange KW (2009) Vigilance and sustained attention in children and adults with ADHD. J Atten Disord 12:410–421. https://doi.org/10.1177/1087054708315065
Tucha O, Walitza S, Mecklinger L, Sontag TA, Kübber S, Linder M, Lange KW (2006) Attentional functioning in children with ADHD - predominantly hyperactive-impulsive type and children with ADHD—combined type. J Neural Transm 113:1943–1953. https://doi.org/10.1007/s00702-006-0496-450
Johnson KA, Robertson IA, Barry E, Mulligan A, Daibhis A, Daly M, Watchorn A, Gill M, Bellgrove MA (2008) Impaired conflict resolution and alerting in children with ADHD: evidence from the Attention Network Task (ANT). J Child Psychol Psychiatr 49:1339–1347. https://doi.org/10.1111/j.1469-7610.2008.01936.x
Samyn V, Roeyers H, Bijttebier P, Wiersema JR (2013) Attentional networks in boys with ADHD or Autism Spectrum Disorder and the relationship with effortful control. J Atten Disord. https://doi.org/10.1177/1087054712473183
Keehn B, Lincoln AJ, Müller RA, Townsend J (2010) Attentional networks in children and adolescents with autism spectrum disorder. J Child Psychol Psychiatr 51:1251–1259. https://doi.org/10.1111/j.1469-7610.2010.02257.x
van den Bergh B, Mennes M, Stevens V, van der Meere J, Börger N, Stiers P, Marcoen A, Lagae L (2006) ADHD deficit as measured in adolescent boys with a continuous performance task is related to antenatal maternal anxiety. Pedriatic Res 59:78–82. https://doi.org/10.1203/01.pdr.0000191143.75673.52
Tucha L, Fuermaier ABM, Koerts J, Buggenthin R, Aschenbrenner S, Weisbrod M, Thome J, Lange KW, Tucha O (2017) Sustained attention in adult ADHD: time-on-task effects of various measures of attention. J Neural Trans 124:39–53. https://doi.org/10.1007/s00702-015-1426-0
Huang-Pollock CL, Karalunas SL, Tam H, Moore AN (2012) Evaluating vigilance deficits in ADHD: a meta-analysis of CPT performance. J Abnorm Psychol 12:360–371. https://doi.org/10.1037/a0027205
Sinzig J, Bruning N, Morsch D, Lehmkuhl G (2008) Attention profiles in autistic children with and without comorbid hyperactivity and attention problems. Acta Neuropsychiatrica 20:207–215. https://doi.org/10.1111/j.1601-5215.2008.00292.x
Frazier TW, Demaree HA, Youngstrom EA (2004) Meta-analysis of intellectual and neuropsychological test performance in attention-deficit/hyperactivity disorder. Neuropsychology 18:543–555. https://doi.org/10.1037/0894-4105.18.3.543
Pasini A, Paloscia C, Alessandrelli R, Porfirio MC, Curatolo P (2007) Attention and executive functions profile in drug naive ADHD subtypes. Brain Dev 29:400–408. https://doi.org/10.1016/j.braindev.2006.11.010
Willcutt EG, Doyle AE, Nigg JT, Faraone SV, Pennington BF (2005) Validity of the executive function theory of attention-deficit/hyperactivity disorder: a meta-analytic review. Biol Psychiat 57:1336–1346. https://doi.org/10.1016/j.biopsych.2005.02.006
Günther T, Knospe EL, Herpertz-Dahlmann B, Konrad K (2015) Sex differences in attentional performance in a clinical sample with ADHD of the combined subtype. J Atten Disord 19:764–770. https://doi.org/10.1177/1087054712461176
Althaus M, de Sonneville LM, Minderaa RB, Hensen LG, Til RB (1996) Information processing and aspects of visual attention in children with the DSM-III-R diagnosis “pervasive developmental disorder not otherwise specified” (PDDNOS): I. Focused and divided attention. Child Neuropsychol 2:7–29. https://doi.org/10.1080/09297049608401347
Albrecht B, Brandeis D, Uebel H, Heinrich H, Mueller UC, Hasselhorn M, Steinhausen H, Rotherberger A, Banaschewski T (2008) Action monitoring in boys with attention-deficit/hyperactivity disorder, their nonaffected siblings, and normal control subjects: evidence for an endophenotype. Biol Psychiat 64:615–625. https://doi.org/10.1016/j.biopsych.2007.12.016
Tye C, Asherson P, Ashwood KL, Azadi B, Bolton P, McLoughlin G (2014) Attention and inhibition in children with ASD, ADHD and co-morbid ASD + ADHD: an event-related potential study. Psychol Med 44:1101–1116. https://doi.org/10.1017/S0033291713001049
Huang-Pollock CL, Nigg JT (2003) Searching for the attention deficit in attention deficit hyperactivity disorder: the case of visuospatial orienting. Clin Psychol Rev 23:801–830. https://doi.org/10.1016/S0272-7358(03)00073-4
Landry O, Parker A (2013) A meta-analysis of visual orienting in autism. Front Hum Neurosci 7:833. https://doi.org/10.3389/fnhum.2013.00833
Semrud-Clikeman M, Walkowiak J, Wilkinson A, Butcher B (2010) Executive functioning in children with Asperger syndrome, ADHD-combined type, ADHD-predominately inattentive type, and controls. J Autism Dev Disord 40:1017–1027. https://doi.org/10.1007/s10803-010-0951-9
Rühl D, Bölte S, Feineis-Matthews S, Poustka F (2004) ADOS. Diagnostische Beobachtungsskala für autistische Störungen. Huber, Bern
Bölte S, Rühl D, Schmötzer GPF (2006) ADI-R. Diagnostisches Interview für Autismus-revidiert. Huber, Bern
American Psychiatric Association (2000) Diagnostic and statistical manual of mental disorders, 4th edition, text revision (DSM-IV-TR). American Psychiatric Association, Washington
Achenbach TM (1991) Manual for the child behavior checklist and 1991 profile. University of Vermont, Department of Psychiatry, Burlington
Schneider S, Unnewehr S, Margraf J (2009) Kinder-DIPS. Diagnostisches Interview bei psychischen Störungen im Kindes- und Jugendalter. Springer, Berlin
Döpfner M, Lehmkuhl G (1998) Diagnostik-System für psychische Störungen im Kindes- und Jugendalter nach ICD-10 und DSM-IV: DISYPS-KJ; klinische Diagnostik—Elternurteil—Erzieher- und Lehrerurteil—Selbsturteil, 1st edn. Huber, Bern
Bölte S, Poustka F (2006) FSK Fragebogen zur Sozialen Kommunikation—Autismus Screening. Huber, Bern
Zimmermann P, Fimm B (2007) Testbatterie zur Aufmerksamkeitsprüfung (TAP), Version 2.1. Psytest, Herzogenrath
Erhart M, Dopfner M, Ravens-Sieberer U (2008) Psychometric properties of two ADHD questionnaires: comparing the Conners’ scale and the FBB-HKS in the general population of German children and adolescents–results of the BELLA study. Eur Child Adolesc Psychiat 17:106–115. https://doi.org/10.1007/s00787-008-1012-1
IBM Corp. Released (2013) IBM SPSS Statistics for Windows, Version 22.0. IBM Corp, Armonk
Benjamini Y, Hochberg Y (1995) Controlling the false discovery rate: a practical and powerful approach to multiple testing. J R Stat Soc Ser B Methodol 57:289–300. https://doi.org/10.2307/2346101
Adams ZW, Derefinko KJ, Milich R, Fillmore MT (2008) Inhibitory functioning across ADHD subtypes: recent findings, clinical implications, and future directions. Dev Disabil Res Rev 14:268–275. https://doi.org/10.1002/ddrr.37
De La Fuente A, Xia S, Branch C, Li X (2013) A review of attention-deficit/hyperactivity disorder from the perspective of brain networks. Front Hum Neurosci 7:192. https://doi.org/10.3389/fnhum.2013.00192
Reed P, McCarthy J (2012) Cross-modal attention-switching is impaired in autism spectrum disorders. J Autism Dev Disord 42:947–953. https://doi.org/10.1007/s10803-011-1324-8
Sachse M, Schlitt S, Hainz D, Ciaramidaro A, Schirman S, Walter H, Poustka F, Bolte S, Freitag CM (2013) Executive and visuo-motor function in adolescents and adults with autism spectrum disorder. J Autism Dev Disord 43:1222–1235
Sacrey LA, Armstrong VL, Bryson SE, Zwaigenbaum L (2014) Impairments to visual disengagement in autism spectrum disorder: a review of experimental studies from infancy to adulthood. Neurosci Biobehav Rev 47:559–577
O’Halloran L, Cao Z, Ruddy K et al (2017) Neural circuitry underlying sustained attention in healthy adolescents and in ADHD symptomatology. Neuroimage 169:395–406. https://doi.org/10.1016/j.neuroimage.2017.12.030
Verté S, Geurts HM, Roeyers H, Oosterlaan J, Sergeant JA (2006) The relationship of working memory, inhibition, and response variability in child psychopathology. J Neurosci Methods 151:5–14. https://doi.org/10.1016/j.jneumeth.2005.08.023
Lukito S, Jones CRG, Pickles A, Baird G, Happé F, Charman T, Simonoff E (2017) Specificity of executive function and theory of mind performance in relation to attention-deficit/hyperactivity symptoms in autism spectrum. Mol Autism 8:60. https://doi.org/10.1186/s13229-017-0177-1
Happé F, Booth R, Charlton R, Hughes C (2006) Executive function deficits in autism spectrum disorders and attention-deficit/hyperactivity disorder: examining profiles across domains and ages. Brain Cogn 61:25–39. https://doi.org/10.1016/j.bandc.2006.03.004
Jepsen JR, Fagerlund B, Mortensen EL (2009) Do attention deficits influence IQ assessment in children and adolescents with ADHD? J Atten Disord 12:551–562
Reiersen AM, Constantino JN, Volk HE, Todd RD (2007) Autistic traits in a population-based ADHD twin sample. J Child Psychol Psychiatry 48:464–472. https://doi.org/10.1111/j.1469-7610.2006.01720.x
Ronald A, Simonoff E, Kuntsi J, Asherson P, Plomin R (2008) Evidence for overlapping genetic influences on autistic and ADHD behaviours in a community twin sample. J Child Psychol Psychiatr 49:535–542. https://doi.org/10.1111/j.1469-76.10.2006.01720.x
Simonoff E, Pickles A, Charman T, Chandler S, Loucas T, Baird G (2008) Psychiatric disorders in children with autism spectrum disorders: prevalence, comorbidity, and associated factors in a population-derived sample. J Am Acad Child Adolesc Psychiatr 47:921–929. https://doi.org/10.1097/CHI.0b013e318179964f
Coghill D, Sonuga-Barke EJS (2012) Annual research review: categories versus dimensions in the classification and conceptualisation of child and adolescent mental disorders–implications of recent empirical study. J Child Psychol Psychiatr 53:469–489. https://doi.org/10.1111/j.1469-7610.2011.02511.x
Acknowledgements
We gratefully thank all children and their families for their participation in our study. Data collection was supported by grants of the German Research Foundation DFG to Christine M. Freitag and Jobst Meyer (Grants ME 1923/5-1, ME 1923/5-3), the German Federal Ministry of Education and Research (BMBF) Grant 01GV0605, and the BMBF/EC ERA-Net Grant 01EW1105 to Christine M. Freitag. Data analysis and writing was supported by the EU funded International Training Network MiND to CMF (Grant Number 82600321). CMF receives royalties for books on Attention-Deficit/Hyperactivity Disorder and Autism Spectrum Disorder. She has served as consultant to Desitin and Roche.
Author information
Authors and Affiliations
Corresponding author
Ethics declarations
Conflict of interest
None of the other authors has to report any conflict of interests.
Rights and permissions
About this article
Cite this article
Boxhoorn, S., Lopez, E., Schmidt, C. et al. Attention profiles in autism spectrum disorder and subtypes of attention-deficit/hyperactivity disorder. Eur Child Adolesc Psychiatry 27, 1433–1447 (2018). https://doi.org/10.1007/s00787-018-1138-8
Received:
Accepted:
Published:
Issue Date:
DOI: https://doi.org/10.1007/s00787-018-1138-8