Abstract
In food science the Maillard reaction is well known to cause degradation of amino acids and an overall decrease in the nutritional value of foods that have been subjected to heat in processing. There has been evidence more recently of the endogenous formation of some Maillard reaction products (MRPs) in biological systems and their association with pathophysiological conditions including diabetes, renal disease and cardiovascular disease. Several studies have suggested that dietary MRPs increase the in vivo pool of MRPs after intestinal absorption and contribute to the development of diabetes and related complications. This review focuses on the animal and human studies which have assessed the eventual implications of dietary MRPs on human health, highlighting the different diets tested, the experimental designs and the biomarkers selected to estimate the health effects. The results of these studies are compared to those of the recently published ICARE study. In this latter study an accurate determination of the MRP content of the diets was achieved, allowing the calculation of the contribution of individual food groups to daily MRP intakes in a regular western diet.
Similar content being viewed by others
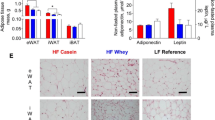
Avoid common mistakes on your manuscript.
Introduction
During the processing of foods, different chemical reactions occur which are essentially dependent on the temperature and duration of treatment and the food water content. They are responsible for the formation of characteristic flavor, color and texture usually wanted by the consumers who have adopted the western diet. In addition heat-processes are used to destroy harmful microorganisms and ensure food safety. However, from a nutritional perspective the heat treatment of foods may be deleterious as it leads to the destruction of nutrients such as vitamins, phenolic compounds and polyunsaturated fatty acids. The non-enzymatic Maillard reaction between free carbonyl groups, mainly reducing sugars, and free amino groups of proteins or free amino acids is also involved in the decrease of nutritional qualities of food. It leads to an irreversible chemical modification of amino acids and, in turn, to a net loss of essential amino acids in the diet (e.g., lysine) and to a decrease in protein digestibility (Rérat et al. 2002; Seiquer et al. 2006).
While the study of the protein nutritional loss within the heated food has been well studied during the past 40 years, two new questions have arisen more recently: are the Maillard reaction products (MRPs) toxic in biological systems? What are the consequences for human health of being exposed to food MRP? Two recent discoveries have stimulated the scientific research on the effect of excessive MRP consumption.
First of all in April 2002, the Swedish National Food Administration announced that acrylamide was present in a high concentration in various foodstuffs (Tareke et al. 2002). It was quickly established that asparagine, an amino acid present in free form in certain foods, was responsible for the food contamination. Indeed, in the presence of reducing sugar and after the Maillard reaction, the asparagine undergoes Strecker degradation leading to the formation of acrylamide. It is interesting to note that the major foods and beverages in which acrylamide has been found in significant concentrations are particularly rich in free asparagine (potato chips, French fries potatoes, cereal-based products and coffee). Despite the fact that the effect on human health of exposure to the relatively low level of acrylamide found in foods has not been clearly elucidated, the study of the deleterious effects causes by consuming a diet containing MRPs and other neoformed compounds has recently re-emerged.
Finally, the study of the Maillard reaction at 37°C in biological systems, called glycation, has revealed that some MRPs, also called advanced glycation end-products (AGEs) when formed in vivo, which accumulate in some tissues or are present in the systemic circulation, play a fundamental physiopathological role in cases of metabolic and aging disorders. For instance, the progression of cardiovascular diseases and non-insulin-dependent diabetes has been associated with the accumulation of N ε-carboxymethyl-lysine (CML) (Knecht et al. 1991; Meng et al. 1998). CML is the first MRP, or AGE, to be isolated and characterized from glycated proteins in vivo (Ahmed et al. 1986). It has also been found in urine (Wadman et al. 1975) and foods (Assar et al. 2009) and is currently used as a marker of both dietary and in vivo MRPs. Since the study of Koschinsky et al. (1997) on the oral absorption of MRPs, which were at that time suspected by the authors of containing reactive products and therefore named glycotoxins, it is still under debate whether an elevated exposure to MRPs could contribute to the systemic level of AGEs and their deposition in tissues, and thus accelerate oxidative stress and chronic diseases (Cai et al. 2008).
Both pre-clinical and clinical studies have been performed in recent years to determine whether a MRP-rich diet poses a risk for human health. This paper summarizes the main results obtained from animal experiments using regular and modified rodent diets. This is followed by a review of the few diet-controlled intervention trial and observation studies which have enrolled either healthy volunteers or subjects with metabolic disorders. Estimation from such studies of the mean exposure to dietary MRPs and the contribution of different foods to this exposure are also presented.
Main results of animal studies and their limits
Animal experiments have fundamentally contributed to the knowledge about the impact of dietary Maillard products on human health. Although its transposition to humans is always difficult to establish, the mouse model which is commonly used has brought many advances in the field of Maillard reaction and health. Two types of studies are usually conducted to evaluate the risk associated with the exposure to MPRs in foods: studies on the absorption, biodistribution, metabolism and elimination of ingested compounds of concern and studies on their biological activities.
Animal-experiment protocols are frequently designed to exaggerate the levels and conditions of exposure to dietary MRPs in order to provide in vivo data about potential health risks. At present and among other constraints, the lack of radioisotopes of MPRs results in a low accuracy in the measurement of the biodistribution and elimination of MRPs. In addition, oral exposure to MRPs is often performed using pure compounds diluted in drinking water or incorporated into the rodent diet which exert probably different health effects than MRPs bound to proteins or present in complex food matrices. These experimental conditions are often far from real exposure situations and may lead to misinterpretations of the relationship between dietary MRPs and health. Taking into account these limitations the main results for the literature are summarized below.
Among the dietary MRPs studied for their potentially deleterious health effects, CML is the one that has been most closely studied. Liardon et al. (1987) were the first to report on the urinary elimination of CML after collecting the urine in rats fed with proteins containing various quantities of CML. The dietary CML exposure has shown a positive relationship with its urinary excretion. The authors of this study concluded that the CML present in the urine was mainly of food origin. A more recent study performed with the same model confirms this relationship and indicates that the maximum urinary excretion of CML was 29% under the conditions of the study (Somoza et al. 2006). A fecal excretion of 15–22% was also observed. This study showed that plasma and tissue accumulations of CML are also significantly affected by food consumption, and that kidneys are the main organs responsible for the regulation of CML metabolism. A CML renal clearance of 0.47 mL/min has been measured in Sprague–Dawley rats (Karachalias et al. 2010).This efficient renal excretion was also confirmed by following the clearance of a [18F]-fluorobenzylated analogue of CML injected into the rat tail vein (Bergmann et al. 2001). Despite the fact that the derivatized CML does not have exactly the same biodistribution and clearance as the unmodified adduct, important observations have been made. Apart from a temporary liver accumulation, no permanent tissue storage of CML was detected. Thus, unlike other data, this study does not confirm that the in vivo level of CML depends on dietary exposure. However, a significant increase in circulating and tissue CML was observed under pathological conditions such as kidney failure conditions. It has been observed mainly from laboratory rats but also human subjects that free CML only, not protein-bound CML, accumulates in the systemic circulation when renal clearance is impaired or lost (Karachalias et al. 2010; Agalou et al. 2005, Rabbani et al. 2007). These studies suggest that it is mainly the free form of CML which is excreted in urine and confirm that reduced kidney function increases the level of circulating CML.
The renal clearance of another MRP, pentosidine, was measured in rats after the intravenous injection of a dose of its radiolabeled form (Miyata et al. 1998). The results of this latter experiment suggest that pentosidine is reabsorbed by the proximal tubule after glomerular filtration. It is then metabolized before excretion in the urine. Overall, this study confirms the key role of the kidney in the metabolism and elimination of some MRPs.
Besides the loss of nutritional value of protein related to the irreversible modification of amino acids in heated foods, such as glucosyllysine, lysinoalanine and CML, potentially deleterious health effects of these non-physiological amino acids have been described. Several laboratory animal experiments were designed to evaluate the biological effects of a dietary exposure to MRPs. A 2-month study on rats has been performed to examine the antinutritional and antigrowth properties of MRPs. Despite the addition of free amino acids in the diet to compensate for nutritional losses during the heat treatment of caseins, the intake of Maillard-modified proteins exposed the animals to unidentified compounds responsible for a slowdown of growth (Utsunomiya et al. 1990). However, this intake of modified proteins did not affect the weight of the internal organs after 2 months of feeding.
These last observations are comparable with those of another study in streptozotocin-diabetic rats which did not reveal any toxicity of dietary MRPs on the biological systems observed (Chuyen et al. 2005). Moreover, the exposure to dietary MRPs does not appear to be responsible for the high oxidative stress observed in diabetic animals.
A series of animal studies conducted by the scientific team of Helen Vlassara found that low MRP consumption may prevent or delay the incidence of complications associated with chronic diseases (Vlassara 2005). From a technical standpoint the rodent food containing a reduced amount of MRPs (e.g. CML) has generally been less severely heated than conventional laboratory food. According to the authors of these studies both types of food were nutritionally identical. However, some doubts have been raised about the amounts of vitamins and minerals in low MRP diets (e.g. thiamine and pyridoxine) which may be significantly higher than in regular feed (Buetler 2007). The level of intake of micronutrients and antioxidants is perhaps a major confounding factor in many studies concerning the association between MRP exposure and health.
Despite a recognized need for a better control of this factor, several observations can be made about the impact of a low MRP diet versus a regular diet: (a) the consumption of a low MRP diet is associated with a reduction of body weight and fasting insulin concentration and an increase of plasma HDL level in diabetic mice (db/db) (Hofmann et al. 2002), (b) in healthy mice, the insulin resistance induced by a high-fat diet is decreased when the exposure to MRP is limited (Sandu et al. 2005), (c) among the complications associated with diabetes mellitus, vasculopathy (Lin et al. 2003), nephropathy (Zheng et al. 2002) and impaired wound healing (Peppa et al. 2003) are improved when MRPs are avoided in the diet. Finally, it was found more recently that a 50% decrease in MRPs of the diet could extend animal lifespan by limiting the exposure to potentially pro-oxidant MRPs (Cai et al. 2007). It was then concluded that the beneficial effects of calorie restriction on aging and related complications would be partly due to a limited exposure to reactive MRPs (Cai et al. 2008).
Overall, the animal experiments conducted in this field provide some reliable scientific data indicating a significant impact of dietary MRPs on metabolism dysregulation and chronic diseases. However, it is difficult to extrapolate human risk from animal studies. For the reasons mentioned previously the type of exposure, the net absorption across the intestine tissue, and the health effects of MRPs in animal models are probably relatively different from that of humans. Therefore, rigorous clinical studies are needed to examine the biological effects of dietary MRPs with regard to the food consumption habits (e.g. food preferences, cooking traditions, and age-related eating patterns) in different populations.
The human studies conducted to evaluate the health impact of dietary MRPs
There is a limited number of studies that have been used to investigate the health effects of dietary Maillard neoformed compounds in humans. Some observational studies have been carried out to address the question of absorption, biodistribution and elimination of dietary MRPs, and to observe the associations between food exposure to MRPs and their in vivo levels (Table 1). Some few randomized controlled studies have been designed to answer the same questions, as well as to provide further evidence of an association between dietary MRPs and biomarkers of cardiovascular and diabetes risks in healthy volunteers or in patients with diabetes or renal disease (Table 2).
Diets and experimental designs of the different human studies
Studies on infants
Among the few observational studies published recently, two have been conducted during infancy. They have compared breastfeeding and formula feeding (Table 1). In both studies CML was used as an indicator of the MRP level in milk. Using a validated ELISA method, Dittrich et al. (2006) found a 35-fold higher amount of CML in infant formulas compared to breast milk. The small size of the groups, due to difficulties in recruiting volunteers, and the high variability of the CML concentration among the few infant formulas administrated, limited the significance of the findings. With a GC–MS/MS method, the CML contents in 16 infant formulas have been measured by Sebeková et al. (2008) in a second study involving 59 infants. In this study, a median 70-fold higher level of CML was measured in formulas compared to breast milk. Thus, a much higher difference of daily CML exposure was found between breast-fed and formula-fed infants (Fig. 1b). The higher difference between the formulas and breast milk in this second study can be explained by a higher CML level in breast milk in the first study and a higher CML concentration in infant formulas in the second one where half formulas were hypoallergenic with three times more CML than regular formulas.
Cross-sectional studies
Two cross-sectional studies from Uribarri et al. (2003b, 2007) have been conducted on large adult populations. In the first published study which was performed on 189 patients with end-stage renal disease, the daily dietary MRP intakes were compared to the serum CML levels. In order to assess the dietary exposure to MPRs, information about concentrations of MRPs in foods was collected in a database (≈200 foods) using a non-validated method, and consumption levels of these foods were estimated based either on a 3-day food record or on a 15-min food frequency questionnaire. Since MRP levels in foods are affected by the way the food is prepared, cooking-related questions were included into the questionnaire. The second cross-sectional study involved 172 healthy volunteers. In this case a similar MRP intake assessment was made using a more complete database (>250 foods). The uncertainty of the exposure assessment is difficult to quantify; however, some potential sources of error can be pointed out. First of all food intake data based on a 3-day record do not account for the individual long-term exposure and may either overestimate or underestimate the regular consumption of some foods which contribute highly to the daily MRP intake and, perhaps, to the in vivo level of MRPs. A combination of complementary methods (Kroes et al. 2002) such as a record method combined with a food frequency questionnaire is probably one of the best ways to assess food consumption and eventually dietary exposure to AGEs. The second gap in the exposure assessment is the use of mean AGE levels from a food database which are not always representative of the real concentration of these compounds in the food consumed by the volunteers surveyed. Indeed, a large variability of acrylamide (Dybing et al. 2005), CML and other neoformed compounds (Birlouez-Aragon et al. 2010a, b) has been reported among the food products available on the market.
Finally, another limitation is that the analytical methods used for MRP measurement in foods are not always fully validated and can lead to incorrect values. This is particularly the case in the methods based on an enzyme-linked immunosorbent assay (ELISA), which have the advantage of being rapid and inexpensive, but not always adapted to the analysis of complex food matrices. Important criticisms have been brought recently about the CML measurements in foods obtained by ELISA (Delatour et al. 2009). For instance, using this method, the concentration of CML in fat products (e.g. butter) was reported to be up to 5,300-fold higher than that in whole milk. Assar et al. who have not found such results using an UPLC–MS method have pointed out that high levels of CML in olive oil and butter are very unlikely to be found since no, or only low concentrations of protein are present in these foodstuffs. Therefore, it is now suggested that uncontrolled matrix interferences impair the CML measurement at least in fat foods when an ELISA method is used.
Unfortunately most of the human studies published on the topic of the Maillard products have used a food database published by Goldberg et al. (2004) and more recently updated by Uribarri et al. (2010). Nowadays, almost 550 foods are listed in this database for which the AGE content (or MRP content) is based on CML content using an ELISA. To our knowledge, no validation of the method used for this database has been published.
Intervention studies
Fewer than 20 intervention studies related to the field of the MRPs and health have been published since Koschinsky et al. (1997) postulated that diet-derived AGEs could be biologically active and thus contribute to the complications of diabetes mellitus. Depending on the goal and the means of a clinical trial, the study design was different (Table 2). In most cases, a 2- to 6-week randomized study was done to assess a long-term relationship between dietary MRPs and health. However, a few studies carried out during only a short period of time resulted in valuable data on the absorption and elimination of MRPs. For instance, the intake of a single meal composed of egg white heated with glucose (Koschinsky et al. 1997) has led to the observation of a postprandial increased serum level of AGEs. Another study with 18 healthy volunteers was conducted to follow the urinary elimination of 3 MRPs (Amadori products, pentosidine and pyrraline) during a 4-day diet containing either 250 g of pretzel sticks, 600 mL of coffee brew or 350 g of custard (Förster et al. 2005). This study of short-term but well-defined exposure to different MRPs has added important new knowledge to the understanding of the bioavailability of dietary MRPs. One last short-term intervention study compared the intake of two meals composed of the same ingredients, chicken breast and vegetables, but which were either fried and grilled or steamed and boiled (Negrean et al. 2007). According to the ELISA method used to quantify CML in foods, the two meals had a 55-fold difference in their MRP concentration (Fig. 1a). In addition to the concerns mentioned above about the validity of the analytical method used, one of the main disadvantages is that the MRP concentrations are expressed in arbitrary units (kilounits AGE) and cannot be compared to other studies which express the exposure to MRPs in mass of neoformed compound per unit mass of food consumed or per unit body weight/day.
Among the intervention trials carried out over a period of 2–6 weeks, two types of diet design were used. The volunteers had either to prepare their food at home, with some instructions regarding the cooking procedures that they could use, and the types of foods that they could buy, or had to eat the meals selected and/or prepared by the investigators. Obviously this second protocol inspires more confidence about the compliance to the diets tested. Pouillard et al. (2008) have presented the limits discovered, and criticisms frequently expressed about this type of clinical study, and exposed the strategy to produce diets that are similar in terms of macronutrient and micronutrient contents, but different in the level of MRPs. Overall, a compromise has to be found between the use of realistic diets which may not be perfectly identical in nutritional quality, and the use of well-defined doses of MRPs added to a basal diet which may not be representative of the types of foods eaten in the countries of the western world.
Only three studies have accurately assessed the daily exposure to CML and Amadori products (AP) by quantifying the meal/food contents using chromatographic methods. When the high MRP diets were consumed, the daily intakes of CML were estimated to be 252 and 83 μg/kg body weight (bw)/day for the study #9 and #12 (Table 2), respectively, whereas when the low MRP diets were selected the intakes were 90 and 34 μg/kg bw/day, respectively (Fig. 1b). These results are largely in agreement with a previous estimation of the daily intake of total MRPs which was essentially based on CML and pyrraline intakes from a conventional diet (Henle 2003). At this time the author estimated that the amount of ingested MRPs was between 385 and 1,154 μg/kg bw/day (assuming an average adult bw of 65 kg). Overall in the studies #9 and #12 the CML exposure from low and high MRP diets differed by ≈3- and 2-fold, respectively, which is close to the fivefold difference in MRPs measured in study #6. The only study which indicated a very high exposure to CML and a considerable difference between the two diets was the one conducted on infants in the frame of the ICARE project (study #4). A 130-fold difference in CML exposure was assessed between breast fed and formula fed infants (Fig. 1b). When expressed as μg/kg bw/day the values of exposure are obviously inversely proportional to body mass. Therefore, the differences of mean body weight between adolescents (55 kg) and adults (65 kg) explain the differences of MRP exposure found between studies. And, still on a body weight basis, the exposure to CML is much higher for 6-month-old infants (≈7 kg) compared to adolescents or adults. However, the high CML exposure found among formula fed infants is also due to the high concentration of this MRP in most commercial infant formulas and the high and exclusive formula intake per bw characteristic of growing infants (Fenaille et al. 2006; Sebeková et al. 2008).
Beside CML, other Maillard products have also been assessed in the different diets. Between the low and high MRP diets the daily intakes of AP were estimated to be 1.88 and 1.65 mg/kg bw/day for the study #9, 0.59 and 0.63 mg/kg bw/day for the study #12, and 55.50 and 0.17 mg/kg bw/day for the study #4, respectively. It has to be mentioned that the AP exposure in study #12 did not take into account the consumption of beverages such as heated milk. Thus, when the intake of a portion of UHT milk (200 mL) was added to the assessment, the daily intakes of AP from low and high MRP diets were 0.86 and 0.90 mg/kg bw/day, respectively. The AP exposures reported in these intervention studies are similar to those estimated earlier (Henle 2003).
The dietary levels of 5-hydroxymethylfurfural (HMF) were measured in two intervention studies. The measurements reported in Fig. 1d show a similar exposure to HMF in the high MRP diets of the studies #9 and #12: 91.5 and 77.0 μg/kg bw/day, respectively. For an arbitrary adult bw of 65 kg, it was previously estimated that the exposure to HMF could be up to 2.3 mg/kg bw/day (Ulbricht et al. 1984). This is much above the levels observed in the two interventions studies which have measured HMF in realistic Spanish and French diets.
Finally, the acrylamide exposure from foods was never assessed in the intervention trials listed in Tables 1 and 2, except for the ICARE study in adults (study #12). In this last study the mean dietary intake of acrylamide averaged 0.49 μg/kg bw/day when a standard diet (high MRP diet) was consumed for 4 weeks whereas the exposure was more than fivefold lower (0.09 μg/kg bw/day) among volunteers who ate a similar diet, but lightly concentrated in MRPs (Fig. 1e). The dietary exposure found with the standard diet of the ICARE intervention trial is in the range of reported average intakes calculated by national food administrations (Mucci and Wilson 2008). For example, the low acrylamide exposure obtained with the low MRP diet was achieved using mashed potatoes instead of French fries, steamed cornflakes instead of extruded ones.
Relative contribution of individual food groups to CML, acrylamide, HMF and Amadori products intakes in the regular diet of the ICARE clinical study on healthy adults
When clinical studies related to health effects of food contaminants are designed, it is important to include accurate and detailed food analyses in the protocol. However, only a few studies have been designed to provide reliable data on MRP content in foods, using chromatographic analytical methods (LC–UV, LC–MS and GC–MS/MS). In order to determine what are the main sources of dietary MRPs, two types of food samples were collected and analyzed in the ICARE clinical study: a mixture of all the portions of food proposed per day, and samples of foods which constitute the diets tested and which are suspected of containing a significant amount of MRPs. Therefore, knowing the daily exposure to MRPs from the proposed menus and the MRP concentrations of each selected food product, it was possible to assess which food items contribute most to CML, HMF, AP and acrylamide exposures. It has to be noted that for technical reasons such as the MRP dilution in food samples, the contribution of beverages was not measured in this part of the ICARE study. Furthermore, other MRPs such as the group of imidazolones which have been detected in beverages and foods, and in vivo, were not quantified in the meals of the ICARE study despite their reported high concentration in foods (Henle et al. 1994; Ahmed et al. 2005).
The food group that contributes most to CML exposure in the ICARE study was the cereal group including bread (27%), breakfast cereals (12%) and biscuits (17%) (Fig. 2a). The grilled meat and fish were also two important contributors to dietary CML (16%), followed by the group of dairy products (14%) where gratins accounted for the highest contribution (10%) and without taking into account the milk ingested at breakfast. When a portion of 200 mL of UHT milk was added to the calculation (1.3 mg CML/L), the relative contribution of the dairy products to total CML intake becomes higher (19%). Despite appropriate portion sizes in the menu and irrespective of the cooking method, vegetables did not contribute to the CML exposure (1%). These data are the first to estimate the relative contribution of different food groups to the CML intake in a regular diet. Without data on the food consumption patterns, a database on the level of CML in foods is not enough to determine what foods or food groups are major sources of CML. However, some scientists have tried to predict which foods could be the main sources of CML. Despite the absence of any survey to measure food intakes in their study, Assar et al. (2009) estimated that bread crust, fried meat and cheddar cheese could be among the main sources of dietary CML. The important contribution of bakery products was also presented by Henle (2003) after collecting data from the literature. Conversely, from the recently published database on the AGE content in 549 foods (using an ELISA based on an anti-CML antibody), it has been stated that the carbohydrate group including cereal-based products contained low levels of AGE and thus was a minor source of dietary AGE. Since there is a certain amount of doubt associated with the analytical method used to establish this database (e.g. potential matrix interferences), no clear conclusion can be drawn in the absence of analytical validation.
Since bread and extruded breakfast cereals were eaten daily in relatively high amounts in the regular diet of the ICARE study, ≈120 and 60 g/day, respectively, the cereal group also contributed a considerably high proportion of the total HMF intake. Together with biscuits and other bakery products, the cereal group was estimated to be almost the unique source of dietary HMF (Fig. 2b). Here again, databases on HMF content in foods have been published but, to our knowledge, no relative contribution of foods to the HMF exposure was presented before the ICARE study. However, the contribution of cereal-based products was predictable since many analytical studies have found this MRP in high amounts in bread, breakfast cereals and other related foodstuffs (Morales 2009). The contribution of hot beverages such as coffee or chicory was not calculated in the ICARE study but may have been important for HMF intakes.
The intake of AP from the regular diet of the ICARE study came also mainly from bread (52%), biscuits (13%) and other bakery products (Fig. 2c). However, when the intake of 200 mL of hot UHT milk was added to the calculation, the contribution from bread (35%) and biscuits (13%) was lower and an estimate of a 32% contribution from the milk was made. Overall, these data are in agreement with previous reports based on furosine analysis in foodstuffs (Erbersdobler and Somoza 2007; Delgado-Andrade et al. 2005).
Finally, the relative contribution of the different food group to the acrylamide intake was as follows: French fries 71%, breakfast cereals 9%, bread 6% and ginger bread and biscuits 7% (Fig. 2d). The relative contribution of foods to dietary acrylamide has been evaluated extensively in the past decade and data from different countries have been reviewed recently (Mucci and Wilson 2008). The sources of acrylamide exposure between populations differ depending on their eating habits and cooking styles. Independent of these variations, the overall daily exposure has been attributed mainly to French fries and chips, and breakfast cereals while the contribution of bread and coffee becomes important in many European countries, due to high intakes compared to other world regions (Dybing et al. 2005). As commented before, drinks such as coffee (0.7 μg/200 mL) and chicory (38 μg/20 g) were not included in the calculation of the main sources of acrylamide in the ICARE clinical study, inducing an overestimation of the relative contributions of French fries (200 g/week), and cereal-based products (≈1,500 g/week).
Compared biological effects of diets with high and low MRP contents
Bioavailability of MRPs under controlled diets
Apart from the assessment of the biological activity of ingested MRPs, some clinical studies have also focused on the evaluation of the absorption, biodistribution and clearance of these compounds. Some recent human studies have examined the biodistribution of orally administered early MRPs (e.g. fructoselysine). The results indicate that more than 95% of the ingested fructoselysine is not recovered in urine and feces, and is probably metabolized (Erbersdobler and Faist 2001). In addition, the small fraction of fructoselysine excreted in the urine is dependent on the content of this MPR in the diet (Förster et al. 2005), and the excretion seems to occur rapidly between 2 and 9 h after the ingestion (Erbersdobler and Faist 2001). Among other advanced MRPs, CML, pentosidine and pyrraline have been the focus of studies concerning their absorption and elimination. In a 4-day clinical experiment approximately 50 and 60% of the ingested pyrraline and free pentosidine were eliminated in the urine, respectively (Förster et al. 2005).
More data have been obtained regarding CML. Using an ELISA with an antibody specific to CML, serum and urine levels of AGEs were determined in some clinical studies after controlled CML ingestion (Tables 1, studies #1–3; 2, studies #5, 6, 8, 10, 11). It was concluded that the so-called “dietary AGEs” are transported to the blood circulation where their concentration was proportional to the quantity ingested through the diet. According to Koschinsky et al. (1997), only 10% of the oral load of AGEs was intestinally absorbed and transported to the bloodstream. In addition, only one-third of the absorbed AGEs was eliminated in the urine.
The amount of AGEs in serum was also found to be proportional to the severity of kidney disease. This has been linked to a decrease in urinary excretion of AGEs related to renal impairment. Using the same controversial immunoassay (detailed above), two observational studies involving large groups have confirmed the significant association between dietary exposure to AGEs (expressed as AGE equivalents/day) and circulating levels of CML (expressed as U/mL) in healthy volunteers and renal failure patients (Table 1, studies #3 and 1). Although it is unclear whether the serum CML is derived from a dietary intake of highly reactive carbonyl intermediates (e.g. glyoxal) or directly from the absorption of CML present in foods, it is assumed that the level of circulating CML is more affected by the dietary exposure to AGEs than endogenous glycation. This recent assumption was strengthened by the observation that both in animal and human studies hyperglycemic conditions have a lower impact on serum CML levels than dietary AGE intakes (Zheng et al. 2002, Uribarri et al. 2003b). However, the accumulation of AGEs in vivo seems not always directly affected by the food exposure to AGEs and the level of glycemia since therapeutic interventions made to decrease AGE precursors in animal models decrease the urinary excretion of AGEs without change in food consumption (Karachalias et al. 2010).
The bioavailability of CML has also been studied in human intervention studies using either gas or liquid chromatographic techniques coupled with tandem mass spectrometry for a direct quantification of CML in foods and biological samples. A 2-week crossover study that compared a low and a high MRP diets in adolescent males was designed to collect urine and feces during the last 72 h (Table 2, study #9). The volume of the urine samples and the weight of the fecal samples were measured. Data on sample preparation are provided in the original publication (Seiquer et al. 2006). The CML analysis in our laboratory showed that the fecal excretion of CML was more important than the urinary excretion (Tessier et al. 2010). The fecal excretion of CML and the urinary ones were 186 and 25% higher, respectively, in adolescents who consumed a high MRP diet compared to adolescents who received a low MRP diet. It was also found that a total of ≈47% of the ingested CML was eliminated in urine and feces regardless of the type of diet received representing 5.2 and 2.5 mg of CML eliminated per day in volunteers on the high and low MRP diets, respectively. It can also be concluded that 53% of the ingested CML was not eliminated in its original chemical form within 72 h. In a similar animal study partial clearance and fecal elimination have been described including the non-recovery of ≈50% of the ingested CML (Somoza et al. 2006). The elimination of an unidentified metabolite of CML and the accumulation of CML into tissues could explain this incomplete elimination.
Another intervention study (Table 2, study #12) confirmed that the clearance of CML was 40% higher when healthy volunteers ate a high MRP diet compared to a low MRP diet. The plasma CML was also found to be 7% higher using the same comparison.
Finally, a positive but not significant association was found between the amount of CML in milk and its urine excretion in 21 infants (Table 1, study #2). With a larger group of infants the association was confirmed with the observation of a 60-fold higher urinary excretion of CML in the formula-fed group compared to the breast-fed group (Table 1, study #4). In this study, the plasma CML concentration in infants was also dependent on the type of formula ingested, hypoallergenic or not.
Other research protocols than intervention studies with controlled diets can provide valuable data regarding the clearance of circulating MRPs. For instance, bearing in mind the limitation of the following in vivo method (Svistounov and Smedsrød 2004), the measurement of MRPs that enter and leave an organ was used by Ahmed et al. (2004) to determine whether or not liver could be a major site of extraction of MRPs. The results of this study did not indicate any hepatic elimination of the protein-bound or free MRPs measured (fructoselysine, CML, N ε-carboxyethyl-lysine, AP, pentosidine, and hydroimidazolones) in healthy subjects. The authors concluded that these results reinforce the hypothesis that the kidneys play a major role in the elimination of circulation free MRPs.
Impact of dietary MRPs on health
It has been a subject of review above that dietary MRPs may contribute to insulin resistance, atherosclerosis, inflammation and endothelial dysfunction in different rodent models. These results cannot be fully extrapolated to humans and, therefore the clinical studies listed in Tables 1 and 2 have been essentially designed to evaluate the health effects of exogenous MRPs. It was initially tested in patients with diabetes and/or renal failure who were believed to be more vulnerable to chronic ingestion of pro-inflammatory and pro-oxidant MRPs.
Several serum markers have been monitored to detect a possible impact of dietary MRP. For instance, a decreased exposure to MRPs was reported to suppress inflammatory mediators such as tumor necrosis factor-α, vascular cell adhesion molecule-1, C-reactive protein (CRP) in study #6 and fibrinogen, interleukin 6 and CRP in study #10. Markers of oxidative stress were also assessed in different intervention studies. The level of malondialdehyde in lipoproteins (study #8) and that of serum TBARS (study #10), two indicators of lipid peroxidation, were found to be promoted by a high dietary exposure to MRPs.
The chemical changes of low-density lipoproteins (LDL) in relation to exogenous MRP loads were observed. Beside glycoxidation of LDL by hyperglycemia (Bucala et al. 1994), exogenous MRPs are suspected of binding to LDL. Study #8 showed that AGE-modified LDL was positively associated with the level of MRPs in the meals consumed. However, despite a similar HbA1c in the two groups tested, the lower fasting glucose in patients on the low MRP diet could be the reason for the apparent association found between AGE-modified LDL and MRP intake. However, similar results were obtained in a second study where the diabetic volunteers on the high MRP diet had higher levels of glycated and oxidized LDL despite comparable fasting glycemia between the groups (study #6). AGE-modified LDL isolated from these patients were found to promote intracellular oxidative stress. In addition to hyperglycemia the food exposure to MRPs can indeed increase the rate of alternated LDL, eventually leading to vascular toxicity including inflammation and oxidative stress. This intervention study was the first to describe the potential atherogenic effect of dietary MRP in diabetic patients, and this result was later confirmed in study #10 in a similar protocol. In addition, this last cross-over study demonstrated that a restriction in dietary MRPs in a single meal improved the postprandial micro- and macro-vascular functions.
In healthy volunteers only two clinical studies have tried to assess the impact of ingestion of MRPs on oxidative stress, inflammation and dysregulation of the glucose metabolism. The observational study #3 performed on 172 healthy individuals demonstrated that the MRPs intakes correlated significantly with a serum marker of inflammation (hsCRP). Plasma 8-isoprostane, indicator of lipid peroxidation, and HOMA, indirect assessment of insulin resistance, were both significantly correlated with the circulating level of CML. However, no direct correlation was found with the quantity of MRPs ingested.
The ICARE study also investigated the possible impact of a diet with high versus low MRP level on well-established indicators of cardiovascular and diabetes risk (study #12). HDL and total cholesterol, as well as triglycerides, fasting insulinemia and HOMA were higher after the 4-week high MRP diet compared to the low MRP diet, while vitamins C and E were lower. The changes affecting the lipid fraction of plasma were correlated with the plasma CML concentration. It was thought necessary to investigate the cause of plasma changes, and whether these had resulted from eating the high MRP meals which included modified nutritional factors resulting unintentionally from the cooking processes. Correlations between the plasma changes and the intakes of macronutrients, vitamin C and calorie content were therefore investigated and none were found. Despite the absence of correlation with serum CML the decreased levels of vitamins E and C in the plasma suggested an increased oxidative stress. However, these last results must be interpreted cautiously since more vitamin C was found in the low MPR diet despite an adjustment of the vitamin intakes between the two tested diets with orange juice and other vitamin C-rich foods (Pouillart et al. 2008). However, no correlation was found between plasma and dietary vitamin C, suggesting that the decrease in vitamin would not be the consequence of a lower intake. In this well-controlled study, no correlation was found between dietary and plasma CML, in contrary to other studies where no precise individual assessment of the CML intake was measured. Of course a global indirect correlation is always expected due to the fact that a high MRP diet through cooking at high temperatures is associated with higher plasma CML levels, but plasma CML was mainly found on proteins and not as free Maillard adduct in the circulation. Consequently, it is difficult to understand that plasma CML could derive from the dietary absorbed CML. We better suggest that the modified amino acid is produced by the in vivo reaction between proteins and dietary reactive carbonyl compounds, also called glycotoxins.
Conclusion
Considerable progress has been made in determining whether an elevated dietary intake of MRPs could contribute to the systemic level of AGEs and thus accelerate oxidative stress and chronic diseases such as cardiovascular disease and diabetes. It can be concluded that a diet rich in MRPs, tested over a period of 2–6 weeks, may accelerate oxidative stress, inflammation, and mechanisms related to glucose regulation especially for some vulnerable groups such as diabetics and renal failure patients. Few studies have evaluated the risk associated with the intake of dietary MRPs in healthy subjects. The recent results of the ICARE study in healthy adults confirmed the effective deleterious effects of a diet mainly composed of highly thermally processed foods.
However, several studies suggest that some MRPs present in foods could have beneficial effects on health. For instance, the melanoidins (reviewed elsewhere in this volume) are brown Maillard polymers which seem to have functional properties in food products (Rufian-Henares and Morales 2007) and are also capable of inhibiting growth of a tumor cell line in culture (Marko et al. 2003). In addition, it was also found recently that a selection of foods rich in MRPs could inhibit the oxidation of LDL in vitro (Dittrich et al. 2009). The high diversity of the MRPs formed in the very diverse food matrices makes it impossible to classify all of them as glycotoxins. It is admitted that they have different beneficial or detrimental biological activities. Thus, more well-controlled clinical experiments are needed to establish the role of the ingested MRPs, pure or added to food matrices, following acute or chronic exposures. Finally, it is essential that only fully validated analytical tools should be used or developed to assess the level of MRPs in foods and biological samples. And original strategies should be proposed to differentiate in vivo the MRPs endogenously formed and those coming from foods.
References
Agalou S, Ahmed N, Babaei-Jadidi R, Dawnay A, Thornalley PJ (2005) Profound mishandling of protein glycation degradation products in uremia and dialysis. J Am Soc Nephrol 16:1471–1485
Ahmed MU, Thorpe SR, Baynes JW (1986) Identification of N epsilon-carboxymethyllysine as a degradation product of fructoselysine in glycated protein. J Biol Chem 261:4889–4894
Ahmed N, Thornalley PJ, Luthen R, Haussinger D, Sebekova K, Schinzel R, Voelker W, Heidland A (2004) Processing of protein glycation, oxidation and nitrosation adducts in the liver and the effect of cirrhosis. J Hepatol 41:913–919
Ahmed N, Mirshekar-Syahkal B, Kennish L, Karachalias N, Babaei-Jadidi R, Thornalley PJ (2005) Assay of advanced glycation endproducts in selected beverages and food by liquid chromatography with tandem mass spectrometric detection. Mol Nutr Food Res 49:691–699
Assar SH, Moloney C, Lima M, Magee R, Ames JM (2009) Determination of Nepsilon-(carboxymethyl)lysine in food systems by ultra performance liquid chromatography-mass spectrometry. Amino Acids 36:317–326
Bergmann R, Helling R, Heichert C, Scheunemann M, Mäding P, Wittrisch H, Johannsen B, Henle T (2001) Radio fluorination and positron emission tomography (PET) as a new approach to study the in vivo distribution and elimination of the advanced glycation endproducts N epsilon-carboxymethyllysine (CML) and N epsilon-carboxyethyllysine (CEL). Nahrung 45:182–188
Birlouez-Aragon I, Morales F, Fogliano V, Pain J-P (2010a) The health and technological implications of a better control of neoformed contaminants by the food industry. Path Biol 58:232–238
Birlouez-Aragon I, Saavedra G, Tessier FJ, Galinier A, Ait-Ameur L, Lacoste F, Niamba CN, Alt N, Somoza V, Lecerf JM (2010) A diet based on high-heat-treated foods promotes risk factors for diabetes mellitus and cardiovascular diseases. Am J Clin Nutr. doi:10.3945/ajcn.2009.28737
Bucala R, Makita Z, Vega G, Grundy S, Koschinsky T, Cerami A, Vlassara H (1994) Modification of low density lipoprotein by advanced glycation endproducts contributes to the dyslipidemia of diabetes and renal insufficiency. Proc Natl Acad Sci USA 91:9441–9445
Buetler T (2007) Heated diets, AGEs and tissue dysfunction. IMARS Highlight 2:1–2
Cai W, He JC, Zhu L, Peppa M, Lu C, Uribarri J, Vlassara H (2004) High levels of dietary advanced glycation end products transform low-density lipoprotein into a potent redox-sensitive mitogen-activated protein kinase stimulant in diabetic patients. Circulation 110:285–291
Cai W, He JC, Zhu L, Chen X, Wallenstein S, Striker GE, Vlassara H (2007) Reduced oxidant stress and extended lifespan in mice exposed to a low glycotoxin diet. Association with increased AGER1 expression. Am J Pathol 170:1893–1902
Cai W, He JC, Zhu L, Chen X, Zheng F, Striker GE, Vlassara H (2008) Oral glycotoxins determine the effects of calorie restriction on oxidant stress, age-related diseases, and lifespan. Am J Pathol 173:327–336
Chuyen NV, Arai H, Nakanishi T, Utsunomiya N (2005) Are food advanced glycation end products toxic in biological systems? Ann NY Acad Sci 1043:467–473
Delatour T, Hegele J, Parisod V, Richoz J, Maurer S, Steven M, Buetler T (2009) Analysis of advanced glycation endproducts in dairy products by isotope dilution liquid chromatography-electrospray tandem mass spectrometry. The particular case of carboxymethyllysine. J Chromatogr A 1216:2371–2381
Delgado-Andrade C, Rufián-Henares JA, Morales FJ (2005) Fast method to determine furosine in breakfast cereals by capillary zone electrophoresis. Eur Food Res Technol 221:707–711
Dittrich R, Hoffmann I, Stahl P, Müller A, Beckmann MW, Pischetsrieder M (2006) Concentrations of N ε-carboxymethyllysine in human breast milk, infant formulas, and urine of infants. J Agric Food Chem 54:6924–6928
Dittrich R, Dragonas C, Kannenkeril D, Hoffmann I, Mueller A, Beckmann MW, Pischetsrieder M (2009) A diet rich in Maillard reaction products protects LDL against copper induced oxidation ex vivo, a human intervention trial. Food Res Int 42:1315–1322
Dybing E, Farmer PB, Andersen M, Fennell TR, Lalljie SP, Müller DJ, Olin S, Petersen BJ, Schlatter J, Scholz G, Scimeca JA, Slimani N, Tornqvist M, Tuijtelaars S, Verger P (2005) Human exposure and internal dose assessments of acrylamide in food. Food Chem Toxicol 43:365–410
Erbersdobler HF, Faist V (2001) Metabolic transit of Amadori products. Nahrung 45:177–181
Erbersdobler HF, Somoza V (2007) Forty years of furosine—forty years of using Maillard reaction products as indicators of the nutritional quality of foods. Mol Nutr Food Res 51:423–430
Fenaille F, Parisod V, Visani P, Populaire S, Tabet JC, Guy PA (2006) Modification of milk constituents during processing: a preliminary benchmarking study. Int Dairy J 16:728–739
Förster A, Kühne Y, Henle T (2005) Studies on absorption and elimination of dietary Maillard reaction products. Ann NY Acad Sci 1043:474–481
Goldberg T, Cai W, Peppa M, Dardaine V, Baliga BS, Uribarri J, Vlassara H (2004) Advanced glycoxidation end products in commonly consumed foods. J Am Diet Assoc 104:1287–1291
Henle T (2003) AGEs in foods: do they play a role in uremia? Kidney Int 84:S145–S147
Henle T, Walter A, Haebner R, Klostermeryer H (1994) Detection and identification of a protein-bound imidazolone resulting from the reaction of arginine residues and methylglyoxal. Z Lebensm Unters Forsch 199:55–58
Hofmann SM, Dong HJ, Li Z, Cai W, Altomonte J, Thung SN, Zeng F, Fisher EA, Vlassara H (2002) Improved insulin sensitivity is associated with restricted intake of dietary glycoxidation products in the db/db mouse. Diabetes 51:2082–2089
Karachalias N, Babaei-Jadidi R, Rabbani N, Thornalley P (2010) Increased protein damage in renal glomeruli, retina, nerve, plasma and urine and its prevention by thiamine and benfotiamine therapy in a rat model of diabetes. Diabetologia 53:1506–1516
Knecht KJ, Dunn JA, Mcfarland KF, McCance DR, Lyons TJ, Thorpe SR, Baynes JW (1991) Effect of diabetes and aging on carboxymethyllysine levels in human urine. Diabetes 40:190–196
Koschinsky T, He CJ, Mitsuhashi T, Bucala R, Liu C, Buenting C, Heitmann K, Vlassara H (1997) Orally absorbed reactive glycation products (glycotoxins): an environmental risk factor in diabetic nephropathy. Proc Natl Acad Sci USA 94:6474–6479
Kroes R, Muller D, Lambe J, Lowik MRH, Klaveren J, Kleiner J, Massey R, Mayer S, Urieta I, Verger P, Visconti A (2002) Assessment of intake from the diet. Food Chem Toxicol 40:327–385
Liardon L, De Weck-Gaudard D, Philippossian G, Finot P-A (1987) Identification of Nepsilon-carboxymethyllysine: a new Maillard reaction product in rat urine. J Agric Food Chem 35:427–431
Lin RY, Choudhury RP, Cai W, Lu M, Fallon JT, Fisher EA, Vlassara H (2003) Dietary glycotoxins promote diabetic atherosclerosis in apolipoprotein E-deficient mice. Atherosclerosis 168:213–220
Marko D, Habermeyer M, Kemeny M, Weyand U, Niederberger E, Frank O, Hofmann T (2003) Maillard reaction products modulating the growth of human tumor cells in vitro. Chem Res Toxicol 16:48–55
Meng J, Sakata N, Takebayashi S, Asano T, Futata T, Nagai R, Ikeda K, Horiuchi S, Myint T, Taniguchi N (1998) Glycoxidation in aortic collagen from STZ-induced diabetic rats and its relevance to vascular damage. Atherosclerosis 136:355–365
Miyata T, Ueda Y, Horie K, Nangaku M, Tanaka S, de Strihou C, Kurokawa K (1998) Renal catabolism of advanced glycation end products: the fate of pentosidine. Kidney Int 53:416–422
Morales FJ (2009) Hydroxymethylfurfural (HMF) and related compounds. In: Stadler RH, Lineback DR (eds) Process-induced food toxicants: occurrence, formation, mitigation, and health risks. Wiley, New York, pp 135–174
Mucci LA, Wilson KM (2008) Acrylamide intake through diet and human cancer risk. J Agric Food Chem 56:6013–6019
Negrean M, Stirban A, Stratmann B et al (2007) Effects of low- and high-advanced glycation endproduct meals on macro- and microvascular endothelial function and oxidative stress in patients with type 2 diabetes mellitus. Am J Clin Nutr 85:1236–1243
Peppa M, Brem H, Ehrlich P, Zhang JG, Cai W, Li Z, Croitoru A, Thung S, Vlassara H (2003) Adverse effects of dietary glycotoxins on wound healing in genetically diabetic mice. Diabetes 52:2805–2813
Pouillart P, Mauprivez H, Ait-Ameur L, Cayzeele A, Lecerf JM, Tessier FJ, Birlouez-Aragon I (2008) Strategy for the study of the health impact of dietary Maillard products in clinical studies: the example of the ICARE clinical study on health adults. Ann NY Acad Sci 1126:173–176
Rabbani N, Sebekova K, Sebekova K Jr, Heidland A, Thornalley PJ (2007) Protein glycation, oxidation and nitration free adduct accumulation after bilateral nephrectomy and ureteral ligation. Kidney Internat 72:1113–1121
Rérat A, Calmes R, Vaissade P, Finot P-A (2002) Nutritional and metabolic consequences of the early Maillard reaction of heat treated milk in the pig. Significance for man. Eur J Nutr 41:1–11
Rufian-Henares JA, Morales FJ (2007) Functional properties of melanoidins: in vitro antioxidant, antimicrobial and antihypertensive activities. Food Res Int 40:995–1002
Sandu O, Song K, Cai W, Zheng F, Uribarri J, Vlassara H (2005) Insulin resistance and type 2 diabetes in high-fat-fed mice are linked to high glycotoxin intake. Diabetes 54:2314–2319
Sebeková K, Saavedra G, Zumpe C, Somoza V, Klenovicsová K, Birlouez-Aragon I (2008) Plasma concentration and urinary excretion of N ε-(carboxymethyl)lysine in breast milk- and formula-fed infants. Ann NY Acad Sci 1126:177–180
Seiquer I, Diaz-Alguacil J, Delgado-Andrade C, Lopez-Frias M, Munoz Hoyos A, Galdo G, Navarro MP (2006) Diets rich in Maillard reaction products affect protein digestibility in adolescent males aged 11–14 y. Am J Clin Nutr 83:1082–1088
Somoza V, Wenzel E, Weiss C, Clawin-Radecker I, Grubel N, Erbersdobler HF (2006) Dose-dependent utilisation of casein-linked lysinoalanine, N(epsilon)-fructoselysine and N(epsilon)-carboxymethyllysine in rats. Mol Nutr Food Res 50:833–841
Svistounov D, Smedsrød B (2004) Hepatic clearance of advanced glycation end products (AGEs)-myth or truth? J Hepatol 41:1038–1040
Tareke E, Rydberg P, Karlsson P, Eriksson S, Törnqvist M (2002) Analysis of acrylamide, a carcinogen formed in heated foodstuffs. J Agric Food Chem 50:4998–5006
Tessier FJ, Niquet C, Rhazi L, Hedhili K, Navarro P, Seiquer I, Delgado-Andrade C (2010) N ε-Carboxymethyllysine: its origin in selected foods and its urinary and faecal excretions in healthy humans. In: Thomas MC, Forbes J (eds) The Maillard reaction. Interface between aging, nutrition and metabolism. Royal Society of Chemistry, London (in press)
Ulbricht RJ, Northup SJ, Thomas JA (1984) A review of 5-hydroxymethylfurfural (HMF) in parenteral solutions. Fundam Appl Toxicol 4:843–853
Uribarri J, Peppa M, Cai W, Goldberg T, Lu M, He C, Vlassara H (2003a) Restriction of dietary glycotoxins reduces excessive advanced glycation end products in renal failure patients. J Am Soc Nephrol 14:728–731
Uribarri J, Peppa M, Cai W, Goldberg T, Lu M, Baliga S, Vassalotti JA, Vlassara H (2003b) Dietary glycotoxins correlate with circulating advanced glycation end product levels in renal failure patients. Am J Kidney Dis 42:532–538
Uribarri J, Cai W, Peppa M, Goodman S, Ferrucci L, Striker G, Vlassara H (2007) Circulating glycotoxins and dietary advanced glycation endproducts: two links to inflammatory response, oxidative stress, and aging. J Gerontol A Biol Sci Med Sci 62:427–433
Uribarri J, Woodruff S, Goodman S, Cai W, Chen X, Pyzik R, Yong A, Striker GE, Vlassara H (2010) Advanced glycation end products in foods and a practical guide to their reduction in the diet. J Am Diet Assoc 110:911–916
Utsunomiya N, Chuyen NV, Kato H (1990) Nutritional and physiological effects of casein modified by glucose, diacetyl, or hexanal. J Nutr Sci Vitaminol 36:387–397
Vlassara H (2005) Advanced glycation in health and disease. Role of the modern environment. Ann NY Acad Sci 1043:452–460
Vlassara H, Cai W, Crandall J, Goldberg T, Oberstein R, Dardaine V, Peppa M, Rayfield EJ (2002) Inflammatory mediators are induced by dietary glycotoxins, a major risk factor for diabetic angiopathy. Proc Natl Acad Sci USA 99:15596–15601
Wadman SK, De Bree PK, Van Sprang FJ, Kamerling JP, Haverkamp J, Vliegenthart JFG (1975) N ε-(Carboxymethyl)lysine, a constituent of human urine. Clin Chim Acta 59:313–320
Zheng F, He C, Cai W, Hattori M, Steffes M, Vlassara H (2002) Prevention of diabetic nephropathy in mice by a diet low in glycoxidation products. Diabetes Metab Res Rev 18:224–237
Author information
Authors and Affiliations
Corresponding author
Rights and permissions
About this article
Cite this article
Tessier, F.J., Birlouez-Aragon, I. Health effects of dietary Maillard reaction products: the results of ICARE and other studies. Amino Acids 42, 1119–1131 (2012). https://doi.org/10.1007/s00726-010-0776-z
Received:
Accepted:
Published:
Issue Date:
DOI: https://doi.org/10.1007/s00726-010-0776-z