Abstract
Silica-supported molybdic acid was employed as novel, recyclable, and safe catalyst in a one-pot three-component condensation of aldehydes, malononitrile, and 4-hydroxycoumarin to produce new and known pyrano[2,3-c]chromenes as potent biologically active compounds. This expedient new route has advantages, such as the use of a safe and reusable catalyst, simple operation, short reaction times, and good to excellent yields. The silica-supported molybdic acid as a novel solid acid was characterized by X-ray fluorescence, X-ray diffraction, and Fourier transform infrared spectroscopy.
Graphical abstract

Similar content being viewed by others
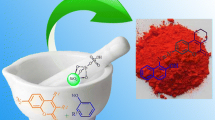
Explore related subjects
Discover the latest articles, news and stories from top researchers in related subjects.Avoid common mistakes on your manuscript.
Introduction
Multicomponent reactions are one of the most interesting concepts in modern synthetic chemistry [1]. Multicomponent reactions (MCRs), defined as one-pot reactions in which at least three different substrates join through covalent bonds, have steadily gained importance in synthetic organic chemistry. MCRs allow the creation of several bonds in a single operation and offer remarkable advantages like convergence, operational simplicity, facile automation, reduction in the number of work-up, extraction, and purification processes, and hence minimize waste generation, rendering the transformations green [2]. Development of MCRs can lead to new efficient synthetic methodologies to afford many small organic compounds in the field of modern organic, bioorganic, and medicinal chemistry [3]. Hence, MCRs are considered as a pivotal theme in the synthesis of many important heterocyclic compounds such as chromene derivatives nowadays [4]. Pyrano[3,2-c]chromene is a class of vital heterocycles with a wide range of biological effects such as spasmolytic, diuretic, anticoagulant, anticancer, and anti-anaphylactic activity [5]. Compounds having dihydropyran structural motif exhibit a wide range of biological activities, such as diuretic, analgesic, myorelaxant activity [6], anticoagulant [7], anticancer [8], anti-tumoral [9], and anti-HIV [10]. In addition, they are also useful for the treatment of neurodegenerative disorders including Alzheimer’s disease, amyotrophic lateral sclerosis, Huntington’s disease, and Parkinson’s disease [11]. Moreover, they are also used as cosmetics, pigments [12], and useful as photoactive materials [13]. A considerable effort has been made for the synthesis of pyran-annulated heterocyclic derivatives due to their wide applications [14].
A literature survey shows that several modified methods have been reported using different homogeneous or heterogeneous catalysts such as cetyltrimethylammonium chloride/bromide [15, 16], tetrabutylammonium bromide (TBAB) [17], triethylbenzylammonium chloride (TEBA) [18], chitosan [19], K3PO4 [20], Na2CO3 under grinding [21], Mg/Al hydrotalcite [22], [BMIm]BF4 [23], [2-aemim][PF6] [24], DBU [25], and piperidine under microwave irradiation [26]. However, many proposed methods for the synthesis of these compounds suffer from disadvantages including relying on multi-step conditions, the use of toxic organic solvents or catalysts containing transition metals, tedious work-up procedure, troublesome waste discarding, high reaction time, and low yields [27]. Thus, obviation of these limitations is necessary to develop a simple and green synthesis of 2-amino-4H-chromenes.
In this work, a new methodology to obtain novel and known pyrano[2,3-c]chromenes, via a one-pot three-component condensation, is reported. In this paper, we introduce silica-supported molybdic acid (SSMA) as a novel and safe catalyst for the synthesis of novel and known pyranocoumarin derivatives.
Results and discussion
In continuation of our previous studies on the development of various catalysts in synthesis of organic compounds [29–31], as can be seen in Scheme 1, from the reaction of readily available materials such as silicagel and thionyl chloride, silica chloride 1 has been prepared [32]. Accordingly, we found that anhydrous sodium molybdate can react with 1 to give silica-supported molybdic acid (SSMA, 2). This reaction is clean and easy. From the synthetic point of view, the nucleophilic substitution at silicon is also attractive.

SSMA was characterized by X-ray fluorescence (XRF), X-ray diffraction (XRD), and FT-IR spectra. As can be seen in Table 1, XRF data for the SSMA show the composition of the catalyst as 71.14 (%W/W) SiO2 and 25.62 (%W/W) MoO4.
Figure 1 shows the XRD patterns for the silica molybdic acid which exhibits the presence of molybdic acid crystalline phase supported on amorphous silica as a broad peak around 23° (2θ). The three peaks in the 35–40° region of the XRD spectrum could be attributed to the presence and linking of MoO3 to the silicagel [33].
The FT-IR spectra for the anhydrous sodium molybdate, silica chloride, and silica-supported molybdic acid are shown in Fig. 2. This spectrum shows the characteristic bonds of anhydrous sodium molybdate and silica chloride. The adsorption in 3452, 1634, 1086, 800 cm−1 in the catalyst spectrum reveals both bonds in SiO2–Cl and MoO4 groups.
The successful incorporation of molybdate groups was also confirmed by EDX analysis (Fig. 3), which showed the presence of Mo in addition to Si and O elements.
We evaluated the amounts of molybdic acid supported on SiO2 using two methods, including (a) titration with 0.1 N NaOH (neutralization reaction) and (b) calculating the weight difference between primary solid acid loosed chloride and new silica-supported molybdic acid. After these experiments, we found that 1 g catalyst includes 0.04 g –OMoO3H. Regarding to the molecular weight of MoO4H (161 g), therefore, 1 g of catalyst is equal to 0.25 mmol.
In continuation of our efforts toward the development of novel, efficient, and green procedures for the synthesis of organic compounds [34–36] using safe catalysts [37–39], we turned our attention toward the three-component condensation of malononitrile (3), aromatic aldehydes 4, and 4-hydroxycoumarin (5) to produce pyrano[2,3-c]coumarins 6 in the presence of SSMA (Scheme 2).

In order to optimize the reaction conditions, we used both protic and aprotic solvents in the presence of various amounts of the SSMA in the reaction of benzaldehyde, 3, and 5 as a model to investigate the effects of the solvent and the catalyst for preparing compounds 6a. In this case, the substrates were mixed together in 10 cm3 of the solvent.
As shown in Table 2, we found that polar and protic solvents, such as H2O and EtOH or MeOH, afford better yields than aprotic ones and also an equal mixture of H2O and EtOH is the most effective solvent. The effect of temperature was also studied for this reaction and it was found that the reaction would be completed after 360 min at room temperature. However, the thermal-assisted model reaction is efficiently carried out by adding catalytic amounts of SSMA (5 mol%) in a mixture of EtOH and H2O (50/50).
After optimization of the reaction conditions, in order to extend the scope of this reaction, a wide range of aromatic aldehydes were used with 3 and 5 (Table 3). All the products were characterized by comparison of their spectra and physical data with those reported in the literature [37, 40–42].
Table 4 demonstrates the merit of this method for the synthesis of pyrano[2,3-c]coumarins in comparison with previously reported results. As it can be seen from Table 4, piperidine was employed as a basic catalyst under refluxing EtOH, but this method could not afford good yields (entry 1). In the case of (S)-proline (entry 4), the product was obtained in low yield in a long time. In other variations, such as DAHP (entry 3), KF-Al2O3 (entry 5), and TEBA (entry 6), the methods need too long reaction times. We believe that these reactions can be efficiently carried out under our suggested conditions with respect to reaction times and product yield.
A mechanistic rationale for the formation of pyranocoumarins 6 is suggested in Scheme 3. It seems that the reaction takes place in three steps. It is reasonable to assume that the initial event involves the generation of the arylmethylene via a Knoevenagel condensation of the aldehyde and malononitrile catalyzed by SSMA 2. In the next steps, a Michael-type addition to the arylmethylene and subsequent heterocyclization promoted by SSMA give the corresponding products 6.

The main disadvantage of many reported methods for these reactions is that the catalysts are destroyed in the work-up procedure and cannot be recovered or reused. In these processes, as outlined in Fig. 4, the SSMA showed recyclability up to six runs without any considerable loss in the product yield and its catalytic activity. The FT-IR spectra of the catalyst after six runs (Fig. 2) showed the same spectral fingerprint of the freshly prepared catalyst indicating the stability of the catalyst throughout the recycling experiment.
Experimental
The chemicals were purchased from Merck and Aldrich chemical companies. The silica chloride 1 was synthesized according to the published procedure [28]. The reactions were monitored by TLC (silicagel 60 F254, hexane : EtOAc). Fourier transform infrared (FT-IR) spectroscopy spectra were recorded on a Shimadzu-470 spectrometer, using KBr pellets and the melting points were determined on a KRUSS model instrument. 1H NMR spectra were recorded on a Bruker Avance II 400 NMR spectrometer at 400 MHz, in which DMSO-d 6 was used as solvent and TMS as the internal standard. X-ray diffraction (XRD) pattern was obtained by Philips X Pert Pro X diffractometer operated with a Ni filtered Cu Kα radiation source. X-ray fluorescence (XRF) spectroscopy was recorded by X-Ray Fluorescence Analyzer, Bruker, S4 Pioneer, Germany. The varioEl CHNS Isfahan Industrial University was used for elemental analysis.
Preparation of silica chloride 1
To an oven-dried (125 °C, vacuum) sample of silicagel 60 (10 g) in a round bottomed flask (250 cm3) equipped with a condenser and a drying tube, thionyl chloride (40 cm3) was added and the mixture in the presence of CaCl2 as a drying agent was refluxed for 48 h. The resulting white-grayish powder was filtered and stored in a tightly capped bottle [28].
Preparation of silica-supported molybdic acid (2)
To a mixture of 6.00 g silica chloride and 3.7 g sodium molybdate, 10 cm3 n-hexane was added. The reaction mixture was stirred under refluxing conditions (70 °C) for 4 h. After completion of the reaction, the reaction mixture was filtered and washed with distilled water, and dried and then stirred in the presence of 40 cm3 0.1 N HCl for 1 h. Finally, the mixture was filtered, washed with distilled water, and dried to afford SSMA.
Preparation of pyrano[2,3-c]coumarin derivatives 5 using SSMA
Malononitrile (3, 1.1 mmol), aromatic aldehyde 4 (1 mmol), 4-hydroxycoumarin (5, 1 mmol), and SSMA (2, 5 mol%) were added to a 10-cm3 mixture EtOH/H2O (50/50) in a 25-cm3 Pyrex flask and refluxed for an appropriate time (Table 3). The reaction progress was controlled by thin layer chromatography (TLC) using hexane/EtOAc (1:1). After completion of the reaction, the solvent was removed under vacuum, the crude products 6 were obtained after recrystallization from EtOH.
2-Amino-4-(4-isopropylphenyl)-3-cyano-4H,5H-pyrano[3,2-c]chromene-5-one (6m, C22H18N2O3)
IR (KBr): \(\overline{v}\) = 3389, 3310, 2201, 1713, 1671, 1606, 1374, 1049 cm−1; 1H NMR (DMSO-d 6 , 400 MHz): δ = 7.90 (dd, J = 6.5, 1.3 Hz, 1H), 7.73–7.69 (m, 1H), 7.51–7.44 (m, 2H), 7.38 (s, 2H), 7.19–7.15 (m, 4H), 4.41 (s, 1H), 2.84 (m, 1H), 1.17 (d, J = 6.9 Hz, 6H) ppm; 13C NMR (DMSO-d 6 , 100 MHz): δ = 159.54, 158.00, 157.95, 153.28, 152.08, 147.11, 140.70, 132.88, 127.45, 126.42, 124.65, 122.41, 119.29, 116.54, 112.95, 104.18, 58.03, 38.85, 36.50, 33.23, 23.79 ppm.
2-Amino-4-cyclohexyl-3-cyano-4H,5H-pyrano[3,2-c]chromene-5-one (6n, C19H18N2O3)
IR (KBr): \(\overline{v}\) = 3427, 3280, 2188, 1720, 1669, 1594, 1389, 1048 cm−1; 1H NMR (DMSO-d 6 , 400 MHz): δ = 7.82 (dd, J = 6.4, 1.4 Hz, 1H), 7.72–7.67 (m, 1H), 7.48–7.43 (m, 2H), 7.35 (s, 2H), 4.43 (s, 1H), 1.74 (m, 1H), 1.63–1.57 (m, 4H), 1.38–1.32 (m, 2H), 1.18–0.94 (m, 4H) ppm; 13C NMR (DMSO-d 6 , 100 MHz): δ = 160.64, 160.06, 154.63, 152.02, 132.67, 124.56, 122.05, 116.53, 116.00, 113.00, 104.60, 52.48, 43.18, 36.66, 30.51, 27.52, 26.11, 25.85, 25.52 ppm.
References
Estevez V, Villacampa M, Menendez JC (2010) Chem Soc Rev 39:4402
Gu Y (2012) Green Chem 14:2091
Elinson MN, Ilovaisky AI, Merkulova VM, Belyakov PA, Chizhov AO (2010) Tetrahedron 66:4043
Dekamin MG, Eslami M, Maleki A (2013) Tetrahedron 69:1074
Mungra DC, Patel MP, Rajani DP, Patel RG (2011) Eur J Med Chem 46:4192
Bonsignore L, Loy G, Secci D, Calignano A (1993) Eur J Med Chem 28:517
Cingolani GM, Gualtteri F, Pigin M (1961) J Med Chem 12:531
Wu JYC, Fong WF, Zhang JX, Leung CH, Kwong HL, Yang MS, Li D, Cheung HY (2003) Eur J Pharmacol 473:9
Perrella FW, Chen SF, Behrens DL, Kaltenbach RF, Seitz SP (1994) J Med Chem 37:2232
Kashman Y, Gustafson KR, Fuller RW, Cardellina JH, McMahon JB, Currens MJ, Buckheit RW, Hughes SH, Gragg GM, Boyd MR (1992) J Med Chem 35:2735
Andreani LL, Lapi E (1960) Chem Pharm Bull 99:583
Ellis GP (1977) The chemistry of heterocyclic compounds. Chromenes, chromanes and chromones. John Wiley, New York
Arnesto D, Horspool WM, Martin N, Ramos A, Seaone C (1989) J Org Chem 54:3069
Khurana JM, Nand B, Saluja P (2010) Tetrahedron 66:5637
Jin TS, Xiao JC, Wang SJ, Li TS (2004) Ultrason Sonochem 11:393
Jin TS, Zhang JS, Liu LB, Wang AQ, Li TS (2006) Synth Commun 36:2009
Khurana JM, Kumar S (2009) Tetrahedron Lett 50:4125
Da-Qing S, Jing W, Qi-Ya Z, Xiang-Shan W (2006) Chin J Org Chem 26:643
Al-Matar M, Khalil KD, Meier H, Kolshorn H, Elnagdi MH (2008) Arkivoc 16:288
Zhou Z, Yang F, Wu L, Zhang A (2012) Chem Soc Trans 1:57
Naimi-jamal MR, Mashkouri S, Sharifi A (2010) Mol Divers 14:473
Surpur MP, Kshirsagar S, Samant S (2008) Tetrahedron Lett 50:719
Rad-Moghadam K, Yoseftabar-Miri L (2011) Tetrahedron 67:5693
Peng Y, Song G (2007) Catal Commun 8:111
Khurana MJ, Nand B, Saluja P (2010) Tetrahedron 66:5637
Raghuvanshi DS, Singh KN (2010) Arkivoc 10:305
Dekamin MG, Varmira K, Farahmand M, Sagheb-Asl S, Karimi Z (2010) Catal Commun 12:226
Cornelis A, Laszlo P (1985) Synthesis 10:909
Karami B, Kiani M (2010) Catal Commun 14:62
Karami B, Khodabakhshi S, Nikrooz N (2011) Polycycl Aromat Compd 31:97
Montazerozohori M, Karami B (2006) Helv Chim Acta 89:2922
Karade H, Sathe M, Kaushik MP (2007) Catal Commun 8:741
Brezesinski T, Wang J, Tolbert SH, Dunn B (2010) Nat Mater 9:146
Khodabakhshi S, Karami B, Baghernejad M (2014) Monatsh Chem 145:1839
Kamaei V, Karami B, Khodabakhshi S (2014) Polycycl Aromat Compd 34:1
Khodabakhshi S, Karami B, Eskandari K (2014) C R Chimie 17:35
Karami B, Khodabakhshi S, Hashemi F (2013) Tetrahedron Lett 54:3583
Karami B, Farahi M, Khodabakhshi S (2012) Helv Chim Acta 95:455
Karami B, Eskandari K, Khodabakhshi S (2012) Arkivoc 9:76
Wang HJ, Lu J, Zhang ZH (2010) Monatsh Chem 141:1107
Patra A, Mahapatra T (2012) J Indian Chem Soc 89:925
Khurana JM, Kumar S (2009) Tetrahedron Lett 50:4125
Greenwood NA, Earnshaw N (1984) Chemistry of the elements. Pergamon, Oxford
Mehrabi H, Abusaidi H (2010) J Iran Chem Soc 78:890
Abdolmohammadi S, Balalaie S (2007) Tetrahedron Lett 48:3299
Xiang-Shan W, Zhao-Sen Z, Da-Qing S, Xian-Yong W, Zhi-Min Z (2005) Chin J Org Chem 25:1138
Acknowledgments
We thank the Yasouj University for their support.
Author information
Authors and Affiliations
Corresponding author
Electronic supplementary material
Below is the link to the electronic supplementary material.
Rights and permissions
About this article
Cite this article
Karami, B., Kiani, M. Silica-supported molybdic acid: preparation, characterization, and its catalytic application in synthesis of pyranocoumarins. Monatsh Chem 147, 1117–1124 (2016). https://doi.org/10.1007/s00706-015-1551-3
Received:
Accepted:
Published:
Issue Date:
DOI: https://doi.org/10.1007/s00706-015-1551-3