Abstract
Background
Glomerular filtration rate (GFR) estimated by creatinine- and/or cystatin C-based equations (eGFR) is widely used in daily practice. The purpose of our study was to compare new and old eGFR equations with measured GFR (mGFR) by iohexol clearance in a cohort of children with chronic kidney disease (CKD).
Methods
We examined 96 children (median age 9.2 years (range 0.25–17.5)) with CKD stages 1–5. A 7-point iohexol clearance (GFR7p) was defined as the reference method (median mGFR 66 mL/min/1.73 m2, range 6–153). Ten different eGFR equations, with or without body height, were evaluated: Schwartzbedside, SchwartzCKiD, SchwartzcysC, CAPA, LMREV, (LMREV + CAPA) / 2, FAScrea, FAScysC, FAScombi, FASheight. The accuracy was evaluated with percentage within 10 and 30% of GFR7p (P10 and P30).
Results
In the group with mGFR below 60 mL/min/1.73 m2, the SchwartzcysC equation had the lowest median bias (interquartile range; IQR) 3.27 (4.80) mL/min/1.73 m2 and the highest accuracy with P10 of 44% and P30 of 85%. In the group with mGFR above 60 mL/min/1.73 m2, the SchwartzCKiD presented with the lowest bias 3.41 (13.1) mL/min/1.73 m2 and P10 of 62% and P30 of 98%. Overall, the SchwartzcysC had the lowest bias − 1.49 (13.5) mL/min/1.73 m2 and both SchwartzcysC and SchwartzCKiD showed P30 of 90%. P10 was 44 and 48%, respectively.
Conclusions
The SchwartzcysC and the combined SchwartzCKiD present with lower bias and higher accuracy as compared to the other equations. The SchwartzcysC equation is a good height-independent alternative to the SchwartzCKiD equation in children and can be reported directly by the laboratory information system.
Clinical Trial Registration
ClinicalTrials.gov, Identifier NCT01092260, https://clinicaltrials.gov/ct2/show/NCT01092260?term=tondel&rank=2
Similar content being viewed by others
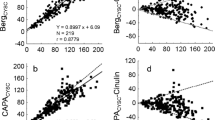
Avoid common mistakes on your manuscript.
Introduction
Glomerular filtration rate (GFR) is an important indicator of renal function in children and is widely used for classifying grade of kidney impairment [1, 2]. The gold standard for measurement of GFR (mGFR), renal inulin clearance, is often replaced by multipoint plasma clearance procedures with other markers than the hardly available inulin [3, 4]. Simplified mGFR methods using one or two blood samplings after marker injection have been shown to exhibit adequate performance for most clinical purposes [4,5,6,7]. To avoid time-consuming procedures in every day clinical practice, estimated GFR (eGFR) based on the natural biomarkers are widely used [1, 2].
Many different eGFR equations have been published, based on both plasma creatinine and cystatin C [8,9,10,11,12]. Schwartz et al. introduced two common ones; a combined equation with several parameters and the bedside equation based on only body height and serum creatinine [8, 9]. The plasma level of creatinine correlates with muscle mass [13, 14] and may be affected by the intake of protein [15], in addition to GFR. The biomarker cystatin C is less influenced by confounding factors and its plasma concentration remains relatively constant from approximately 1 year of age [16]. Cystatin C can thus be used as an alternative for estimating GFR in children and patients with abnormal muscle mass, or in other situations where renal tubular excretion of creatinine is modified (e.g., by the use of trimethoprim) [17, 18]. On the other hand, cystatin C may be influenced by factors such as corticosteroids and thyroid hormones [19,20,21,22]. The preferred choice is, according to guidelines, to use an eGFR value and not only to look at the absolute value of creatinine or cystatin C [23, 24]. This is easily done in adults because most parameters needed for GFR estimation are already present in the laboratory information systems. Several eGFR formulas for children are based on height, which is not readily available in the laboratory systems. During recent years, different height-independent eGFR equations have been published, e.g., FAS (full age spectrum) equations with or without cystatin C [10, 11].
The purpose of our study was to evaluate in total 10 formulae for eGFR: the four different FAS equations [10, 11, 25] (with or without cystatin C), the three commonly used Schwartz equations (with or without cystatin C) [8, 9], the CAPA (Caucasian, Asian, pediatric, and adult) equation based on cystatin C [12], the LMREV equation (Lund-Malmø revised) [26], and the LMREV-CAPA equation (mean of LMREV and CAPA) in a cohort of children at different stages of chronic kidney disease (CKD) and with different kidney disorders.
Patients and methods
Patients
A total of 96 patients with CKD were recruited at Haukeland University Hospital and Oslo University Hospital (ClinicalTrial.gov Identifier NCT01092260). The children had a stable kidney function without edema and were distributed evenly over the different GFR stages: 28, 27, 23, and 18 patients in CKD stages 1, 2, 3, and 4–5, respectively. The patients included had various kidney disorders; congenital anomalies of kidney and urinary tract (CAKUT) (n = 30), hereditary kidney disorder (n = 27), acquired kidney disorder (n = 12), glomerulonephritis (n = 9), hydronephrosis/vesicoureteral reflux (n = 9), and CKD of unknown etiology/miscellaneous (n = 9). Median (range) demographic characteristics were age 9.2 years (3 months to 17.5 years), weight 28.3 kg (6.6–84.6), and height 134 cm (59–177). Median multipoint mGFR was 65.9 (range 6.3–153) mL/min/1.73 m2. Forty-seven of the participants had proteinuria with urinary protein/creatinine ratio ≥ 30 mg/mmol, and seven of these had a ratio > 300 mg/mmol (Table 1). The study was approved by the Regional Ethics Committee of Western Norway and written informed consent was obtained in all cases before inclusion in the study. The procedures were in accordance with the Helsinki Declaration and Good Clinical Practice.
Methods
Iohexol was administrated via an intravenous cannula as Omnipaque® 300 mg I/mL (647 mg iohexol/mL, GE Healthcare, Oslo, Norway) in doses according to the patient’s weight; < 10 kg, 1 mL; 10–20 kg, 2 mL; 20–30 kg, 3 mL; 30–40 kg, 4 mL; ≥ 40 kg, 5 mL. Serum samples were collected from a vein of the contralateral arm of the iohexol injection at seven time points 10–300 min after injection for calculation of the seven-point reference mGFR (GFR7p), using the method of Sapirstein [27]. GFR was normalized to body surface area calculated by the method of Haycock [28]. Details about the procedures and methods are described in earlier studies published on the same cohort [5, 7, 29]. Serum creatinine was measured by an enzymatic colorimetric method (reagents from Roche Diagnostics®), IDMS traceable. The coefficient of variation (CV) (i.e., total analytical imprecision) was ≤ 3.7% and limit of detection was 5 μmol/L. Serum cystatin C was measured by a turbidimetric immunoassay, traceable to the ERM-DA471/IFCC reference material (reagents from Gentian, Moss, Norway). The CV was ≤ 5.0% and limit of detection was 0.03 mg/L. Serum urea was measured by a kinetic UV method. Urine protein and creatinine measurements were obtained in morning void urine. Urine protein was analyzed by an immunoturbidimetric method (CV ≤ 9%) and urine creatinine by an enzymatic colorimetric method (CV ≤ 3.7%). All markers were analyzed on Modular P8000 (Roche Diagnostics®).
Formulae
Table 2 describes the 10 different eGFR equations evaluated in this study: Schwartzbedside [8], SchwartzCKiD [9], SchwartzcysC [9], FAScrea [11, 25], FAScysC [10], FAScombi [10], FASheight [25], CAPA [12], LMREV [26], and the LMREV-CAPA (mean of LMREV and CAPA). The Schwartzbedside, SchwartzCKiD, and FASheight equations require a height measurement, but the SchwartzcysC, FAScrea, FAScysC, FAScombi, CAPA, LMREV, and the LMREV-CAPA equations are height-independent.
Statistics
The performance statistics are presented as bias, interquartile range (IQR), and accuracy. Bias was defined as the difference between the eGFR and mGFR, calculated as median absolute differences (mL/min/1.73 m2) in GFR. Precision was assessed as the IQR of the differences. Accuracy was defined by the percentage of eGFR results within 10 and 30% of the mGFR (mL/min/1.73 m2); P10 and P30. Confidence intervals were calculated by means of bootstraps methods with 1000 replicates. Bias, IQR, and accuracy were also evaluated in relation to two mGFR regions, below or above (including) 60 mL/min/1.73 m2. A priori we defined bias ± 3 mL/min/1.73 m2 as “clinically irrelevant.” Bland Altman plots, McNemars test with Holm-Bonferroni method for multiple comparisons for accuracy, and t tests with Holm-Bonferroni method for multiple comparisons for bias were used for comparison of estimates between the equations [30]. Subgroup analyses with different age groups and proteinuria/non-proteinuria were also performed. We used R version 3.3.2 for all statistical analyses and making figures.
Results
Patient characteristics are listed in Table 1. Median bias, IQR, and accuracy for the 10 tested equations are presented in Tables 3–4 and Figs. 1–2, both above and below mGFR 60 mL/min/1.73 m2. In the group with mGFR below 60 mL/min/1.73 m2, the SchwartzcysC equation had the lowest bias (IQR) 3.27 (4.80) mL/min/1.73 m2 and the highest accuracy with P10 of 44% and P30 of 85%. All other equations demonstrated P30 below 80% in this group. In the group with mGFR above 60 mL/min/1.73 m2, the SchwartzCKiD presented with the lowest bias (IQR) 3.41 (13.1), a P10 of 62% and P30 of 98%. In addition, the SchwartzcysC showed a P30 of 93%. The FAScysC, FAScombi, and LMREV had P30 below 90% (82, 82, and 87%, respectively). SchwartzcysC demonstrated a bias (IQR) of − 7.46 (14.8) mL/min/1.73 m2 (underestimation). The CAPA equation gave a bias of 7.45 (13.4) and 24.0 (33.4) mL/min/1.73 m2 below and above 60 mL/min/1.73 m2, respectively, and a P30 of 55% in the total group. For the whole group (n = 96), the SchwartzcysC had the lowest bias (IQR) − 1.49 (13.5) and both the SchwartzcysC and the SchwartzCKiD showed a P30 of 90%, whereas P10 was 44% and 48% respectively. All 10 equations overestimated eGFR as compared to mGFR, except the SchwartzcysC equation which gave an underestimation in the GFR level above 60 mL/min/1.73 m2 (Figs. 1–2). The SchwartzcysC formula also performed relatively well in the subgroup with proteinuria (n = 47) with bias 1.98 (7.16), and a P10 of 45% and P30 of 87%. In the group without proteinuria, the SchwartzCKiD formula had the lowest bias 3.84 (10.7) and a P10 of 55% and P30 of 98% (Fig. 3 and Online Resource 1). In the age group < 2 years, the SchwartzcysC showed the best results with bias 3.27 (5.37), and a P30 of 89% (Fig. 4 and Online Resource 2). This formula also performed relatively well in the age group from 2 to 12 years with bias − 1.46 (14.1) and P30 of 93%. The SchwartzCKiD formula also demonstrated a relatively high accuracy with P30 of 91%. In the group with adolescents (age > 13 years), the LMREV gave a very low bias − 0.21 (11.3) mL/min/1.73 m2 and a P30 of 89%, but also the SchwartzCKiD, SchwartzcysC, Schwartzbedside, CAPA, LMREV, and LMREV-CAPA had a bias < 3 mL/min/1.73 m2. The LMREV-CAPA and SchwartzCKiD had the highest accuracy in this group with P30 of 100 and 95%, respectively (Fig. 4 and Online Resource 2).
Scatter plots of estimated glomerular filtration rate (eGFR) and measured GFR (mGFR) in children. Correlation between the 10 different eGFR equations and mGFR in children with chronic kidney disease (CKD) (n = 96). The dark gray area represents P10 (the percentage of eGFR results within 10% of the mGFR (mL/min/1.73 m2) and the light gray area P30 (the percentage of eGFR results within 30% of the mGFR (mL/min/1.73 m2)). The black line represents the multipoint mGFR and the dashed line the regression line. The gray box represents the CKD stage 3 (30–60 mL/min/1.73 m2)
Bland Altman plots with absolute bias between estimated glomerular filtration rate (eGFR) and measured GFR (mGFR) for the different equations in children (n = 96) with chronic kidney disease (CKD). The solid line represents the regression line. The dashed line represents the median bias and the dotted line the 2.5–97.5% confidence interval for all points
Bland Altman plots with absolute bias between estimated glomerular filtration rate (eGFR) and measured GFR (mGFR) for the different equations in children (n = 96) with chronic kidney disease (CKD). The blue lines represent the group with proteinuria ≥ 30 mg/mmol (n = 47) and the red lines the group with non-proteinuria (n = 49). The solid lines represent the regression line, the dashed lines the median bias, and the dotted lines the 2.5–97.5% confidence interval for all points
Bland Altman plots with absolute bias between estimated glomerular filtration rate (eGFR) and measured GFR (mGFR) for the different equations in children (n = 96) with chronic kidney disease (CKD). The red lines, green lines, and blue lines represent the age group < 2 years (n = 9), 2–12 years (n = 68), and ≥ 13 years of age (n = 19), respectively. The solid lines represent the regression line, the dashed lines the median bias, and the dotted lines the 2.5–97.5% confidence interval for all points
Discussion
Among the 10 eGFR equations evaluated in this study, the SchwartzcysC and SchwartzCKiD equations were superior to the other eight eGFR equations and performed equally well with P30 of 90% for the total group (n = 96) (Figs. 1–2 and Table 3–4). Based on P30 and P10, the SchwartzcysC equation seemed to give a higher accuracy in the group with mGFR below 60 mL/min/1.73 m2 (P10 44% and P30 85%) and the SchwartzCKiD in the group with mGFR above 60 mL/min/1.73 m2 (P10 62% and P30 98%) (Table 4). The commonly used Schwartzbedside equation based solely on creatinine as endogenous metabolite showed low accuracy with P30 of 53% and a median bias of 15.5 mL/min/1.73 m2 for the total group of patients. The creatinine-based equation with the best accuracy was the LMREV equation with a P30 of 72% (Fig. 1 and Table 4). The new height-independent FAS equations showed lower accuracy and higher bias than the SchwartzcysC and the SchwartzCKiD equations, and the SchwartzcysC equation had the lowest bias of − 1.49 mL/min/1.73 m2 (Figs. 1–2 and Tables 3–4).
Most eGFR-studies state error as a percentage, that includes the majority of estimations, and a commonly used criterion is error within ± 30% compared with the gold standard, which allows for a quite wide limit. Ideally, the majority of the estimations (90%) should be included in a 10% difference limit of a gold standard method as recommended recently by Lima et al. [31]. A new review from Levey and Inker recommends, on the other hand, P30 of > 90% as an adequate standard [32]. In our study, the SchwartzcysC and the SchwartzCKiD equations were the only formulae with P30 > 90% in the complete cohort, but in the group with mGFR < 60 mL/min/1.73 m2 none of the formulas fulfilled these requirements (Table 4). In the adolescent group, however, the SchwartzcysC equation demonstrated a P30 of just 79% (Online Resource 2).
The SchwartzcysC formula performed best when mGFR was < 60 mL/min/1.73 m2 and the SchwartzCKiD when mGFR was > 60 mL/min/1.73 m2. All the FAS equations were inferior to these equations (Figs. 1–2 and Tables 3–4) with P30 between 56 and 66%, and bias between 10.4 and 13.3 mL/min/1.73 m2. Pottel et al. recently published a study on new equations; FAScysC and FAScombi, where they in contrast to us found better performance in children and adolescents with FAScysC than with CAPA or SchwartzcysC (P30 86.1, 76.6, and 68.8%, respectively) [10]. When mGFR was below 60 mL/min/1.73 m2, however, their results were in accordance with our study where the SchwartzcysC equation gave the lowest bias and highest accuracy; bias of − 2.4 mL/min/1.73 m2 and P30 of 86% in their study and 3.27 mL/min/1.73 m2 and 85% in our study (Tables 3–4). The FAScombi outperformed all equations included in the Pottel study, with a P30 of 92.1%; however, the SchwartzCKiD equation was not included in their evaluation [10].
In our study, the SchwartzCKiD performed relatively well with P30 of 90%, but the other combined equations, LMREV-CAPA and FAScombi, did not have acceptable accuracy (P30 of 65 and 66%, respectively) (Table 4). A recent study from the Schwartz-group compared Schwartzbedside and SchwartzCKiD with mGFR by iohexol plasma clearance in 730 children and adolescents, and reported that SchwartzCKiD closely approximated mGFR to describe relationships with CKD severity indicators and progression, and concluded that this formula should be the preferred eGFR method for research studies of pediatric CKD [33]. Deng et al. published a study evaluating 14 published eGFR equations with iohexol-GFR. They found that the combined formulas with creatinine and cystatin C, including the SchwartzCKiD, outperformed equations that used serum creatinine or cystatin C alone. The SchwartzcysC equation also performed relatively well in their study with median bias 1.9 mL/min/1.73 m2 and P30 of 79% [34]. From others, it has previously been demonstrated that combination of creatinine and cystatin C for GFR estimation increases the performance [35, 36].
Overall, the height-independent SchwartzcysC equation showed better performance than the other height-independent formulas in our study (FAScrea, FAScombi, FAScysC, LMREV, CAPA, LMREV-CAPA) (Figs. 1–2 and Table 3–4). Another recent study found that by combining the height-independent FAScrea and SchwartzcysC equations, the accuracy improved and that these two equations performed comparably to height-dependent equations [37]. A study from 2018 recommends reporting eGFR by calculating the geometric mean between the height-independent FAScrea and FAScysC equations when ΔeGFR is smaller than 40%. If there was a large disagreement between the two, weighted means based on diagnosis improved accuracy [38]. This facilitates direct eGFR reporting by the laboratory information system.
As may be expected, eGFR formulas are shown to work better in the same kind of cohort from which they were developed [39]. Differences among equations using the same filtration markers reflect differences in the variables in the equations and their coefficients. For equations using different filtration markers, differences in the validation will on the other hand also reflect differences regarding biological variations, error in assay measurements, or the influence of non-GFR determinants [32]. The Schwartzbedside equation is similar to the two former equations, generated from the CKiD study. This study included patients from 1 to 16 years of age with eGFR in the range of 30–90 mL/min/1.73 m2 [8, 9]. The CAPA equation was developed from a cohort of children with median age (2.5th, 97.5th percentile) 12 (2.0, 17.5) years and median mGFR 103 mL/min/1.73 m2, with 88% of the mGFR > 60 mL/min/1.73 m2 [12]. The CAPA equation did not perform well in our study, neither below nor above mGFR 60 mL/min/1.73 m2, with a P30 of only 55% (Figs. 1–2, Tables 3–4). The FAScrea and FASheight were evaluated for subjects aged between 2 and 25 years [25, 40], and in our study, we included nine patients < 2 years. The SchwartzcysC equation was the only equation with P30 around 90% in patients below 2 years of age (Fig. 4 and Online Resource 2). The study of FAScysC and FAScombi equations included children between 1 and 18 years with mean (± SD) mGFR 89.2 (30.4) [10], but these equations showed low P30 in patients < 13 years of age in our study (Fig. 4 and Online Resource 2). LMREV formula was however developed in a cohort (n = 850) of Swedish adults (> 18 years), and in the present study, this formula performed best in the group of adolescents with P30 of 89% (Fig. 4 and Online Resource 2).
In our study, the highest P10 was 62% with the SchwartzCKiD formula in the group with mGFR > 60 mL/min/1.73 m2 (Table 4). eGFR equations are hampered by low accuracy compared to mGFR, and the preferred method should be to measure GFR, if possible. We have previously published studies on simplified mGFR methods with validation of multiple formulas, single-point and two-point sampling times as well as capillary blood spot method [5, 7, 41], and we found that the highest P10 for children with CKD stage 1–5 was 95.8%, using the formula of Jødal and Brøchner-Mortensen from 2009 with blood sampling 2 and 5 h after marker injection [5, 42]. Single-point sampling at 3 h using the Fleming formula [43] showed a P10 of 92.3% in children with GFR ≥ 30 mL/min/1.73 m2. For children with GFR < 30 mL/min/1.73 m2, two blood samples were needed to achieve sufficiently high accuracy [5, 7]. The use of simplified mGFR methods is of great clinical importance especially in patient groups where exact level and change in GFR are important to monitor and where muscle mass might change significantly due to disease, e.g., children with cancer. Oncology remains one important area where mGFR is still the gold standard instead of eGFR [18]. For clinical studies in children, simplified mGFR methods can also be an essential supplement to eGFR.
A limitation of our study is the relatively low number of patients, but on the other hand, there are few other studies with multipoint reference mGFR from children with CKD. One patient was younger than 1 year, but nine patients were < 2 years. Another limitation is that the last plasma sampling of mGFR at 5 h may be too early in patients with severely reduced kidney function. However, our study has a high number of blood samples at different time points obtained during daytime at the outpatient clinic, and multipoint iohexol clearance has proven to be close to the gold standard inulin clearance [1]. The creatinine and cystatin C were calibrated against a standardized reference method. However, important differences still remain despite the fact that only since 2010, a certified reference material ERM-DA471/IFCC has been available for cystatin C [44].
In conclusion, we recommend the SchwartzcysC and the SchwartzCKiD as preferred eGFR equations when mGFR is not performed in children with CKD. In addition, the height-independent SchwartzcysC equation can be implemented to be reported directly by most laboratory information systems. The commonly used creatinine-based equations, like Schwartzbedside equation, showed relatively low accuracy.
References
Schwartz GJ, Work DF (2009) Measurement and estimation of GFR in children and adolescents. Clin J Am Soc Nephrol 4:1832–1843
Pottel (2017) Measuring and estimating glomerular filtration rate in children. Pediatr Nephrol 32:249–263
Delanaye P, Ebert N, Melsom T, Gaspari F, Mariat C, Cavalier E, Bjork J, Christensson A, Nyman U, Porrini E, Remuzzi G, Ruggenenti P, Schaeffner E, Soveri I, Sterner G, Eriksen BO, Back SE (2016) Iohexol plasma clearance for measuring glomerular filtration rate in clinical practice and research: a review. Part 1: how to measure glomerular filtration rate with iohexol? Clin Kidney J 9:682–699
McMeekin H, Wickham F, Barnfield M, Burniston M (2016) A systematic review of single-sample glomerular filtration rate measurement techniques and demonstration of equal accuracy to slope-intercept methods. Nucl Med Commun 37:743–755
Tøndel C, Bolann B, Salvador CL, Brackman D, Bjerre A, Svarstad E, Brun A (2017) Iohexol plasma clearance in children: validation of multiple formulas and two-point sampling times. Pediatr Nephrol 32:311–320
Schwartz GJ, Furth S, Cole SR, Warady B, Munoz A (2006) Glomerular filtration rate via plasma iohexol disappearance: pilot study for chronic kidney disease in children. Kidney Int 69:2070–2077
Tøndel C, Salvador CL, Hufthammer KO, Bolann B, Brackman D, Bjerre A, Svarstad E, Brun A (2018) Iohexol plasma clearance in children: validation of multiple formulas and single-point sampling times. Pediatr Nephrol 33:683–696
Schwartz GJ, Munoz A, Schneider MF, Mak RH, Kaskel F, Warady BA, Furth SL (2009) New equations to estimate GFR in children with CKD. J Am Soc Nephrol 20:629–637
Schwartz GJ, Schneider MF, Maier PS, Moxey-Mims M, Dharnidharka VR, Warady BA, Furth SL, Munoz A (2012) Improved equations estimating GFR in children with chronic kidney disease using an immunonephelometric determination of cystatin C. Kidney Int 82:445–453
Pottel H, Delanaye P, Schaeffner E, Dubourg L, Eriksen BO, Melsom T, Lamb EJ, Rule AD, Turner ST, Glassock RJ, De Souza V, Selistre L, Goffin K, Pauwels S, Mariat C, Flamant M, Ebert N (2017) Estimating glomerular filtration rate for the full age spectrum from serum creatinine and cystatin C. Nephrol Dial Transplant 32:497–507
Pottel H, Hoste L, Dubourg L, Ebert N, Schaeffner E, Eriksen BO, Melsom T, Lamb EJ, Rule AD, Turner ST, Glassock RJ, De Souza V, Selistre L, Mariat C, Martens F, Delanaye P (2016) An estimated glomerular filtration rate equation for the full age spectrum. Nephrol Dial Transplant 31:798–806
Grubb A, Horio M, Hansson LO, Bjork J, Nyman U, Flodin M, Larsson A, Bokenkamp A, Yasuda Y, Blufpand H, Lindstrom V, Zegers I, Althaus H, Blirup-Jensen S, Itoh Y, Sjostrom P, Nordin G, Christensson A, Klima H, Sunde K, Hjort-Christensen P, Armbruster D, Ferrero C (2014) Generation of a new cystatin C-based estimating equation for glomerular filtration rate by use of 7 assays standardized to the international calibrator. Clin Chem 60:974–986
Perrone RD, Madias NE, Levey AS (1992) Serum creatinine as an index of renal function: new insights into old concepts. Clin Chem 38:1933–1953
Heymsfield SB, Arteaga C, McManus C, Smith J, Moffitt S (1983) Measurement of muscle mass in humans: validity of the 24-hour urinary creatinine method. Am J Clin Nutr 37:478–494
Mayersohn M, Conrad KA, Achari R (1983) The influence of a cooked meat meal on creatinine plasma concentration and creatinine clearance. Br J Clin Pharmacol 15:227–230
Bokenkamp A, Domanetzki M, Zinck R, Schumann G, Brodehl J (1998) Reference values for cystatin C serum concentrations in children. Pediatr Nephrol 12:125–129
Delanaye P, Mariat C, Cavalier E, Maillard N, Krzesinski JM, White CA (2011) Trimethoprim, creatinine and creatinine-based equations. Nephron Clin Pract 119:c187–c193 discussion c193–194
Filler G, Lee M (2017) Educational review: measurement of GFR in special populations. Pediatr Nephrol https://doi.org/10.1007/s00467-017-3852-8
Fricker M, Wiesli P, Brandle M, Schwegler B, Schmid C (2003) Impact of thyroid dysfunction on serum cystatin C. Kidney Int 63:1944–1947
Risch L, Herklotz R, Blumberg A, Huber AR (2001) Effects of glucocorticoid immunosuppression on serum cystatin C concentrations in renal transplant patients. Clin Chem 47:2055–2059
Simeoni M, Cerantonio A, Pastore I, Liguori R, Greco M, Foti D, Gulletta E, Brunetti A, Fuiano G (2016) The correct renal function evaluation in patients with thyroid dysfunction. J Endocrinol Investig 39:495–507
Risch L, Huber AR (2002) Glucocorticoids and increased serum cystatin C concentrations. Clin Chim Acta 320:133–134
Levey AS, Coresh J, Balk E, Kausz AT, Levin A, Steffes MW, Hogg RJ, Perrone RD, Lau J, Eknoyan G (2003) National Kidney Foundation practice guidelines for chronic kidney disease: evaluation, classification, and stratification. Ann Intern Med 139:137–147
Kidney Disease: Improving Global Outcomes (KDIGO) CKD Work Group (2013) KDIGO 2012 clinical practice guideline for the evaluation and management of chronic kidney disease. Kidney Int Suppl 3:1–150
Hoste L, Dubourg L, Selistre L, De Souza VC, Ranchin B, Hadj-Aissa A, Cochat P, Martens F, Pottel H (2014) A new equation to estimate the glomerular filtration rate in children, adolescents and young adults. Nephrol Dial Transplant 29:1082–1091
Bjork J, Grubb A, Sterner G, Nyman U (2011) Revised equations for estimating glomerular filtration rate based on the Lund-Malmo Study cohort. Scand J Clin Lab Invest 71:232–239
Sapirstein LA, Vidt DG, Mandel MJ, Hanusek G (1955) Volumes of distribution and clearances of intravenously injected creatinine in the dog. Am J Phys 181:330–336
Haycock GB, Schwartz GJ, Wisotsky DH (1978) Geometric method for measuring body surface area: a height-weight formula validated in infants, children, and adults. J Pediatr 93:62–66
Salvador CL, Tøndel C, Rowe AD, Bjerre A, Brun A, Brackman D, Bolstad N, Mørkrid L (2017) Renal function influences diagnostic markers in serum and urine: a study of guanidinoacetate, creatine, human epididymis protein 4, and neutrophil gelatinase–associated lipocalin in children. J Appl Lab Med 2:297–308. https://doi.org/10.1373/jalm.2016.022145
Holm S (1979) A simple sequentially rejective multiple test procedure. Scand J Stat 6:65–70
Luis-Lima S, Marrero-Miranda D, Gonzalez-Rinne A, Torres A, Gonzalez-Posada JM, Rodriguez A, Salido E, Aldea-Perona A, Gaspari F, Carrara F, Gomez-Gerique JA, Negrin-Mena N, Perez-Tamajon L, Gonzalez-Rinne F, Jimenez-Hernandez H, Jimenez-Sosa A, Porrini E (2015) Estimated glomerular filtration rate in renal transplantation: the nephrologist in the mist. Transplantation 99:2625–2633
Levey AS, Inker LA (2017) Assessment of glomerular filtration rate in health and disease: a state of the art review. Clin Pharmacol Ther 102:405–419
Ng DK, Schwartz GJ, Warady BA, Furth SL, Munoz A (2017) Relationships of measured iohexol GFR and estimated GFR with CKD-related biomarkers in children and adolescents. Am J Kidney Dis 70:397–405
Deng F, Finer G, Haymond S, Brooks E, Langman CB (2015) Applicability of estimating glomerular filtration rate equations in pediatric patients: comparison with a measured glomerular filtration rate by iohexol clearance. Transl Res 165:437–445
Grubb A, Nyman U, Bjork J (2012) Improved estimation of glomerular filtration rate (GFR) by comparison of eGFRcystatin C and eGFRcreatinine. Scand J Clin Lab Invest 72:73–77
Leion F, Hegbrant J, den Bakker E, Jonsson M, Abrahamson M, Nyman U, Bjork J, Lindstrom V, Larsson A, Bokenkamp A, Grubb A (2017) Estimating glomerular filtration rate (GFR) in children. The average between a cystatin C- and a creatinine-based equation improves estimation of GFR in both children and adults and enables diagnosing Shrunken Pore Syndrome. Scand J Clin Lab Invest 77:338–344
den Bakker E, Gemke R, van Wijk JAE, Hubeek I, Stoffel-Wagner B, Grubb A, Bokenkamp A (2017) Accurate eGFR reporting for children without anthropometric data. Clin Chim Acta 474:38–43
den Bakker E, Gemke R, van Wijk JAE, Hubeek I, Stoffel-Wagner B, Bökenkamp A (2018) Combining GFR estimates from cystatin C and creatinine-what is the optimal mix? Pediatr Nephrol 33:1553–1563
Pottel H, Dubourg L, Goffin K, Delanaye P (2018) Alternatives for the bedside Schwartz equation to estimate glomerular filtration rate in children. Adv Chronic Kidney Dis 25:57–66
Pottel H, Hoste L, Martens F (2012) A simple height-independent equation for estimating glomerular filtration rate in children. Pediatr Nephrol 27:973–979
Salvador CL, Tøndel C, Mørkrid L, Bjerre A, Brun A, Bolann B, Brackman D, Bergan S (2015) Glomerular filtration rate measured by iohexol clearance: a comparison of venous samples and capillary blood spots. Scand J Clin Lab Invest 75:710–716
Jødal L, Brøchner-Mortensen J (2009) Reassessment of a classical single injection 51Cr-EDTA clearance method for determination of renal function in children and adults. Part I: analytically correct relationship between total and one-pool clearance. Scand J Clin Lab Invest 69:305–313
Fleming JS, Persaud L, Zivanovic MA (2005) A general equation for estimating glomerular filtration rate from a single plasma sample. Nucl Med Commun 26:743–748
Bargnoux AS, Pieroni L, Cristol JP, Kuster N, Delanaye P, Carlier MC, Fellahi S, Boutten A, Lombard C, Gonzalez-Antuna A, Delatour V, Cavalier E (2017) Multicenter evaluation of cystatin C measurement after assay standardization. Clin Chem 63:833–841
Acknowledgements
We wish to express our gratitude to the pediatric study nurses Mai Britt Lynum (Oslo University Hospital), Hildur Grindheim, and Renathe Håpoldøy (Haukeland University Hospital) for their technical assistance with the sample collection and to the laboratory engineer Kjersti Carstensen for the iohexol analyses (Haukeland University Hospital). The study was supported by grants from the Health Trust of Western Norway, The Norwegian Society of Nephrology, Haukeland University Hospital, and Oslo University Hospital.
Author information
Authors and Affiliations
Corresponding author
Ethics declarations
Financial disclosure
The authors have no financial relationships relevant to this article to disclose.
Conflict of interest
The authors declare that they have no conflict of interest.
Ethical approval
The study was approved by the Regional Ethics Committee of Western Norway and an informed consent form was obtained by all patients and/or their designees. The study was performed in accordance with the Declaration of Helsinki.
Electronic supplementary material
ESM 1
(PDF 268 kb)
Rights and permissions
About this article
Cite this article
Salvador, C.L., Tøndel, C., Rowe, A.D. et al. Estimating glomerular filtration rate in children: evaluation of creatinine- and cystatin C-based equations. Pediatr Nephrol 34, 301–311 (2019). https://doi.org/10.1007/s00467-018-4067-3
Received:
Revised:
Accepted:
Published:
Issue Date:
DOI: https://doi.org/10.1007/s00467-018-4067-3