Abstract
Background
The introduction of robotic technology has revolutionized radical prostatectomy surgery. However, the potential benefits of robotic techniques may have trade-offs in increased mental demand for the surgeon and the physical demand for the assisting surgeon. This study employed an innovative motion tracking tool along with validated workload questionnaire to assess the ergonomics and workload for both assisting and console surgeons intraoperatively.
Methods
Fifteen RARP cases were collected in this study. Cases were performed by 10 different participants, six primarily performed console tasks and four primarily performed assisting tasks. Participants had a median 12 (min—3, max—25) years of surgical experience. Both console and assisting surgeons performed robotic prostatectomy cases while wearing inertial measurement units (IMUs) that continuously track neck, shoulder, and torso motion without interfering with the sterile environment. Postoperatively, participants completed a workload questionnaire (SURG-TLX) and a body part discomfort questionnaire.
Results
Twenty-six questionnaires were completed from 13 assisting and 13 console surgeons over the 15 cases. Postoperative pain was reported highest for the right shoulder and neck. Mental demands were 41 % higher for surgeons at the console than assisting (p < 0.05), while physical demands were not significantly different. Assisting surgeons worked in demanding neck postures for 58 % of the procedure compared to 24 % for the console surgeon (p < 0.01). Surgeons at the console were primarily static and showed 2–5 times fewer movements than assisting surgeons (p < 0.01).
Conclusions
Postures were more ergonomic during console tasks than when assisting by the bedside; however, the console may constrain postures leading to static loads that have been associated with musculoskeletal symptoms for the neck, torso, and shoulders. The IMU sensors were effective at quantifying ergonomics in robotic prostatectomies, and these methods and findings have broad applications to other robotic procedures.
Similar content being viewed by others
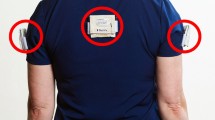
Avoid common mistakes on your manuscript.
The introduction of robotic techniques has revolutionized radical prostatectomy surgery service delivery. In the USA between 2003 and 2010, robotic-assisted radical prostatectomy (RARP) adoption increased from 0.7 to 42 % of surgeons performing radical prostatectomy [1]. RARP provides surgeons with improved anatomical vision and more precise instrument control compared to open or laparoscopic techniques [2–6]. Recent meta-analyses have concluded that a robotic approach results in improved functional outcomes with better potency rates and urinary continence at 12 months compared to open radical prostatectomy (ORP) and laparoscopic radical prostatectomy (LRP) [7, 8]. Blood loss and transfusion rates are also reduced with RARP, although the impact of RARP on overall oncological outcomes remains inconclusive [9, 10]. It is also recognized that RARP results in an increased economic burden for prostate cancer surgery due to increased technology and operative duration costs [1]. Despite additional costs of the surgery, investigators generally agree that robotic techniques improve surgeon ergonomics by providing increased dexterity (e.g., 3 vs. 9 degrees of freedom) than standard laparoscopy, a seated position, armrests, and stereoscopic vision [3, 11–14]. These improvements are a potential key innovation for addressing the pressing issue of ergonomics and musculoskeletal symptoms that impact surgeon health and operative performance that has been attributed to minimally invasive surgeries performed with laparoscopy techniques [15, 16].
Musculoskeletal symptoms have been widely reported among surgeons in large survey studies conducted across specialties. Many studies have suggested that the prevalence of musculoskeletal symptoms is as high as 87 % in surgeons who perform minimally invasive procedures [17, 18], with 40 % of surgeons experiencing at least one work-related injury which have noticeable impact on surgical performance [19]. Key contributors to musculoskeletal symptoms in laparoscopy include tool and equipment design, port placement, video monitor position, and patient position [16, 20–22]. These detrimental effects may be reduced with robotic technology; however, intraoperative evidence measuring the ergonomics of robotic techniques is currently limited.
A few studies comparing robotic with laparoscopic techniques have found that robotic techniques lowered mental and physical workload, reduced muscle activity, and decreased awkward postures (e.g., shoulder abduction that increases shoulder joint loads) [23–26]. However, critical limitations to these findings are that they were performed either by novices, on simulated skill tasks, or in a controlled and timed animal laboratory setting. Intraoperative measurements by an observer using ergonomic worksheets found robotic surgery was rated as hazardous to the surgeon and called for interventions to address observed ergonomic deficits in robotic console surgery [27]. In addition, no previous study has examined the impact of robotic techniques on the surgical assistant, who in addition to performing laparoscopic surgery may need to assume awkward positions to maneuver around the intermittent movements of the robotic arms to perform the required roles of the bedside surgeon (Fig. 1). These work conditions have potential to transfer the ergonomic risks and burden of musculoskeletal injuries from the primary (console) surgeon to the bedside surgical assistant.
Quantifying the biomechanical and perceived workload of robotic surgery will provide a foundation for identifying intraoperative ergonomic risk factors and designing and evaluating solutions to improve surgical techniques and the associated patient outcomes. Toward this end, our objective is to (1) determine the feasibility of novel wearable sensors for continuously assessing biomechanical exposures and perceived workload during live surgery and (2) quantify and compare ergonomic strain of console surgeon and surgeon assistant roles during RARP.
Materials and methods
Participants
This study was approved by the institution’s ethics review board (dnr: 2014/1120-31) and urology surgeons performing RARP from a large academic hospital provided written consent to participate in this study. All RARP procedures were performed using the Karolinska technique, which is an established approach and has been previously described [28]. During this procedure, two surgeons perform parallel tasks at the robotic console or assist at the patient bedside. In this study, their role is classified according to the task they spent the majority of the case on.
Subjective and objective measures
Two questionnaires were used to measure self-reported musculoskeletal symptoms and perceived intraoperative workload. The first questionnaire was adapted from previous works [29, 30] and measured body part-specific musculoskeletal symptoms on mutually exclusive 3-point categorical scales, i.e., “No,” “Slight,” and “Substantial.” The second questionnaire included the validated surgery task load index (SURG-TLX), which measures six subdimensions of workload, i.e., mental demand, physical demand, temporal demand, task complexity, situational awareness, and distractions, using 20-point visual analogue scales (VAS) anchored by very low and very high [31]. In addition, surgeons self-reported the degree of difficulty of each procedure on a similar 20-point VAS adapted from previous work [32, 33]. Participants completed the musculoskeletal symptom questionnaire before and after each procedure. The SURG-TLX workload questionnaire was only completed postoperatively.
State-of-the-art wireless and wearable motion tracking devices were used to objectively quantify surgeon postures throughout the procedure. The OpalTM system (APDM, Inc., Portland, OR, USA) consists of six inertial measurement units (IMUs), each sensor containing accelerometer, gyroscope, and magnetometer. These sensors have previously been validated [34] and used for motion tracking in sports, space suits, and improving patient health [35–38]. Prior to the surgical procedure, IMUs were worn on the surgeon’s head, sternum, upper arms, and pelvis without interfering with surgeons’ performance and the sterile field (Fig. 2). The sixth sensor was used by a study team member to time stamp procedure start and stop times of procedures and roles. After donning the sensors and before starting each procedure, sensors were calibrated using a static I-pose as described by the software vendor (NexGen Ergonomics, Montreal, Quebec). The units logged data at 64 Hz onto onboard memory cards that were downloaded and processed after the full surgical day.
Data analysis
Body regions with no musculoskeletal symptom responses (i.e., no indication of “No,” “Slight,” or “Substantial”) were conservatively assumed to be “No” symptoms. “Slight” and “Substantial” musculoskeletal symptom responses were collapsed into a single category (i.e., “Yes” musculoskeletal symptoms) and compared with “No” musculoskeletal symptoms for each body region. Workload data were analyzed and summarized for each subdimension of workload, and total workload was calculated as both the overall sum and the weighted sum of the six SURG-TLX scales as described in previous works [31, 39].
Sensor data were collected throughout the entire procedure, and procedure time was defined as incision to skin closure for the assisting surgeon and console start to console stop for the surgeon at the robotic console. Accelerometer, gyroscope, and magnetometer data from the IMU sensors were processed into postural angles using scripts programmed in MATLAB® (R2015b, Mathworks Inc., Natick, MA, USA). Specifically, these low-pass-filtered (fourth-order Butterworth filter set at 32 Hz) data streams were used to calculate neck flexion, torso flexion, and left/right shoulder elevation over time throughout the entire procedure with reference to the static I-pose. Neck flexion was defined as the head motion relative to the torso in the sagittal plane, torso flexion was defined relative to vertical, and shoulder elevation was defined as the upper arm motion relative to vertical [40]. These continuous computed postures were summarized for each procedure into (1) mean posture angles, (2) range of motion, defined as the difference between 95th percentile and 5th percentile posture angles, (3) percent time in demanding postures, (4) percent time in static postures, and (5) number of posture changes per minute. Demanding postures were based on previous commonly used definitions, >10° neck flexion, >20° torso flexion, and >45° shoulder elevation [22, 41]. Time in static posture was defined based on previously published surgical ergonomics literature as duration the flexion (neck and torso) or elevation (shoulders) angular velocities are <1°/s normalized by procedure time [42, 43]. Posture changes were defined as movements >10° with angular velocity faster than 1°/s.
Statistical analyses were performed using SPSS (v22, IBM, Inc.). Equal variance, two-tailed t tests were used to compare the demographics between participants performing console and assisting tasks. Analysis of variance was performed on all response variables that did not violate the assumption of normality, to identify the impact of surgeon role (console vs. assist). Nonparametric Mann–Whitney U tests were performed to identify differences between surgeon roles when response variables violated normality. Additionally paired t tests were performed for cases where data both console and assisting surgeons are available, i.e., same case.
Results
Fifteen RARP cases were collected in this study. Eight cases were first case of the day, six cases were second case of the day, and one was third case of the day. Due to surgeon and equipment availability (three sets of sensors), only 11 of the cases captured both assisting and console roles, i.e., same cases. Cases were performed by 10 different participants, six primarily performed console tasks and four primarily performed assisting tasks (Table 1). Eight participants were males, and two were females. Nine participants were right-handed, and one was completely ambidextrous. Five surgeons reported extensive robotic surgery experience, and five reported having some robotic surgery experience. Operative duration was longer assisting than for those performing the console role (142 ± 52 vs. 129 ± 37 min, p = 0.38); this was due to the different definitions of procedure time for the assistant and console surgeons (see “Materials and methods” section).
Self-reported metrics
Musculoskeletal pain was present across all measured regions except for left finger and wrist (Table 2). Surgeons performing assisting tasks only reported experiencing left shoulder and neck pain. Postoperative pain was reported in 46 % of the cases in the right shoulder and neck among surgeons performing console tasks. Musculoskeletal pain and numbness were more frequently reported after performing console than assisting cases; however, half of all participants (two out of the four assisting and three out of six console surgeons) experienced postoperative musculoskeletal pain for at least one or more cases.
The SURG-TLX questionnaire mean rated workload scores were significantly higher (p = 0.004–0.04) for console compared to assisting tasks for mental demand, temporal demand, task complexity, and situational awareness (Fig. 3). Findings from paired analysis on the subset of matched cases (n = 11) were similar, and workload was statistically different between roles for temporal demand, task complexity, and situational awareness. Rated overall workload was 22 % higher (p = 0.01) during console tasks than assisting tasks. Overall workload exceeded 50 (out of 100) for 62 and 23 % of the cases for console and assisting surgeons, respectively. Comparison between overall workload and weighted overall workload, as defined Wilson et al. [31], showed that the measures were highly correlated (Adj. R 2 = 0.92, p < 0.001). Thus, discussion will focus on the overall workload metric.
Posture and movement metrics
To illustrate postural patterns, an example of neck, chest, and left/right shoulder posture angles for one participant during an entire case is shown in Fig. 4, which shows two distinct patterns. The first pattern was observed from the start of the procedure to the 65th-min mark, where (1) neck flexion was high and the amplitude of the movements was large, and (2) shoulder elevation was low and amplitude of movements was small. Second pattern was observed from the 65th-min mark to the end of procedure, where (1) neck flexion was near zero and movements were small in amplitude, and (2) shoulders were elevated and had larger amplitude of movements (Fig. 4). These distinct patterns represented the rotation of roles by this participant, where he first assisted in the procedure by the patient bedside and then finished the procedure at the console. At the participating university teaching hospital, this rotation between assisting and console tasks during the same procedure was frequently used and was observed for two cases.
A summary of the biomechanical posture measurements across all participants is shown in Tables 3 and 4. In comparison with surgeons at the console, assisting surgeons exhibited 9° more flexed neck posture (p < 0.05) and 14°–41° larger (p < 0.05) neck, torso, and right shoulder range of motion (Table 3). Time in demanding neck postures was over twice as high (p < 0.001) for assisting than console (Table 3). Measures of posture over time (Table 4) differed significantly between console and assisting roles for every metric. Specifically, assisting surgeons had 5.0 times more (p < 0.001) neck and torso movements and 1.6 times more (p < 0.01) shoulder movements than console (Table 4). In addition, console surgeons were observed to have more (p < 0.05) static neck, torso, and left shoulder postures than assisting surgeons. Right shoulder postures were more (p < 0.01) static for assisting than console surgeons (Table 4). These postures are shown in Fig. 5A which demonstrate elevated and unsupported right shoulder postures for the surgeon at the console (shoulders should be lower and ideally resting on elbow rests as shown in Fig. 1). Non-upright neck postures are shown for the assistant in Fig. 5B.
Discussion
Surgical ergonomics and musculoskeletal injuries are recognized and significant issues in both laparoscopic and robotic surgeries [17, 21, 27, 44]. Suboptimal robotic operating room techniques may be detrimental to both patient outcomes and the occupational health of the surgical team [17, 21, 27, 44]. Results of the present study provide support and quantitative intraoperative data that can be used to help address the identified issues which require intervention. Similar to studies in laparoscopic surgery reporting prevalence of musculoskeletal symptoms as high as 87 % among surveyed surgeons [17, 18], our study in robotic surgery found that 50 % of participating surgeons experienced postoperative pain for one or more cases over the observed procedures (Table 2). Although these numbers are lower than previously reported prevalence, key differences include (1) musculoskeletal symptoms were measured prospectively after each case instead of retrospective surveys performed by previous works [17, 18], and (2) surgeon population in this study performed robotic surgeries which may overall improve surgeon ergonomics [3, 6].
Contrary to the conclusions reached in previous publications [3, 6], we found that the ergonomic benefits of robotic surgery did not eliminate musculoskeletal pain among console surgeons. Half of the participants on the console experienced pain (three of six), and pain occurred postoperatively in one or more body regions for 62 % of the cases (8 out of 13 cases). These findings are similar to Plerhoples et al.’s [44] survey study indicating that over half of robotic surgeons believed robotic surgery led to musculoskeletal symptoms and called for studies that quantify ergonomic benefits and risk factors for robotic surgeries.
The novel motion tracking sensors and methodology used in the present study can identify potential causal factors for the musculoskeletal pain and high physical workload. Continuous tracking of postures facilitates (1) quantifying of posture angles outside the range of recommended safe posture angles [41], (2) as a proxy for the frequency of prolonged muscle exertions due to static postures, and (3) identify areas needing ergonomic interventions. For example, right shoulder pain during console tasks occurred at an alarming rate of 46 % of cases and motion tracking data found that shoulders were elevated away from neutral at 24°–26° and were static for 42–52 % of the procedure. Elevated shoulders (Fig. 5A) have been shown to lead to fatigue and injuries [45], especially if sustained/static for extended periods of time without sufficient rest or recovery time. Although observed postures are less extreme than other similarly high-risk occupations [46–48], it is important to note physical symptoms may be exacerbated by the high mental workload. Specific, perceived workload was high (Fig. 3) and exceeded 50 % for 62 % of the observed cases, a threshold that has been suggested to increase errors in clinical tasks [49, 50]. This suggests either operative posture or surgeon technique requires addressing or that current console designs are insufficient. The robotic console allows adjustment of four different parameters, namely raising or lowering the armrests and headrest, the headrest can also be tilted to alter the flexion of the neck, and the footswitch panel can be moved closer to the body of further away. Although the console can be repositioned to improve posture for individual surgeons, suboptimal positioning may also constrain the surgeon’s dexterity, and Craven et al. [27] observed that console surgeons were not using armrests for 37 % of the robotic procedures. These issues can be improved, but require the surgeons to be aware of them and to be given suitable feedback (e.g., from motion tracking), education on the significance of ergonomics, and appropriate training.
Although preliminary, comparison of postures between console surgeons who did not report musculoskeletal pain and those who did indicated that surgeons who do not report pain had:
-
Mean postures closer to neutral (neck: −2° vs. 6°, L. shoulder: 24° vs. 29°, and R. shoulder: 21° vs. 26°),
-
Spent less time in postures that increase ergonomic risk (neck: 10 vs. 26 %, L. shoulder: 6 vs. 7 %, and R. shoulder: 5 vs. 12 %), and
-
Spent less time in static postures (neck: 61 vs. 64 %, L. shoulder: 50 vs. 54 %, and R. shoulder: 43 vs. 44 %).
Although only based on six console surgeons, these preliminary data suggest that novel intraoperative motion tracking has potential to be translated into practice for providing relevant feedback on surgeon ergonomics and identifying surgeons who may be at risk of musculoskeletal fatigue and injuries. Additionally, these data also have potential to guide corrections to poor body posture and reduce the risks.
The increasing adoption of robotic techniques for prostatectomies [1] was hypothesized in this study to adversely impact ergonomics for the assisting surgeon who now must maneuver around the sometimes unpredictable and bulky arms of the robot (Fig. 1). Motion tracking findings showed that assisting surgeons spend more time in demanding neck and torso postures than console surgeons (Table 3); however, musculoskeletal pain was reported less frequently by assisting than console surgeons at 15 % of observed cases (Table 2). Although this may be partially due to the younger age of assisting surgeons, the time-based motion tracking highlights additional factors in work constraints that are reducing the assisting surgeon’s risk of musculoskeletal pain during RARP. Specifically, assisting surgeons were observed to have higher range of motion (Table 3), more frequent posture movements (Table 4), and generally spend less time in static postures (Table 4). In addition, workload was significantly less (Fig. 3) for assisting than console surgeons, e.g., overall workload was 22 % lower. These findings suggest that the ergonomic risk factors, static postures and perceived workload, have an important impact of the reported musculoskeletal fatigue and pain, more so than other factors (e.g., forceful exertion, duty cycle) in other industries. However, it is important to note assisting tasks were observed to require neck postures that increase injury risks (Table 3) for 58 % of the procedure which may be associated with the prevalence of reported neck pain among assisting surgeons. Non-upright neck postures can be observed in Fig. 5B, where both patient and monitor positioning constrain the assistant’s neck and upper-body positioning.
Wearable intraoperative motion tracking has potential for identifying ergonomic risks in the operating room (OR) and can be used to assess the impact of new interventions, workplace layout, and surgical techniques. However, several limitations in the current technology warrant further examination. Specifically, motion tracking data are (1) collected prospectively and require a trained researcher, and (2) do not differentiate whether the musculoskeletal efforts of static postures were demanding or not. However, the present study demonstrates that this technology is feasible and provides actionable data and feedback to surgeons, e.g., degree of static postures or arm elevation. Findings in this study focus on RARP; however, this technology and similar ergonomic concerns have wider implications for other surgical specialties performing complex robotic procedures. Finally, predictors for musculoskeletal fatigue and injuries are multifactorial and are influenced by individual, environmental, and psychosocial factors. Due to the limited sample size and the duration of the study, only trends between measured ergonomics and postoperative musculoskeletal symptoms are identified. However, strong evidence exists linking workplace ergonomics and work duration with musculoskeletal injuries [45, 51, 52] and this technology can be used to identify and address these risk factors.
In conclusion, robotic surgery currently lacks objective research data assessing the important ergonomic issues of musculoskeletal fatigue and injuries in the operating room. Current ergonomic recommendations require further analysis of the unique work exposures in robotic surgery including the static work constraints, awkward postures, and high workload. Novel intraoperative motion wearables can quantify ergonomic deficiencies in the operating room and provide relevant personalized feedback (Fig. 5). Future application of this technology includes workplace interventions and identifying surgeons who are at risk. This will enable corrections to suboptimal ergonomic posture that aims to reduce these prevalent occupational health risks.
References
Chang SL, Kibel AS, Brooks JD, Chung BI (2015) The impact of robotic surgery on the surgical management of prostate cancer in the USA. BJU Int 115(6):929–936. doi:10.1111/bju.12850
Carlsson S, Nilsson AE, Schumacher MC, Jonsson MN, Volz DS, Steineck G, Wiklund PN (2010) Surgery-related complications in 1253 Robot-assisted and 485 open retropubic radical prostatectomies at the Karolinska University Hospital, Sweden. Urology 75(5):1092–1097. doi:10.1016/j.urology.2009.09.075
Diana M, Marescaux J (2015) Robotic surgery. Br J Surg 102(2):e15–e28. doi:10.1002/bjs.9711
Alemozaffar M, Sanda M, Yecies D, Mucci LA, Stampfer MJ, Kenfield SA (2015) Benchmarks for operative outcomes of robotic and open radical prostatectomy: results from the health professionals follow-up study. Eur Urol 67(3):432–438. doi:10.1016/j.eururo.2014.01.039
Reza M, Maeso S, Blasco JA, Andradas E (2010) Meta-analysis of observational studies on the safety and effectiveness of robotic gynaecological surgery. Br J Surg 97(12):1772–1783. doi:10.1002/bjs.7269
Close A, Robertson C, Rushton S, Shirley M, Vale L, Ramsay C, Pickard R (2013) Comparative cost-effectiveness of robot-assisted and standard laparoscopic prostatectomy as alternatives to open radical prostatectomy for treatment of men with localised prostate cancer: a health technology assessment from the perspective of the UK national health service. Eur Urol 64(3):361–369. doi:10.1016/j.eururo.2013.02.040
Ficarra V, Novara G, Rosen RC, Artibani W, Carroll PR, Costello A, Menon M, Montorsi F, Patel VR, Stolzenburg J-U, Van der Poel H, Wilson TG, Zattoni F, Mottrie A (2012) Systematic review and meta-analysis of studies reporting urinary continence recovery after robot-assisted radical prostatectomy. Eur Urol 62(3):405–417. doi:10.1016/j.eururo.2012.05.045
Ficarra V, Novara G, Ahlering TE, Costello A, Eastham JA, Graefen M, Guazzoni G, Menon M, Mottrie A, Patel VR (2012) Systematic review and meta-analysis of studies reporting potency rates after robot-assisted radical prostatectomy. Eur Urol 62(3):418–430
Novara G, Ficarra V, Mocellin S, Ahlering TE, Carroll PR, Graefen M, Guazzoni G, Menon M, Patel VR, Shariat SF, Tewari AK, Van Poppel H, Zattoni F, Montorsi F, Mottrie A, Rosen RC, Wilson TG (2012) Systematic review and meta-analysis of studies reporting oncologic outcome after robot-assisted radical prostatectomy. Eur Urol 62(3):382–404. doi:10.1016/j.eururo.2012.05.047
Novara G, Ficarra V, Rosen RC, Artibani W, Costello A, Eastham JA, Graefen M, Guazzoni G, Shariat SF, Stolzenburg J-U (2012) Systematic review and meta-analysis of perioperative outcomes and complications after robot-assisted radical prostatectomy. Eur Urol 62(3):431–452
Boggi U, Signori S, De Lio N, Perrone VG, Vistoli F, Belluomini M, Cappelli C, Amorese G, Mosca F (2013) Feasibility of robotic pancreaticoduodenectomy. Br J Surg 100(7):917–925. doi:10.1002/bjs.9135
Gutt CN, Oniu T, Mehrabi A, Kashfi A, Schemmer P, Büchler MW (2004) Robot-assisted abdominal surgery. Br J Surg 91(11):1390–1397. doi:10.1002/bjs.4700
Butturini G, Damoli I, Crepaz L, Malleo G, Marchegiani G, Daskalaki D, Esposito A, Cingarlini S, Salvia R, Bassi C (2015) A prospective non-randomised single-center study comparing laparoscopic and robotic distal pancreatectomy. Surg Endosc 29(11):3163–3170. doi:10.1007/s00464-014-4043-3
Bagrodia A, Raman JD (2009) Ergonomic considerations of radical prostatectomy: physician perspective of open, laparoscopic, and robot-assisted techniques. J Endourol 23(4):627–633
Park JY, Jo MJ, Nam BH, Kim Y, Eom BW, Yoon HM, Ryu KW, Kim YW, Lee JH (2012) Surgical stress after robot-assisted distal gastrectomy and its economic implications. Br J Surg 99(11):1554–1561. doi:10.1002/bjs.8887
Lowndes BR, Hallbeck MS (2014) Overview of human factors and ergonomics in the OR, with an emphasis on minimally invasive surgeries. Hum Factors Ergon Manuf Serv Ind 24(3):308–317. doi:10.1002/hfm.20383
Park A, Lee G, Seagull FJ, Meenaghan N, Dexter D (2010) Patients benefit while surgeons suffer: an impending epidemic. J Am Coll Surg 210(3):306–313
Szeto GP, Ho P, Ting AC, Poon JT, Cheng SW, Tsang RC (2009) Work-related musculoskeletal symptoms in surgeons. J Occup Rehabil 19(2):175–184
Davis WT, Fletcher SA, Guillamondegui OD (2014) Musculoskeletal occupational injury among surgeons: effects for patients, providers, and institutions. J Surg Res 189(2):207–212. doi:10.1016/j.jss.2014.03.013
van Det MJ, Meijerink WJHJ, Hoff C, van Veelen MA, Pierie JPEN (2008) Ergonomic assessment of neck posture in the minimally invasive surgery suite during laparoscopic cholecystectomy. Surg Endosc 22(11):2421–2427. doi:10.1007/s00464-008-0042-6
Miller K, Benden M, Pickens A, Shipp E, Zheng Q (2012) Ergonomics principles associated with laparoscopic surgeon injury/illness. Hum Factors: J Hum Factors Ergon Soc 54(6):1087–1092
Yu D, Lowndes B, Morrow M, Kaufman K, Bingener J, Hallbeck S (2015) Impact of novel shift handle laparoscopic tool on wrist ergonomics and task performance. Surg Endosc. doi:10.1007/s00464-015-4634-7
Stefanidis D, Wang F, Korndorffer JR Jr, Dunne JB, Scott DJ (2010) Robotic assistance improves intracorporeal suturing performance and safety in the operating room while decreasing operator workload. Surg Endosc 24(2):377–382
Berguer R, Smith W (2006) An ergonomic comparison of robotic and laparoscopic technique: the influence of surgeon experience and task complexity. J Surg Res 134(1):87–92
Lee GI, Lee MR, Clanton T, Sutton E, Park AE, Marohn MR (2014) Comparative assessment of physical and cognitive ergonomics associated with robotic and traditional laparoscopic surgeries. Surg Endosc 28(2):456–465
Hubert N, Gilles M, Desbrosses K, Meyer JP, Felblinger J, Hubert J (2013) Ergonomic assessment of the surgeon’s physical workload during standard and robotic assisted laparoscopic procedures. Int J Med Robot Comput Assist Surg 9(2):142–147. doi:10.1002/rcs.1489
Craven R, Franasiak J, Mosaly P, Gehrig PA (2013) Ergonomic deficits in robotic gynecologic oncology surgery: a need for intervention. J Minim Invasive Gynecol 20(5):648–655
Nilsson AE, Carlsson S, Laven BA, Wiklund NP (2006) Karolinska prostatectomy: a robot-assisted laparoscopic radical prostatectomy technique. Scand J Urol Nephrol 40(6):453–458. doi:10.1080/00365590600911357
Trejo A, Doné K, DiMartino A, Oleynikov D, Hallbeck M (2006) Articulating vs. conventional laparoscopic grasping tools—surgeons’ opinions. Int J Ind Ergon 36(1):25–35
Beurskens AJ, Bültmann U, Kant I, Vercoulen JH, Bleijenberg G, Swaen GM (2000) Fatigue among working people: validity of a questionnaire measure. Occup Environ Med 57(5):353–357
Wilson MR, Poolton JM, Malhotra N, Ngo K, Bright E, Masters RS (2011) Development and validation of a surgical workload measure: the surgery task load index (SURG-TLX). World J Surg 35(9):1961–1969
Vassiliou MC, Feldman LS, Andrew CG, Bergman S, Leffondré K, Stanbridge D, Fried GM (2005) A global assessment tool for evaluation of intraoperative laparoscopic skills. Am J Surg 190(1):107–113
Abdelrahman A, Bingener J, Yu D, Lowndes B, Mohamed A, McConico A, Hallbeck MS (2015) Impact of single-incision laparoscopic cholecystectomy (SILC) versus conventional laparoscopic cholecystectomy (CLC) procedures on surgeon stress and workload: a randomized controlled trial. Surg Endosc. doi:10.1007/s00464-015-4332-5
Schall MC, Fethke NB, Chen H, Oyama S, Douphrate DI (2015) Accuracy and repeatability of an inertial measurement unit system for field-based occupational studies. Ergonomics. doi:10.1080/00140139.2015.1079335
Fantozzi S, Giovanardi A, Magalhães FA, Di Michele R, Cortesi M, Gatta G (2015) Assessment of three-dimensional joint kinematics of the upper limb during simulated swimming using wearable inertial-magnetic measurement units. J Sports Sci. doi:10.1080/02640414.2015.1088659
Anderson A, Hilbert A, Bertrand P, McFarland S, Newman D (2014) In-suit sensor systems for characterizing human-space suit interaction. In: 44th International conference on environmental systems
Cohen RG, Gurfinkel VS, Kwak E, Warden AC, Horak FB (2015) Lighten up specific postural instructions affect axial rigidity and step initiation in patients with Parkinson’s disease. Neurorehabil Neural Repair 29(9):878–888
Horak F, King L, Mancini M (2015) Role of body-worn movement monitor technology for balance and gait rehabilitation. Phys Ther 95(3):461–470
Hart SG, NASA-Task Load Index (NASA-TLX); 20 years later. In: Proceedings of the human factors and ergonomics society annual meeting, 2006. Sage Publications, pp 904–908
Morrow MM, Hurd WJ, Kaufman KR, An K-N (2010) Shoulder demands in manual wheelchair users across a spectrum of activities. J Electromyogr Kinesiol 20(1):61–67
McAtamney L, Corlett EN (1993) RULA: a survey method for the investigation of work-related upper limb disorders. Appl Ergon 24(2):91–99
Szeto GP, Cheng SW, Poon JT, Ting AC, Tsang RC, Ho P (2012) Surgeons’ static posture and movement repetitions in open and laparoscopic surgery. J Surg Res 172(1):e19–e31
Yu D, Green C, Kasten SJ, Sackllah ME, Armstrong TJ (2016) Effect of alternative video displays on postures, perceived effort, and performance during microsurgery skill tasks. Appl Ergon 53:281–289. doi:10.1016/j.apergo.2015.10.016
Plerhoples TA, Hernandez-Boussard T, Wren SM (2012) The aching surgeon: a survey of physical discomfort and symptoms following open, laparoscopic, and robotic surgery. J Robot Surg 6(1):65–72
Bernard BP, Putz-Anderson V (1997) Musculoskeletal disorders and workplace factors: a critical review of epidemiologic evidence for work-related musculoskeletal disorders of the neck, upper extremity, and low back. US Department of Health and Human Services (DHHS) publication no 97-141. National Institute for Occupational Safety and Health, Cincinnati
Åkesson I, Balogh I, Hansson GÅ (2012) Physical workload in neck, shoulders and wrists/hands in dental hygienists during a work-day. Appl Ergon 43(4):803–811. doi:10.1016/j.apergo.2011.12.001
Arvidsson I, Balogh I, Hansson G-Å, Ohlsson K, Åkesson I, Nordander C (2012) Rationalization in meat cutting—consequences on physical workload. Appl Ergon 43(6):1026–1032. doi:10.1016/j.apergo.2012.03.001
Byström JU, Hansson G-Å, Rylander L, Ohlsson K, Källrot G, Skerfving S (2002) Physical workload on neck and upper limb using two CAD applications. Appl Ergon 33(1):63–74. doi:10.1016/S0003-6870(01)00044-8
Mazur LM, Mosaly PR, Hoyle LM, Jones EL, Marks LB (2013) Subjective and objective quantification of physician’s workload and performance during radiation therapy planning tasks. Pract Radiat Oncol 3(4):e171–e177. doi:10.1016/j.prro.2013.01.001
Mosaly PR, Mazur LM, Jones EL, Hoyle L, Zagar T, Chera BS, Marks LB (2013) Quantifying the impact of cross coverage on physician’s workload and performance in radiation oncology. Pract Radiat Oncol 3(4):e179–e186. doi:10.1016/j.prro.2013.02.007
Descatha A, Dale AM, Silverstein BA, Roquelaure Y, Rempel D (2015) Lateral epicondylitis: new evidence for work relatedness. Joint Bone Spine 82(1):5–7
Fan ZJ, Harris-Adamson C, Gerr F, Eisen EA, Hegmann KT, Bao S, Silverstein B, Evanoff B, Dale AM, Thiese MS (2015) Associations between workplace factors and carpal tunnel syndrome: a multi-site cross sectional study. Am J Ind Med 58(5):509–518
Acknowledgments
The authors would like to acknowledge the support and participation of the surgeons involved in this study. This research made possible in part by the Mayo Clinic Robert D. and Patricia E. Kern Center for the Science of Health Care Delivery.
Author information
Authors and Affiliations
Corresponding author
Ethics declarations
Disclosures
Dr. Collins reports grants from Intuitive Surgical, outsider of the submitted work. Drs. Yu, Morrow, Dural, Hallbeck, Kjellman, Forsman, and Ms. Yang have no conflicts of interest or financial ties to disclose.
Additional information
Susan Hallbeck, Magnus Kjellman and Mikael Forsman have contributed equally to this work.
Rights and permissions
About this article
Cite this article
Yu, D., Dural, C., Morrow, M.M.B. et al. Intraoperative workload in robotic surgery assessed by wearable motion tracking sensors and questionnaires. Surg Endosc 31, 877–886 (2017). https://doi.org/10.1007/s00464-016-5047-y
Received:
Accepted:
Published:
Issue Date:
DOI: https://doi.org/10.1007/s00464-016-5047-y