Abstract
Submerged fermentation (SmF) and solid-state fermentation (SSF) of Aspergillus japonicus PJ01 for multi-enzyme complexes (MEC) production were comparatively studied. The results showed that orange peel and wheat bran were the best substrates for MEC production in SmF and SSF, respectively. After 72 h of cultivation under SmF, the maximal pectinase, CMCase, and xylanase activities reached 2610, 85, and 335 U/gds (units/gram dry substrate), respectively; while after 72 h of cultivation under SSF, these three enzymes’ activities reached 966, 58, and 1004 U/gds, respectively. Effects of ultrasound on extraction of crude enzymes from SSF medium were determined, the maximal activities of pectinase, CMCase, and xylanase increased to 1.20, 1.48, and 1.30-fold, respectively. Apparent different mycelia growths of SSF and SmF were observed by scanning electron microscopy; and different isoforms of the crude enzyme extracts from SSF and SmF were presented by zymogram analysis.
Similar content being viewed by others
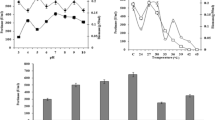
Explore related subjects
Discover the latest articles, news and stories from top researchers in related subjects.Avoid common mistakes on your manuscript.
Introduction
Cellulose, hemicellulose, and pectin are the main components of plant cell wall polysaccharides which widely exist in agricultural residues [1]. It is well known that fungi could produce cellulolytic multi-enzyme complexes (MEC) of pectinase, xylanase, and cellobiase to degrade these polysaccharides [2–4]. Cellulolytic MEC have attracted great attention in biofuel technology as they offer a solution to the effective degradation of complex plant materials into fermentable sugars [5]. Many fungi such as Scytalidium, Trichoderma, Aspergillus, and Penicillium have been proved effective for MEC production by agro residues fermentation [6–9].
MEC production is usually carried out in solid-state (SSF) and submerged fermentation (SmF). Compared to SmF, SSF presents unparalleled advantages, such as higher productivity per reactor volume, lower capital and operating costs, lower space requirements, simpler equipment and easier downstream processing [10]. However, there are still some obstacles needed to overcome, such as unsatisfactory reproducibility of the results, difficulty in scale-up, regulation and monitoring of biomass concentration, and complicated product purification [11]. Recently, SSF and SmF have been compared for production of single enzyme, e.g., endoglucanase [12], xylanase [13], and cellulase [14]. However, the studies on MEC production are still lacking [15]. In addition, although the morphological and physiological evolution of Aspergillus mycelium in submerged culture and its relation to the formation of secondary metabolites have already been investigated [16, 17], little is known on the characteristics of Aspergillus mycelium and spores in SSF.
Extraction of enzyme from the crude fermented products is an important aspect of SSF technology. Extraction solvent, time, agitation, solid/liquid ratio, and temperature have been normally taken into consideration for enzyme recovery from SSF substrates [18, 19]. Power ultrasound refers to sound waves with low frequencies (20–100 kHz) and high sound intensities (10 W/cm2–1 kW/cm2). Cavitation bubbles created by sonication collapse asymmetrically near the solid surface and produce high speed liquid jets, which provide a greater penetration of the solvent into solid materials and improve the mass transfer [20]. It has been established that low-frequency ultrasound had a positive effect on the activity of cellulase, though the selection of operating conditions played a crucial role in deciding the intensification [21, 22]. Recently, ultrasonic stimulation has been demonstrated to significantly improve not only fungal enzymes production in SmF [23, 24], but also the efficiency of enzymes extraction from the SSF materials [20, 25]. However, little was known about the effects of sonication on microstructure of SSF materials and zymograms of SSF enzymes.
In our previous work, a newly Aspergillus japonicus PJ01 strain was screened, isolated, and identified. The strain could efficiently degrade pectin, carboxymethylcellulose (CMC), and xylan by a Congo red overlay assay. The aims of this work are: (a) to screen the most suitable substrates for multi-enzyme complexes production by A. japonicus PJ01 in SmF and SSF; (b) to compare the multi-enzymes production, mycelia growths, and zymograms between SSF and SmF using the most suitable substrates; and (c) to investigate the effect of ultrasound irritation on fungal crude enzyme recovery from SSF material.
Materials and methods
Microorganism
Strain A. japonicus PJ01 (GenBank accession number KF550286) was isolated from a soil sample from Yuelu Mountain, Changsha, China. The identified A. japonicas strain was deposited at the China Center for Type Culture Collection (CCTCC), Wuhan, China, with an accession no. CCTCC M2013323. This strain’s colonies showed degradation capacities of pectin, CMC and xylan by Congo red overlay method [26]. The strain was grown on potato dextrose agar (PDA) slants at 35 °C for 4 days and subsequently stored at 4 °C. Inoculum was prepared by suspending the spores from PDA slants by adding sterile distilled water with 0.1 % Tween-80.
Raw materials
Typical agro-industrial residues such as orange peel (OP), wheat bran (WB), orange bagasse (OB), sugarcane bagasse (SB), and rice straw (RS) were obtained locally (Changsha, China). They were dried in an oven at 60 °C for 48 h, milled, and sieved to particles (20–40 mesh). Table 1 shows the main compositions of these residues.
Submerged fermentation (SmF)
WB, OP, OB, and substrates mixture (WB:OP = 1:1) were evaluated for their potentials for multi-enzyme complexes production in SmF. Single factor tests have been used for the following culture conditions optimization: 75 mL of a mineral salt media (NaNO3 5 g; K2HPO4 1 g; KCl 0.5 g; MgSO4·7H2O 0.5 g; FeSO4·7H2O 0.01 g in 1000 mL of distilled water) were supplemented with 1.5 % (w/v) of substrates in 250 mL Erlenmeyer flasks, spores concentration was approximately 5 × 105 spores/mL medium, and culture temperature was 35 °C under agitation at 170 rpm. The cultured media were filtered through coarse filter paper, filtrates were centrifuged at 10,000×g for 10 min at 4 °C, and the supernatants were used as crude enzyme solutions.
Solid-state fermentation (SSF)
WB, RS, OP, OB, SB, and substrate mixtures (WB:OP = 1:1, WB:RS = 1:1) were evaluated for their potentials for multi-enzyme complex production in SSF. Single factor tests have been used for the following culture conditions optimization: 100 mL flasks containing 1.5 g substrates, moistened with 2.25 mL mineral salt media (peptone 3 g; K2HPO4 1 g; KCl 0.5 g; MgSO4·7H2O 0.5 g; FeSO4·7H2O 0.01 g; in 1000 mL of water), spores concentration was approximately 1 × 107 spores/gram dry substrate (gds), and culture temperature was 30 °C. Enzymes extractions in 0.1 M acetate buffer pH 5 were carried out at a solid/liquid ratio of 1:20 for 45 min on a rotary shaker at 170 rpm. Extracts were filtered through coarse filter paper, filtrates were centrifuged at 10,000×g for 10 min at 4 °C, and the supernatants were used as crude enzyme solutions.
Sonication system
After extraction in acetate buffer for 45 min, the SSF material was exposed to ultrasonic treatment. The device was a probe sonicator (VOSHIN-650Y, Wuxi Voshin Instruments Co., LTD, Wuxi, China) with a driving frequency of 20 kHz and a power of 20–650 W supplied by a piezoelectric transducer and with a 6 mm diameter replaceable tip. The temperature was set to 50 ± 2 °C by jacket cooling. The ultrasonic stimulation was supplied using the “duty cycle” control, which consisted of 3 s working time and 3 s stop time. After 5, 10, 20, and 30 min of ultrasonic pretreatments, the SSF materials were filtered and centrifuged (see "Solid-state fermentation (SSF)"), respectively. The supernatants were used as crude enzyme solutions; the filtered residues were used for SEM analyses.
Analytical methods
Cellulose, lignin, hemicellulose, and pectin were assayed by the methods of Liu [27]. Briefly, the cellulose was determined by HNO3-ethanol method, 1.00 g of sample was placed in a 250 mL Erlenmeyer flask to which 25 mL of HNO3 and ethanol (1:4 v/v) was added, the solution was immersed in a boiling bath for 2 h until the fiber had faded to white, the cellulose was collected by filtration, dried at 105 ± 3 °C and reweighed. The lignin was determined by Klason method, 1.00 g of sample was placed in a 100 mL Erlenmeyer flask to which 15 mL of H2SO4 (72 % w/w) was added and shaken for 2 h, then content was diluted to 3 % (w/w) in a 1000 mL Erlenmeyer flask and hydrolyzed for 4 h with boiling water, the lignin was collected by filtration, dried at 105 ± 3 °C and reweighed. The hemicellulose was determined by two-brominating method, 1.00 g of the sample was added into a round bottom flask and distillated with HCl solution (12 % w/w) for 100 min, 300 mL of furfural distillate was obtained. 200 mL of distillate was transferred to a conical flask and 25 mL of NaBrO3 (1.2 % w/v)/NaBr (0.25 % w/v) solution was added and allowed to stand for 5 min. 10 mL of potassium iodide (10 % w/v) was then added and the liberated iodine titrated with a standard 0.1 M sodium thiosulphate solution. The pectin was determined by alcohol extraction method, 1.00 g of the sample was weighed into a round bottom flask and refluxed with 100 mL of 1.0 and 0.5 % (w/v) oxalate ammonium for 3 h successively. All extracts were recovered by filtration and precipitated with four volume of ethanol. The pectin was collected by filtration, dried at 105 ± 3 °C and reweighed. Protein content (N × 6.25) was determined by the combustion method with Kejeltec 2300 autoanalyzer (Foss Tecator AB, Hoganas, Sweden).
Pectinase, CMCase, and xylanase activities were determined according to the methods of Díaz et al. [28], Ghose [29], and Bailey et al. [30], respectively. One unit of pectinase, CMCase, and xylanase was defined as the amount of enzyme required to release 1 μmol of d-galacturonic acid, glucose, and xylose, respectively, per minute under standard assay conditions. Reducing sugar content of fermentation broth was determined by DNS method [31]. Soluble proteins content was determined by modified Bradford method using bovine serum albumin (BSA) as a standard [32].
Due to the difficulty in separating the mycelia from the medium, the biomass was measured by the inner nucleic acid (INA) method. Medium was filtered on a weighting filter, and the cake was washed with distilled water three times. Dry weight was measured after drying in an oven at 60 °C for 24 h. 0.02 g of dried biomass was incubated at 80 °C with 10 mL of trichloroacetic acid (5 % w/v) on a rotary shaker at 130 rpm for 25 min. After centrifuging (15 min at 10,000×g), the supernatant was diluted and assayed by UV–vis spectrophotometer (UV-670, AOE instruments, Shanghai, China) at wavelength of 260 nm. Concentration was calculated using appropriate standard curve of dry mycelium [33].
The enzyme activity was expressed as international activity unit (U) per gram dry substrate (gds); and the reducing sugar, soluble protein, and INA content were expressed as milligram per gram dry substrate.
Scanning electron microscopy (SEM)
To understand the hypha difference in morphology among SmF, SSF, and ultrasound treated SSF materials, samples were analyzed by SEM (JEOL, JSM-6360LV, Tokyo, Japan). The samples for SEM analysis were fixed in glutaraldehyde (10 % w/v) and dehydrated with a gradient of ethanol and hexamethyldisilazane (HMDS). The fixed cells were dried by a drying apparatus and kept in desiccator until use. Then it was placed on the stubs, mounted with silver tape, and sputter coated with gold using fine coat and examined at 20 kV.
SDS-PAGE and zymography assay
Crude enzymes from SmF, SSF, and ultrasound treated SSF materials were placed in treated dialysis bags and concentrated 20 times by PEG 8000. The concentrated proteins were centrifuged at 10,000×g for 10 min at 4 °C, and subjected to sodium dodecyl sulfate-polyacrylamide electrophoresis (SDS-PAGE) using the Bio-Rad electrophoresis system. The samples were loaded onto the acrylamide gel (12 % w/v) containing a final concentration (0.2 % w/v) of pectin, CMC, and birchwood xylan, respectively [34]. Concentrated proteins (10 μL) were loaded onto the gels. Protein molecular weight markers (Tiangen Biotech, Co., Ltd., Beijing, China) were also loaded for estimation of the molecular weights of the active protein bands. Zymography assay was determined according to Ncube et al. [35].
Statistical analysis
SPSS version 19 software was used for the statistical analyses. The results are shown as the mean ± 1 standard deviation (SD), derived from three replications. The significance between different means was tested for using one-way analysis of variance (ANOVA) and Duncan’s new multiple range test, with significance accepted at the p ≤ 0.05 level.
Results and discussion
Submerged fermentation
Table 2 shows pectinase, CMCase, and xylanase production using different agro-industrial residues in SmF. Maximum enzyme activities of pectinase (2610 U/gds), CMCase (85 U/gds), and xylanase (335 U/gds) were achieved using OP as sole carbon source after 72 h of fermentation. It is generally agreed that extracellular pectinase was induced by the pectin-containing materials in the medium [36]. In this work, the selected substrates OP, OB, and WB are all pectin-containing materials; however, pectin content of OP is more than that of OB and WB (Table 1), indicating that OP is a more suitable substrate for pectinase production. Beside pectinase, the higher activities of CMCase and xylanase were also obtained using OP as the sole substrate. Table 2 also shows that the mixed carbon source (OP:WB = 1:1) was not favorable to multi-enzyme complex (MEC) production, compared with the sole substrate OP. This result could be explained that WB was not a suitable substrate in SmF. In addition, the pectin content of WB is less than that of OP, which might play a negative effect on pectinase production in SmF. Thus, OP was selected as the most suitable sole carbon source in SmF.
Figure 1 shows the cultivation profile of A. japonicas under SmF using substrate OP. The peaks of pectinase, CMCase, and xylanase activities were observed on the 72, 144, and 96 h of cultivation, respectively (Fig. 1a). The soluble protein content increased from 4.5 to 8.5 mg/gds after 120 h of cultivation (Fig. 1b), which indicated the increase of extracellular enzymes. Reducing sugar content decreased from 145 to 25 mg/gds, which indicates the extensive utilization of the carbon and energy (C/E) sources was available for biomass and product synthesis [37]. Inner nucleic acid content reached maximum value 580 mg/gds at 24 h and declined afterwards. These data indicate that the fungi grew fast until 24 h, afterward fungal metabolism rate was slow and inner nucleic acid decreased.
Solid-state fermentation
Effects of carbon sources on MEC production
Table 3 shows pectinase, CMCase, and xylanase production using different agro-industrial residues in SSF. Maximum activities of pectinase (966 U/gds), CMCase (58 U/gds), and xylanase (1004 U/gds) were achieved using WB as the sole carbon source. Table 3 also shows that WB was more suitable for CMCase and xylanase production than the other substrates. WB has also been reported to induce high levels of CMCase and xylanase in Aspergillus sp. Delabona et al. [38] observed higher CMCase and xylanase activities using wheat bran as substrate for A. fumigatus. The presence of minerals and amino acids in WB may help inducing cellulase and xylanase production [39]. In this work, protein content of WB (15.0 % w/w) was much more than that of OP (6.3 % w/w) and OB (6.4 % w/w) (Table 1), indicating of more amino acids in WB. On the other hand, there was no significant difference in pectinase activities between WB and OP. It indicates the different chemical composition and biomass structure between OP and WB did not affect pectinase production. Different combination of the substrates WB and OP, WB and RS were also tested in this work. The combination of WB with OP and RS resulted in decrease of three enzymes activities compared with single WB (Table 3). The results are in accordance with the previous study [40]. It might be explained that WB provided ample amounts of nutrients in the medium. Such nutrients could be necessary for the growth of the microorganisms and subsequently for cellulase production. Since WB was superior to other substrates, it was selected as the most suitable carbon source in SSF.
Solid-state fermentation by wheat bran
The solid-state cultivation profile of A. japonicus using wheat bran as sole carbon source is shown in Fig. 2. The peaks of pectinase, CMCase, and xylanase activities were observed after 72 h of cultivation (Fig. 2a). Soni et al. [41] reported activities of CMCase (20 IU/g) and xylanase (1722 IU/g) were obtained after 120 h of cultivation of the A. fumigatus fresenius strain on WB. Dhillon et al. [40] achieved higher CMCase (48 IU/g) and xylanase (2604 IU/g) production after 96 h of incubation using the A. niger strain cultivated on wheat bran. Compared with the previous works, the present work represents a substantial improvement in enzyme production, especially in terms of pectinase productivity, and higher enzyme activities were achieved in shorter period of time.
The soluble proteins content decreased from 9.0 to 4.2 mg/gds after 24 h of cultivation, and increased to 9.0 mg/gds after 96 h of cultivation (Fig. 2b). Heerd et al. [37] observed that the total proteins content decreased at the beginning of the fermentation, and increased in the following cultivation, which meant that more extracellular enzymes were secreted. Unlike SmF, reducing sugar content increased to 102 mg/gds after 24 h of cultivation, and decreased to 18 mg/gds after 96 h of cultivation (Fig. 2b). These data indicate soluble sugars from wheat bran degradation were produced abundantly in the initial stage of growth, and reduced in the next growth phase because of the massive growth of microorganisms. Inner nucleic acid concentration reached maximum value (160 mg/gds) after 96 h of cultivation and declined afterwards, which reflected the biomass changes during fermentation.
Effects of ultrasound on the crude enzymes recovery in SSF
To investigate the effects of low frequency ultrasound on enzymes recovery, SSF materials were exposed to ultrasonic powers (20–200 W) for different times (5–30 min). As shown in Fig. 3, under lower ultrasonic powers from 20 to 100 W, extraction of pectinase, CMCase, and xylanase increased until 20 min, and afterwards enzymes recovery decreased slowly. The results are in accordance with previous reports that low frequency ultrasound had a positive effect on the extraction of enzymes from SSF materials [20, 25]. Figure 3 shows that a prolonged time led to harmful effects on the activities of these enzymes. By increasing the ultrasonic power to 130 and 200 W, and increasing treatment time to 30 min, the losses in CMCase and xylanase activities became more significant: the former decreased to 48 U/gds (95 % of the original) and 33 U/gds (65 % of the original), respectively; the latter decreased to 686 U/gds (69 % of the original) and 473 U/gds (47 % of the original), respectively. These statements are in accordance with the results published in previous study [22], where the FPA activity decreased to 80 and 75 % of the original when the amplitude increased to 60 and 80 %, respectively. Szabó et al. [22] believed that sonication has a “sonochemical” effect on the enzyme molecules, which was manifested unambiguously in the diminished enzyme activity. Subhedar et al. [21] believed that continuous exposure to cavitating conditions for prolonged time led to degradation of the amino acid residues, which contributed to the substrate binding domain or catalytic domain of the enzyme molecules resulting in decrease in enzyme stability.
The highest activities of pectinase (1200 U/gds), CMCase (74 U/gds), and xylanase (1300 U/gds) were obtained under 33 W of ultrasonic power with treatment time 20 min (Fig. 3). Compared with control, these three enzyme activities exhibited 1.20, 1.48, and 1.30-fold enhancements, respectively. In addition, the soluble proteins concentration increased until 30 min under all the ultrasonic powers (Fig. 3d), the increased soluble proteins might come from absorbed enzymes and intracellular proteins. Effect of ultrasound irradiation on the morphology of hyphae cultured in SSF was also detected (Fig. 4c). Under 33 W of ultrasonic power with treatment time 20 min, most of hyphae were broken into pieces. Cell disruption by ultrasound irradiation could enhance the release of enzymes and proteins, which has been proved in previous literatures [42, 43].
Ultrasound-assisted extraction is widely used in food industry. The main positive effects can be explained by the intensified mass transfer, the reduction of particle size, the disruption of the cell wall, and as a consequence, the increased accessibility of the cell content by the solvent [25]. Further attempts are needed to shed more insight into ultrasonic power stimulation on multi-enzyme extraction of SSF.
SEM of A. japonicus morphology
Figure 4 shows SEM of A. japonicus morphology in SmF and SSF for 72 h (OP and WB was used as the mono substrate for SmF and SSF, respectively). The mycelia diameter in SSF was nearly 10 μm, and the mycelia diameter in SmF was only 3 μm. Compared with SmF, more spores were produced in SSF in this work. These data indicate that SSF was in favor of the growth and reproduction of A. japonicus, which might affect extracellular enzymes production. It indicates that SSF processes simulated the living conditions of many filamentous fungi, for which the enzymes, spores or metabolites were well adjusted to growth on solid wet substrates [11]. Therefore, SSF has been used successfully for the production of enzymes and secondary metabolites.
The SEM analyses showed significant differences in images of cells between SSF and SmF; however, a simple correlation of morphology and enzyme productivity could not be derived. To unfold the relationship between morphology and productivity, process parameters, mass transport, nutrient transport, and metabolic flux analysis have to be evaluated further.
SDS-PAGEs and zymograms
SDS-PAGEs of enzyme extracts from SmF, SSF, and ultrasound treated SSF material are shown in Fig. 5. It shows a notable difference in protein bands between SmF and SSF, but the bands of SSF are similar with that of ultrasound-treated SSF materials (Fig. 5, Lanes 1–3). The data indicate that ultrasound treatment did not increase the variety of proteins or enzymes. The zymographic study indicates that pectinase, CMCase, and xylanase produced by A. japonicus PJ01 were likely to be consisted of different isoforms (Fig. 5, Lanes 4–6). The pectinase zymograms for SmF and SSF were similar, i.e., one wide band about 85 kDa and one finer band about 45 kDa (Fig. 5a). The CMCase zymograms for SmF and SSF were different, the former showed three bands from 36 to 73 kDa, and the latter showed two bands from 27 to 30 kDa (Fig. 5b). CMCase isoforms from SSF were similar with previous work, where cellulase isoforms ranged from 20 to 43 kDa when A. niger was grown on Jatropha curcas seed cake [35]. The xylanase zymograms from SmF and SSF were also different, the former showed two weak bands from 24 to 34 kDa, and the latter showed four bright bands from 20 to 34 kDa (Fig. 5c). It clearly indicates that the expression of xylanase isoforms and levels were dependent on substrate types as well as culture conditions [44].
SDS-PAGEs and zymograms of extracellular pectinase (a), CMCase (b), and xylanase (c) produced by A. japonicas, where lane M molecular weight markers; lanes 1–3 SDS-PAGEs of enzyme extracts from SmF, SSF, ultrasonic treated SSF material; lanes 4–6 zymograms of enzyme extracts from SmF, SSF, ultrasonic treated material from SSF
Conclusions
The typical agricultural residues including orange peel, wheat bran, citrus bagasse, sugarcane bagasse, and rice straw were comparatively studied for production of multi-enzyme complexes (pectinase, CMCase, and xylanase) by A. japonicus PJ01 in SmF and SSF. The results indicated orange peel was the best substrate in SmF, and wheat bran was the best substrate in SSF. Low frequency ultrasound could improve the leaching efficiency of crude enzymes from SSF materials. Zymograms analyses demonstrated the multi-enzyme complexes consisted of different isozymes between SmF and SSF. Further study on scale-up operations of SSF or SmF for multi-enzyme complexes production could be helpful in faster commercialization of the process.
References
Brink J, Vries R (2011) Fungal enzyme sets for plant polysaccharide degradation. Appl Microbiol Biotechnol 91:1477–1492
Mamma D, Kourtoglou E, Christakopoulos P (2008) Fungal multienzyme production on industrial by-products of the citrus-processing industry. Bioresour Technol 99:2373–2383
Rodríguez-Fernández DE, Rodríguez-León JA, de Carvalho JC, Sturm W, Soccol CR (2011) The behavior of kinetic parameters in production of pectinase and xylanase by solid-state fermentation. Bioresour Technol 102:10657–10662
Delabona PdS, Pirota RDPB, Codima CA, Tremacoldi CR, Rodrigues A, Farinas CS (2012) Using Amazon forest fungi and agricultural residues as a strategy to produce cellulolytic enzymes. Biomass Bioenerg 37:243–250
van Dyk JS, Sakka M, Sakka K, Pletschke BI (2010) Characterisation of the multi-enzyme complex xylanase activity from Bacillus licheniformis SVD1. Enzyme Microb Technol 47:174–177
Jatinder K, Chadha B, Saini H (2006) Optimization of culture conditions for production of cellulases and xylanases by Scytalidium thermophilum using response surface methodology. World J Microbiol Biotechnol 22:169–176
Sukumaran RK, Singhania RR, Mathew GM, Pandey A (2009) Cellulase production using biomass feed stock and its application in lignocellulose saccharification for bio-ethanol production. Renew Energy 34:421–424
Rezazadeh Bari M, Alizadeh M, Farbeh F (2010) Optimizing endopectinase production from date pomace by Aspergillus niger PC5 using response surface methodology. Food Bioprod Process 88:67–72
Gonçalves D, Teixeira JA, Bazzolli DS, de Queiroz M, de Araújo E (2012) Use of response surface methodology to optimize production of pectinases by recombinant Penicillium griseoroseum T20. Biocatal Agric Biotechnol 1:140–146
Pandey A, Soccol CR, Nigam P, Soccol VT (2000) Biotechnological potential of agro-industrial residues. I: sugarcane bagasse. Bioresour Technol 74:69–80
Hölker U, Lenz J (2005) Solid-state fermentation—are there any biotechnological advantages? Curr Opi Microbiol 8:301–306
Saqib AAN, Hassan M, Khan NF, Baig S (2010) Thermostability of crude endoglucanase from Aspergillus fumigatus grown under solid state fermentation (SSF) and submerged fermentation (SmF). Process Biochem 45:641–646
Kar S, Gauri SS, Das A, Jana A, Maity C, Mandal A, Mohapatra PKD, Pati BR, Mondal KC (2013) Process optimization of xylanase production using cheap solid substrate by Trichoderma reesei SAF3 and study on the alteration of behavioral properties of enzyme obtained from SSF and SmF. Bioprocess Biosyst Eng 36:57–68
Gamarra NN, Villena GK, Gutiérrez-Correa M (2010) Cellulase production by Aspergillus niger in biofilm, solid-state, and submerged fermentations. Appl Microbiol Biotechnol 87:545–551
Kumar S, Sharma H, Sarkar B (2011) Effect of substrate and fermentation conditions on pectinase and cellulase production by Aspergillus niger NCIM 548 in submerged (SmF) and solid state fermentation (SSF). Food Sci Biotechnol 20:1289–1298
Bizukojc M, Ledakowicz S (2010) The morphological and physiological evolution of Aspergillus terreus mycelium in the submerged culture and its relation to the formation of secondary metabolites. World J Microbiol Biotechnol 26:41–54
Wucherpfennig T, Hestler T, Krull R (2011) Morphology engineering-Osmolality and its effect on Aspergillus niger morphology and productivity. Microb Cell Fact 10:58
Pal A, Khanum F (2010) Production and extraction optimization of xylanase from Aspergillus niger DFR-5 through solid-state-fermentation. Bioresour Technol 101:7563–7569
Chen H-Q, Chen X-M, Chen T-X, Xu X-M, Jin Z-Y (2011) Extraction optimization of inulinase obtained by solid state fermentation of Aspergillus ficuum JNSP5-06. Carbohydr Polym 85:446–451
Szabo OE, Csiszar E, Koczka B, Kiss K (2015) Ultrasonically assisted single stage and multiple extraction of enzymes produced by Aspergillus oryzae on a lignocellulosic substrate with solid-state fermentation. Biomass Bioenerg 75:161–169
Subhedar PB, Gogate PR (2014) Enhancing the activity of cellulase enzyme using ultrasonic irradiations. J Mol Catal B Enzym 101:108–114
Szabó OE, Csiszár E (2013) The effect of low-frequency ultrasound on the activity and efficiency of a commercial cellulase enzyme. Carbohydr Polym 98:1483–1489
Wang F, Ma A-Z, Guo C, Zhuang G-Q, Liu C-Z (2013) Ultrasound-intensified laccase production from Trametes versicolor. Ultrason Sonochem 20:118–124
Avhad DN, Rathod VK (2014) Ultrasound stimulated production of a fibrinolytic enzyme. Ultrason Sonochem 21:182–188
Szabo OE, Csiszar E, Toth K, Szakacs G, Koczka B (2015) Ultrasound-assisted extraction and characterization of hydrolytic and oxidative enzymes produced by solid state fermentation. Ultrason Sonochem 22:249–256
Teather RM, Wood PJ (1982) Use of Congo red-polysaccharide interactions in enumeration and characterization of cellulolytic bacteria from the bovine rumen. Appl Environ Microbiol 43:777–780
Liu S (2004) Analysis and measurement in papermaking industry. China Chemical Industry Press, Beijing
Díaz AB, Bolívar J, de Ory I, Caro I, Blandino A (2011) Applicability of enzymatic extracts obtained by solid state fermentation on grape pomace and orange peels mixtures in must clarification. LWT Food Sci Technol 44:840–846
Ghose T (1987) Measurement of cellulase activities. Pure Appl Chem 59:257–268
Bailey MJ, Biely P, Poutanen K (1992) Interlaboratory testing of methods for assay of xylanase activity. J Biotechnol 23:257–270
Miller GL (1959) Use of dinitrosalicylic acid reagent for determination of reducing sugar. Anal Chem 31:426–428
Bradford MM (1976) A rapid and sensitive method for the quantitation of microgram quantities of protein utilizing the principle of protein-dye binding. Anal Biochem 72:248–254
Liu G, Xu Z, Cen P (2000) A morphologically structured model for mycelial growth and secondary metabolite formation. Chin J Chem Eng 8:46–51
Lo Y-C, Huang C-Y, Cheng C-L, Lin C-Y, Chang J-S (2011) Characterization of cellulolytic enzymes and bioH2 production from anaerobic thermophilic Clostridium sp. TCW1. Bioresour Technol 102:8384–8392
Ncube T, Howard RL, Abotsi EK, van Rensburg ELJ, Ncube I (2012) Jatropha curcas seed cake as substrate for production of xylanase and cellulase by Aspergillus niger FGSCA733 in solid-state fermentation. Ind Crops Prod 37:118–123
Naidu G, Panda T (1998) Production of pectolytic enzymes-a review. Bioprocess Biosyst Eng 19:355–361
Heerd D, Yegin S, Tari C, Fernandez-Lahore M (2012) Pectinase enzyme-complex production by Aspergillus spp. in solid-state fermentation: a comparative study. Food Bioprod Process 90:102–110
Delabona PdS, Pirota RDPB, Codima CA, Tremacoldi CR, Rodrigues A, Farinas CS (2013) Effect of initial moisture content on two Amazon rainforest Aspergillus strains cultivated on agro-industrial residues: biomass-degrading enzymes production and characterization. Ind Crops Prod 42:236–242
Brijwani K, Oberoi HS, Vadlani PV (2010) Production of a cellulolytic enzyme system in mixed-culture solid-state fermentation of soybean hulls supplemented with wheat bran. Process Biochem 45:120–128
Dhillon GS, Oberoi HS, Kaur S, Bansal S, Brar SK (2011) Value-addition of agricultural wastes for augmented cellulase and xylanase production through solid-state tray fermentation employing mixed-culture of fungi. Ind Crops Prod 34:1160–1167
Soni R, Nazir A, Chadha BS (2010) Optimization of cellulase production by a versatile Aspergillus fumigatus fresenius strain (AMA) capable of efficient deinking and enzymatic hydrolysis of Solka floc and bagasse. Ind Crops Prod 31:277–283
Vargas LHM, Pião ACS, Domingos RN, Carmona EC (2004) Ultrasound effects on invertase from Aspergillus niger. World J Microbiol Biotechnol 20:137–142
Liu D, Zeng X-A, Sun D-W, Han Z (2013) Disruption and protein release by ultrasonication of yeast cells. Innov Food Sci Emerg Technol 18:132–137
Badhan AK, Chadha BS, Sonia KG, Saini HS, Bhat MK (2004) Functionally diverse multiple xylanases of thermophilic fungus Myceliophthora sp. IMI 387099. Enzyme Microb Technol 35:460–466
Acknowledgments
This work was supported by a Grant from the National High Technology Research and Development Program of China (863 Program) (No. 2011AA100804), Hunan Provincial Innovation Foundation for Postgraduate (CX2013B084), and Open-End Fund for the Valuable and Precision Instruments of Central South University (CSUZC2014003).
Author information
Authors and Affiliations
Corresponding author
Rights and permissions
About this article
Cite this article
Li, Pj., Xia, Jl., Shan, Y. et al. Comparative study of multi-enzyme production from typical agro-industrial residues and ultrasound-assisted extraction of crude enzyme in fermentation with Aspergillus japonicus PJ01. Bioprocess Biosyst Eng 38, 2013–2022 (2015). https://doi.org/10.1007/s00449-015-1442-3
Received:
Accepted:
Published:
Issue Date:
DOI: https://doi.org/10.1007/s00449-015-1442-3