Abstract
The activation of Trichinella spiralis muscle larvae (ML) by exposure to intestinal contents or bile and the intestinal epithelial cells (IECs) themselves are two pivotal requirements for the in vitro larval invasion of IECs. However, it is yet unknown which genes are involved in the process of larval invasion. The purpose of the present study was to analyze the differentially expressed genes of T. spiralis larvae activated by bile and cultured with IECs by using real-time polymerase chain reaction. Ten T. spiralis genes encoded the proteins produced by the larvae after co-culture with IECs were selected. Compared with untreated ML, four genes were up-regulated in both bile-activated and cell-cultured larvae, including calcium-dependent secretion activator (Csa; 2.55- and 16.04-fold, respectively), multi cystatin-like domain protein precursor (Mcd; 4.36 and 52), serine protease (Sp; 2.03 and 20.02), and intermediate filament protein ifa-1 (Ifa 1; 2 and 3.31). The expression of two genes, enolase (Eno; 1.51) and ribosomal protein S6 kinase beta-1 (Rsk; 1.49), was up-regulated only in cell-cultured larvae, not in bile-activated larvae. The expression of secreted 5′-nucleotidase (5 N; 1.42) and putative serine protease (Psp; 1.41) was up-regulated in bile-activated larvae, but was not changed or down-regulated after cultured with IECs. ATP synthase F1, beta subunit (ATPase; 0.58 and 0.51) and serine protease precursor (Spp; 0.42 and 0.65) were down-regulated in both bile-activated and cell-cultured larvae. This study provide some differentially expressed genes among the untreated (normal), bile-activated and cell-cultured larvae of T. spiralis. The up-regulated genes might be related with the larval invasion of IECs, but their exact biological functions need to be further investigated. This study will be helpful to further elucidate the molecular mechanism of the invasion of IECs by T. spiralis larvae and to better understand the interaction between parasite and host enterocytes.
Similar content being viewed by others
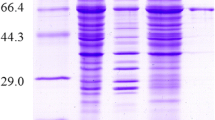
Avoid common mistakes on your manuscript.
Introduction
Trichinella spiralis is a tissue-dwelling parasitic nematode that infects humans and other mammals. Infection occurs by the consumption of animal meat containing the Trichinella larvae. Once ingested, muscle larvae (ML) are released from their capsules in the stomach by the digestive enzymes, and activated into the intestine infective larvae by exposure to intestinal contents or bile. Then, the activated larvae penetrate into host's intestinal epithelium where they molt and develop to adults that mate and reproduce the next generation of larvae. The life cycle of T. spiralis is completed when the newborn larvae invade and mature in the skeletal muscles of the host (Campbell 1983; Kang et al. 2012). It is well known that the invasion of host intestinal epithelial cells (IECs) by the infective larvae is the first step during T. spiralis infection. The larvae do not possess oral appendices or a spike (Bruce 1970), implying that the larval invasion of IECs may be not simply a result of mechanical penetration but possibly mediated by secretory–excretory (ES) proteins produced by the infective larvae (Man Warren et al. 1997; Ren et al. 2013a). Although the IEC model for the in vitro invasion of T. spiralis infective larvae has been established (Gagliardo et al. 2002; Ren et al. 2011), the mechanisms by which the larvae recognize and invade the host's IECs at the molecular levels has not been understood yet.
Previous studies showed that two pivotal requirements for the in vitro larval invasion of IECs are the activation of the larvae by exposure to intestinal contents or bile and the IECs themselves (Man Warren et al. 1997; Wang et al. 2010). The exposure can alter the behavior of the larvae, changing the type of movement exhibited from coiling to serpentine. Serpentine movement facilitate for the worm to gain entry into the cell monolayer. The bile-activated larvae invaded the IECs in vitro more aggressively than the unactivated ML larvae, and bile has been proven to be a cue that triggers the penetration into the IECs by the infective larvae. Therefore, bile is responsible for providing the necessary host–parasite communication required for successful invasion (Li et al. 1998; Theodoropoulos et al. 2005; Theodoropoulos and Petrakos 2010). Moreover, after the ML were activated by bile, the activated-larvae produce or excrete a few proteins which might be related with the invasion (Wang et al. 2013b). When the T. spiralis infective larvae were inoculated onto monolayers of IECs, they invade the IECs and produced several proteins, and some of these proteins entered the IECs (Wang et al. 2011, 2012; Wang et al. 2013a). However, it has not been clarified which larval proteins can interact with host enterocytes and play critical roles during recognition and invasion by T. spiralis. Additionally, the T. spiralis genes related to invasion of IECs have not yet been identified.
Real-time polymerase chain reaction (PCR) is a powerful tool to quantify gene expression. It is highly sensitive and allowed quantification of rare transcripts and small changes in gene expression (Cuttell et al. 2012). The purpose of this study was to analyze the differentially expressed genes of T. spiralis larvae activated by bile and cultured with IECs by using real-time PCR. Ten genes that encoded the novel proteins produced by the infective larvae after co-culture with IECs and entered the IECs were selected (Wang et al. 2012). This study will be helpful to further determine these protein functions, elucidate the molecular mechanism of the invasion of IECs by T. spiralis larvae and better understand the interaction between parasite and host enterocytes.
Material and methods
Parasites and experiment animals
The isolates (ISS534) of T. spiralis used in this study were obtained from a domestic pig in Nanyang City of Henan Province, China. The isolate was maintained by serial passages in Kunming mice in our laboratory. Six-week-old male Kunming mice were obtained from the Experimental Animal Center of Henan Province (Zhengzhou, China). The mice were maintained under specific pathogen-free conditions with sterilized food and water.
Cell culture
Human colonic epithelial cell line HCT-8 was obtained from the Cell Resource Center of Biolobical Sciences of the Chinese Academy of Science. HCT-8 cells were cultured in complete RPMI-1640 containing l-glutamine, nonessential amino acids and 10 % fetal bovine serum (FBS) at 37°C in 5 % CO2. The cell monolayer was dispersed by trypsinization (0.25 % trypsin, 0.02 % ethylenediaminetetraacetic acid [EDTA]) and passaged no more than 15 times before being used in experiments (Wang et al. 2011, 2012).
Treatment of ML
T. spiralis ML was recovered from infected mice at 42 days post infection by artificial digestion of carcasses with 1 % pepsin (1:3,000) and 1 % hydrochloric acid as described previously (Gamble et al. 2000; Yang et al. 2010). The recovered ML was enumerated under microscope and used in this study (Li et al. 2010). In order to imitate the normal intestinal milieu, ML were activated by the mouse bile (diluted 1:20 in saline) at 37 °C in 5 % CO2 for 2 h (Stewart et al. 1987). The bile was removed prior to analysis by exhaustively washing the worms in PBS supplemented with 100 U/ml penicillin and 100 μg/ml streptomycin, and the larvae were incubated in PBS at 37 °C in 5 % CO2 for additional 1 h (Gagliardo et al. 2002). Then, the bile-activated larvae at concentrations of 5,000/ml were inoculated onto the HCT-8 cells, and incubated at 37 °C in 5 % CO2 for 18 h (Kapel and Gamble 2000). The partial invasion of HCT-8 cells by the infective larvae was observed at 1 h after incubation. All larvae in the media were collected and counted at 18 h after incubation. The untreated (25,000 ± 100), bile-activated (25,033 ± 58) and cell-cultured (25,000 ± 100) larvae were respectively used for each incubation. The larval viability was determined by the activity and morphology. The live larvae are active in wriggling motion and exhibited serpentine movement, and the dead larvae are not active and had a fishhook or "C" shape (Jiang et al. 2012). After several washes in sterile RNase-free water, three groups of T. spiralis ML (untreated, bile-activated and cell-cultured larvae) were centrifuged at 600×g for 10 min and stored at −80 °C until use.
Total RNA isolation and reverse transcription (RT)
Total RNA was extracted from the samples of untreated, bile-activated and cell-cultured larvae using a TRIZOL RNA extraction kit (Takara, Japan) according to manufacturer's instructions. The extracted RNA was dissolved in DEPC-treated water, the concentration of RNA was 0.2–0.5 μg/μl and then stored in aliquots at −80 °C. The isolated RNA purity was determined spectrophotometrically (1.8–2.0 in ratio of OD260/OD280) and by formaldehyde agarose gel eletrophoresis (more than 1.6 in ratio of 28SrRNA/18SrRNA). A portion (1 μg) of RNA from each sample was used for cDNA synthesis. RT was performed using a PrimeScript® RT reagent Kit With gDNA Eraser (Takara) according to the manufacturer's instructions. Firstly, to eliminate genomic DNA contamination, the RNA extracts were treated with gDNA eraser and the mixture was incubated at 42 °C for 2 min. Secondly, The RNA was reverse-transcribed to cDNA using Oligo dT Primer and Random 6-mers. The RT reaction was carried out at 37 °C for 15 min and then 85 °C for 5 s. The cDNA product in a final volume of 20 μl was stored at −20 °C until use.
Primer design
The primers of ten genes were designed based on the sequences from GenBank database. Glyceraldehyde-3-phosphate dehydrogenase (G3PDH) which was confirmed to be expressed constitutively in different developmental stages of T. spiralis or in the larvae treated by different method was selected as internal control (Nagano et al. 2011; Chen et al. 2012; Ren et al. 2013b). The sequences of real-time PCR primers of the target genes and G3PDH and the lengths of the PCR amplicons are shown in Table 1.
Reverser transcription-polymerase chain reaction
The PCR reaction mixture contained 5 μl of the above cDNA, 2.5 μl of PCR buffer (10×), 1.5 μl of Mg2+ (2.5 mM), 2 μl of dNTP (2.5 mM), 1 μl of each primer (10 μmol/l), 0.4 μl of Ex Taq DNA polymerases (5 U/μl; Takara, Japan) and 11.6 μl of sterile water to produce the final volume of 25 μl. PCR reaction was conducted under the following conditions: an initial denaturation at 95 °C for 5 min, 35 cycles of denaturation at 95 °C for 30 s, annealing at 54 °C for 30 s and elongation at 72 °C for 1 min, and a final extension at 72 °C for 3 min. The PCR products were analyzed by electrophoresis on 1.5 % agarose gel.
Real-time PCR assay
The real-time PCR assay was performed on 7500 Fast real-time PCR system (Applied Biosystems, USA). First, an optimal condition of real-time RT-PCR for the ten genes and G3PDH was established using the SYBR Premix Ex Taq (Takara) according to the manufacturer's instructions. Twenty microliters of the reaction solution consisted of 2 μl of the template (1:20 dilution of cDNA product), 10 μl SYBR Premix Ex Taq (2×), 0.4 μl of 10 μM of each primer and 0.4 μl of ROX Reference Dye II. PCR amplification was performed as follows: predenature for 1 cycle at 95 °C for 30 s, and 40 cycles at 95 °C for 3 s, 60 °C for 30 s. The fold change in the target genes, normalized to G3PDH and relative to the expression in the larvae, was calculated using comparative Ct (2−ΔΔCt) method (Schmittgen and Livak 2008). Three independent experiments for three groups of larvae were performed and three-well repeats were measured for each sample.
Statistical analyses
Data were expressed as the mean ± standard deviation. Intra- and intergroup statistical analyses were performed with one-way ANOVA (LSD test) using SPSS version 17.0 software (SPSS Inc., Chicago, IL). Differences with P < 0.05 were considered to be statistically significant in this study.
Results
The extraction of RNA and the amplification of the target genes
The A 260/A 280 ratio of total RNA extracted was between 1.8 and 2.0 for all samples. The results of agarose gel electrophoresis showed that the bands of 18S and 28S were clear and complete, indicated that the total RNA had good quality and high purity (data not shown). For gel electrophoresis, 5 μl of RT-PCR products was used and analyzed. No primer dimers were visible and the amplified ten genes and G3PDH gene fragments with the expected size by RT-PCR were specific as confirmed by gel electrophoresis (Fig. 1).
Agarose gel electrophoresis of RT-PCR products of ten T. spiralis genes. M DNA marker DL2000; lane 1: intermediate filament protein ifa-1 (182 bp); lane 2: ATP synthase F1, beta subunit (185 bp); lane 3: enolase (173 bp); lane 4: multi-cystatin-like domain protein precursor (103 bp); lane 5: calcium-dependent secretion activator (164 bp); lane 6: serine protease precursor (185 bp); lane 7: ribosomal protein S6 kinase beta-1 (141 bp); lane 8: putative serine protease (177 bp); lane 9: serine protease (192 bp); lane 10: secreted 5′-nucleotidase (121 bp); lane 11: glyceraldehyde 3-phosphate dehydrogenase (196 bp) as control
Validation of the comparative Ct (2−ΔΔCt) method for genes mRNA quantification
The validation of the comparative Ct (2−ΔΔCt) method, in order to be feasible, requires two presuppositions. More specifically, the PCR amplification efficiencies of the target and the reference genes should be approximately 100 % and quite equal to each other (Schmittgen and Livak 2008). In the present study, both prerequisites were tested in a validation experiment, in which the Ct values of target genes and G3PDH cDNA amplification were determined in a dilution series of cDNA over a 104-fold range and then plotted against the log cDNA dilution. The Ct values corresponded to the number of cycles at which the fluorescence emission monitored in real time reached a threshold of 10 times the standard deviation of the mean baseline emission from cycles 3 to 15.
The resultant standard curve showed a linear relationship between Ct value and concentration, with an R 2 value above 0.98 (Table 2). The slopes of the target genes and G3PDH amplification plots are similar (between −3.1606 and −3.4438). Applying the formula E (%) = [−1 + 10(−1/α)] × 100, where E (%) is the real-time PCR efficiency for amplification of each gene and α is the slope of the corresponding amplification plot, the values of the efficiencies of the target genes and G3PDH amplification are between 94 % and 108 %. Therefore, both prerequisites for the application of the 2−ΔΔCt method were satisfied.
Real-time PCR analysis of differentially expressed genes of the larvae
Analysis of fold changes in transcript levels in total RNA extracted from bile-activated and cell-cultured larvae was performed using the comparative Ct (2−ΔΔCt) relative quantification (RQ) method of real-time PCR. Values were expressed as RQ index and compared to gene expression level of untreated larvae. The result of the fold differences of the genes is shown in Fig. 2. Compared with untreated ML, four genes were up-regulated in both bile-activated and cell-cultured larvae, including calcium-dependent secretion activator (Csa; 2.55- and 16.04-fold, respectively), multi-cystatin-like domain protein precursor (Mcd; 4.36- and 52-fold, respectively), serine protease (Sp; 2.03 and 20.02, respectively), and intermediate filament protein ifa-1 (Ifa 1; 2 and 3.31, respectively). The expression of two genes, enolase (Eno; 1.51) and ribosomal protein S6 kinase beta-1 (Rsk;1.49), was up-regulated only in the cell-cultured larvae, not in bile-activated larvae. The expression of excreted 5′-nucleotidase (5 N; 1.42) and putative serine protease (Psp; 1.41) was up-regulated in bile-activated larvae, but was not changed or down-regulated after cultured with HCT-8 cells. The transcription levels of ATP synthase F1, beta subunit (ATPase; 0.58- and 0.51-fold, respectively) and serine protease precursor (Spp; 0.42 and 0.65, respectively) were down-regulated in both of bile-activated and cell-cultured larvae.
Real-time PCR analysis of ten T. spiralis genes. Total RNA was isolated from the untreated (U), bile-activated (B) and cell-cultured larvae (C). The fold change in the target genes, normalized to glyceraldehyde 3-phosphate dehydrogenase (G3PDH) and relative to the expression in the larvae, was calculated using comparative Ct (2−ΔΔCt) method. Three-well repeats for each sample were measured and three independent experiments were performed. The value was expressed as the mean ± standard deviation. *P < 0.05: different from the value in the untreated larvae; # P < 0.05: different from the values between the bile-activated and cell-cultured larvae
Discussion
Quantifying gene expression levels has become a staple of most molecular biological laboratories. By measuring the amount of cellular RNA, one is able to determine to what extent that particular gene is being expressed. Real-time PCR is an extremely sensitive, rapid and accurate technique for quantification of the target nucleotides (Whelan et al. 2003; Han et al. 2006; Guenther et al. 2008). The quantitative endpoint for real-time PCR is the threshold cycle (Ct). The numerical value of the Ct is inversely related to the amount of amplicon in the reaction (i.e., the lower the Ct, the greater the amount of amplicon). A widely used method to present relative gene expression is the comparative Ct method also referred to as the 2−ΔΔCt method (Kenneth and Thomas 2001). In the present study, the efficiency of the target gene and G3PDH amplification was firstly calculated and they were between 94 % and 108 %. Therefore, the prerequisites for the gene application by using the 2−ΔΔCt method were satisfied.
Previous studies showed that some proteases in the ES products of T. spiralis ML had collagenolytic and elastolytic activities and might play an important role in the invasion and developmental process of Trichinella larvae (Bolás-Fernandez and Corral-Bezara 2006; Nagano et al. 2009). In the present study, seven of the ten examined T. spiralis proteins are proteases or their precursor. Four (Eno, 5 N, Rsk and ATPase) had binding activity, three (Spp, Sp and Psp) had hydrolase activity, one (Ifa 1) had structural molecule activity, and two (Mcd and Csa) had not be annotated. Out of four proteins with binding activity, 5 N and Rsk had nucleotide binding function, Eno had magnesium ion binding function, and ATPase had nucleotide binding and ATP binding function. 5 N participated in nucleotide catabolic process, and the expression was up-regulated in bile-activated larvae; Eno related with glycolysis and Rsk participated in protein phosphorylation were up-regulated in the cell-cultured larvae; ATPase associated with ATP biosynthetic process was down-regulated in bile activated and the cell-cultured larvae.
5 N is a nucleotide-metabolizing enzyme secreted by the parasitic nematodes. 5 N exhibits unique properties in catalyzing the hydrolysis of nucleoside 5′-diphosphates in addition to nucleoside 5′-monophosphates, but shows no activity against nucleoside 5′-triphosphates. Evidence is provided that 5 N constitutes an enzymatic cascade which catalyzes the degradation of extracellular nucleotides, with a potential physiological role in the regulation of purinergic signaling. Glycolytic enzymes are essential and important for the survival of the parasite. T. spiralis larvae and adults depend largely on anaerobic energy metabolism for their growth and development. The up-regulation of enolase (Eno) could enhance 2-phosphoglycerate conversion to phosphoenolpyruvate. Ribosomal s6 kinase (Rsk) is a family of protein kinases involved in signal transduction. Rsks are serine/threonine kinases and are activated by the MAPK/ERK pathway. The transcriptions levels of the three genes (5 N, Eno and Rsk) may affect the T. spiralis survival and development by affecting the nucleotide catabolic process, carbohydrate metabolism and protein phosphorylation.
ATP synthases are membrane-bound enzyme complexes/ion transporters that combine ATP synthesis and/or hydrolysis with the transport of protons across a membrane. ATPase was also the T. spiralis protein which was identified in the IECs after culture with the larvae (Wang et al. 2012). In this study, the expression of this gene was confirmed to be down-regulated in both bile-activated and cultured larvae. Its biological function in the process of T. spiralis infection is unknown.
Serine proteases are important in a wide variety of biological processes, including digestion, blood coagulation and fibrinolysis. They are enzymes that cleave peptide bonds in proteins, in which serine serves as the nucleophilic amino acid at the enzyme's active site (Hedstrom 2002). In parasites, serine proteases are known to be involved in the invasion of host tissues and cells (Dzik 2006), and in nematodes are likely to be important in molting. Several secreted serine proteases have been identified in T. spiralis ML ES proteins, including the trypsin-like 45-kDa antigen and the serine protease TspSP-1 (Romaris et al. 2002; Robinson and Connolly 2005). In our study, serine protease (Sp) was up-regulated in bile activated and cultured larvae. The results further indicated that Sp might be related with the larval invasion of IECs.
Intermediate filaments are intermediate between the two other principal elements of the cytoskeleton, actin filaments and microtubules. They appear to play basically a structural role by providing mechanical strength to cells and in cell growth (Wang and Stamenović 2000). The up-regulation of Ifa 1 gene expression in bile-activated and cell-cultured larvae might represent the ML cell proliferation.
In comparison to untreated (normal) larvae, the expression of two genes (Mcd and Csa) was increased by 4.36- and 32.05-fold as well as 2.55 and 16.04-fold in bile-activated and cell-cultured larvae, respectively. However, these two proteins were not annotated and thus no information on their function is available. Cystatins are reversible inhibitors of cysteine protease. A number of parasitic nematodes including Brugia malayi, Heamonchus contortus and Onchocerca volvulus, secrete cystatins, which are prominent gene products among parasitic nematode, and that they have evolved features quite different from Caenorhabditis elegans, suggesting that cystatins play an critical role in invasion, and/or immune evasion (Hartmann and Lucius 2003; Newlands et al. 2001; Murray et al. 2005).
Conclusion
The present study provided the mRNA relative expression levels of ten T. spiralis genes in untreated, bile-activated and cell-cultured larvae. The expression levels of eight genes (Csa, Mcd, Sp, Ifa 1, Eno, Rsk, 5 N and Psp) were up-regulated in bile-activated larvae and/or cell-cultured larvae. These gene-encoded proteins might be expressed on the exterior of the parasite and be available for interaction with the host cells, and related with the larval invasion of IECs, but their exact biological functions have not been fully clarified. Further experiments in vitro and in vivo are needed to determine the functions of these genes in the process of T. spiralis invasion and development. This study will be helpful to further elucidate the molecular mechanism of the invasion of IECs by T. spiralis larvae and better understand the interaction between parasite and host enterocytes.
References
Bolás-Fernandez F, Corral-Bezara LD (2006) TSL-1 antigens of Trichinella: an overview of their potential role in parasite invasion, survival and serodiagnosis of trichinellosis. Res Vet Sci 81:297–303
Bruce RG (1970) Structure of the esophagus of the infective juvenile and adult Trichinella spiralis. J Parasitol 56:540–549
Campbell WC (1983) Trichinella and trichinosis. Plenum, New York
Chen X, Yang Y, Yang J, Zhang Z, Zhu X (2012) RNAi-mediated silencing of paramyosin expression in Trichinella spiralis results in impaired viability of the parasite. PLoS One 7:e49913. doi:10.1371/journal.pone.0049913
Cuttell L, Corley SW, Gray CP, Vanderlinde PB, Jackson LA, Traub RJ (2012) Real-time PCR as a surveillance tool for the detection of Trichinella infection in muscle samples from wildlife. Vet Parasitol 188:285–293. doi:10.1016/j.vetpar.2012.03.054
Dzik JM (2006) Molecules released by helminth parasites involved in host colonization. Acta Biochim Pol 53:33–64
Gagliardo LF, McVay CS, Appleton JA (2002) Molting, ecdysis, and reproduction of Trichinella spiralis are supported in vitro by intestinal epithelial cells. Infect Immun 70:1853–1859
Gamble HR, Bessonov AS, Cuperlovic K, Gajadhar AA, van Knapen F, Noeckler K, Schenone H, Zhu X (2000) International Commission on Trichinellosis: recommendations on methods for the control of Trichinella in domestic and wild animals intended for human consumption. Vet Parasitol 93:393–408
Guenther S, Nockler K, Von N, Rosenegk M, Landgraf M, Ewers C, Wieler LH, Schierack P (2008) Detection of Trichinella spiralis, T. britovi and T. pseudospiralis in muscle tissue with real-time PCR. J Microbiol Methods 75:287–292
Han CX, Liu HX, Zhao DM (2006) The quantification of prion gene expression in sheep using real-time RT-PCR. Virus Genes 33:359–364
Hartmann S, Lucius R (2003) Modulation of host immune responses by nematode cystatins. Int J Parasitol 33:1291–302
Hedstrom L (2002) Serine protease mechanism and specificity. Chem Rev 102:4501–4524. doi:10.1021/cr000033x
Jiang P, Wang ZQ, Cui J, Zhang X (2012) Comparison of artificial digestion and Baermann's methods for detection of Trichinella spiralis pre-encapsulated larvae in muscles with low-level infections. Foodborne Pathog Dis 9:27–31. doi:10.1089/fpd.2011.0985
Kang SA, Cho MK, Park MK, Kim DH, Hong YC, Lee YS, Cha HJ, Ock MS, Yu HS (2012) Alteration of helper T-cell related cytokine production in splenocytes during Trichinella spiralis infection. Vet Parasitol 186:319–327. doi:10.1016/j.vetpar.2011.12.002
Kapel CM, Gamble HR (2000) Infectivity, persistence, and antibody response to domestic and sylvatic Trichinella spp. in experimentally infected pigs. Int J Parasitol 30:215–21
Kenneth JL, Thomas DS (2001) Analysis of relative gene expression data using real-time quantitative PCR and the 2−ΔΔCt CT method. Methods 25:402–408
Li F, Cui J, Wang ZQ, Jiang P (2010) Sensitivity and optimization of artificial digestion in the inspection of meat for Trichinella spiralis. Foodborne Pathog Dis 7:879–885
Li CK, Seth R, Gray T, Bayston R, Mahida YR, Wakelin D (1998) Production of proinflammatory cytokines and inflammatory mediators in human intestinal epithelial cells after invasion by Trichinella spiralis. Infect Immun 66:2200–2206
Man Warren T, Gagliardo L, Geyer J, McVay C, Pearce-Kelling S, Appleton J (1997) Invasion of intestinal epithelia in vitro by the parasitic nematode Trichinella spiralis. Infect Immun 65(11):4806–4812
Murray J, Manoury B, Balic A, Watts C, Maizels RM (2005) Bm-CPI- 2, a cystatin from Brugia malayi nematode parasites, differs from Caenorhabditis elegans cystatins in a specific site mediating inhibition of the antigen-processing enzyme AEP. Mol Biochem Parasitol 139:197–203
Nagano I, Wu Z, Takahashi Y (2009) Functional genes and proteins of Trichinella spp. Parasitol Res 104:197–207. doi:10.1007/s00436-008-1248-1
Nagano I, Wu Z, Asano K, Takahashi Y (2011) Molecular cloning and characterization of transgelin-like proteins mainly transcribed in newborn larvae of Trichinella spp. Vet Parasitol 178:134–142
Newlands GF, Skuce PJ, Knox DP, Smith WD (2001) Cloning and expression of cystatin, a potent cysteine protease inhibitor from the gut of Haemonchus contortus. Parasitology 122:371–378
Ren HJ, Cui J, Wang ZQ, Liu RD (2011) Norma l mouse intestinal epithelial cells as a model for the in vitro invasion of Trichinella spiralis infective larvae. PLoS One 6:e27010
Ren HJ, Cui J, Yang W, Liu RD, Wang ZQ (2013a) Identification of differentially expressed genes in Trichinella spiralis larvae after exposure to host intestine milieu. PLoS One 8:e67570. doi:10.1371/journal.pone.0067570
Ren HJ, Liu RD, Wang ZQ, Cui J (2013b) Construction of a Trichinella spiralis phage display library and use for identifying the host enterocyte–parasite interactions. Parasitol Res 112:1857–1863. doi:10.1007/s00436-013-3339-x
Robinson MW, Connolly B (2005) Proteomic analysis of the excretory–secretory proteins of the Trichinella spiralis L1 larva, a nematode parasite of skeletal muscle. Proteomics 5:4525–4532
Romaris F, North SJ, Gagliardo LF, Butcher BA, Ghosh K, Beiting DP, Panico M, Arasu P, Dell A, Morris HR, Appleton JA (2002) A putative serine protease among the excretory–secretory glycoproteins of L1 Trichinella spiralis. Mol Biochem Parasitol 122:149–160
Schmittgen TD, Livak KJ (2008) Analyzing real-time PCR data by the comparative C (T) method. Nat Protoc 3:1101–1108
Stewart GL, Despommier DD, Burnham J, Raines KM (1987) Trichinella spiralis: behavior, structure, and biochemistry of larvae following exposure to components of the host enteric environment. Exp Parasitol 63:195–204
Theodoropoulos G, Petrakos G (2010) Trichinella spiralis: differential effect of host bile on the in vitro invasion of infective larvae into epithelial cells. Exp Parasitol 126:441–444
Theodoropoulos G, Prokou M, Georgiadou V, Petrakos M, Webster P, Kapel CM (2005) Effects of raw biles and their non-protein fractions from fox, pig, sheep and chicken on the survival of Trichinella spp. in vitro. Vet Parasitol 132:63–67
Wang N, Stamenović D (2000) Contribution of intermediate filaments to cell stiffness, stiffening, and growth. Am J Physiol Cell Physiol 279:C188–194
Wang L, Cui J, Wang SW, Wang ZQ (2010) Observation of the in vitro invasion of intestinal epithelial cells by Trichinella spiralis larvae and their development in those cells. J Pathog Biol 5:901–903
Wang SW, Wang ZQ, Cui J (2011) Protein change of intestinal epithelial cells induced in vitro by Trichinella spiralis infective larvae. Parasitol Res 108:593–599. doi:10.1007/s00436-010-2102-9
Wang ZQ, Wang L, Cui J (2012) Proteomic analysis of Trichinella spiralis proteins in intestinal epithelial cells after culture with their larvae by shotgun LC-MS/MS approach. J Proteomics 75:2375–2383. doi:10.1016/j.jprot.2012.02.005
Wang L, Wang ZQ, Cui J (2013a) Proteomic analysis of the changed proteins of Trichinella spiralis infective larvae after co-culture in vitro with intestinal epithelial cells. Vet Parasitol 194:160–163. doi:10.1016/j.vetpar.2013.01.045
Wang L, Wang ZQ, Cui J (2013b) Protein changes of Trichinella spiralis muscle larvae in vitro induced by bovine bile. Vet Parasitol 194:164–167. doi:10.1016/j.vetpar.2013.01. 046
Whelan JA, Russell NB, Whelan MA (2003) A method for the absolute quantification of cDNA using real-time PCR. J Immunol Methods 278:261–269
Yang Y, Jian W, Qin W (2010) Molecular cloning and phylogenetic analysis of small GTPase protein Tscdc42 from Trichinella spiralis. Parasitol Res 106:801–808. doi:10.1007/s00436-010-1735-z
Acknowledgements
This work was supported by the National Natural Science Foundation of China (No. 81271860, 81371843 and 30972579) and the specialized research fund for the doctoral universities of China (20124101110005).
Author information
Authors and Affiliations
Corresponding authors
Rights and permissions
About this article
Cite this article
Liu, R.D., Wang, Z.Q., Wang, L. et al. Analysis of differentially expressed genes of Trichinella spiralis larvae activated by bile and cultured with intestinal epithelial cells using real-time PCR. Parasitol Res 112, 4113–4120 (2013). https://doi.org/10.1007/s00436-013-3602-1
Received:
Accepted:
Published:
Issue Date:
DOI: https://doi.org/10.1007/s00436-013-3602-1