Abstract
Purpose
High-mobility group box 1 (HMGB1) has been implicated in a variety of biologically important processes, including transcription, DNA repair, V(D)J recombination, differentiation, development, and extracellular signaling. The increased expression of HMGB1 has been described in colorectal cancer (CRC). However, there is no report about the correlation of HMGB1 with clinicopathologic features, including the survival of patients with CRC.
Methods
In present study, we investigated HMGB1 expression and its prognostic significance in CRC by performing immunohistochemical analysis, using a total of 192 paraffin-embedded archival CRC samples. Moreover, disruption of endogenous HMGB1 protein through a siRNA knockdown technique was performed to investigate the possible role of HMGB1 in the process of tumor invasion and metastasis.
Results
Overexpression of HMGB1 was shown in 55.7% cases. Statistical analysis showed that HMGB1 expression was positively correlated with tumor invasion (P = 0.048), lymph node metastasis (P = 0.011), distant metastasis (P = 0.031), and Duke’s stage (P = 0.029) of CRC patients. Patients with higher HMGB1 expression had shorter overall survival time, whereas patients with lower level of HMGB1 had better survival. Multivariate analysis suggested that HMGB1 expression might be an independent prognostic indicator for the survival of patients with CRC. Disruption of endogenous HMGB1 protein through a siRNA knockdown technique was shown to suppress substantially the invasion ability of SW620 cells.
Conclusions
Our results suggest that HMGB1 protein is a valuable marker for progression of CRC patients. High HMGB1 expression is associated with poor overall survival in patients with CRC.
Similar content being viewed by others
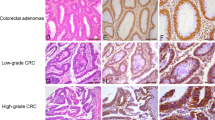
Avoid common mistakes on your manuscript.
Introduction
Colorectal cancer (CRC) is one of the most common malignancies in the world, and represents the second most common cause for cancer deaths in western countries (Jemal et al. 2006). In China, CRC occupies the fifth position in the mortalities caused by cancer, and its incidence still continues to increase (You et al. 2002). Despite significant improvements in the treatments for patients with CRC over the last decade, cases with metastatic cancer frequently result in death (Gutman and Fidler 1995). Metastasis is one of the major factors for the poor outcome. Therefore, it is critical for us to advance in early diagnosis, before metastasis in distant organs occurs, in order to increase the survival rate of patients with CRC. Recent research revealed that the high-mobility group box 1 (HMGB1) may play an important role in the process of tumor invasion and metastasis (Wu et al. 2008; Chung et al. 2009; Sharma et al. 2008).
High-mobility group box 1, an important member of the high-mobility group protein superfamily, has been implicated in a variety of biologically important processes, including transcription, DNA repair, V(D)J recombination, differentiation, development, and extracellular signaling (Czura et al. 2001). As a nuclear protein, HMGB1 was originally characterized as a non-histone, nuclear DNA-binding protein which binds non-specifically to the minor groove of DNA and facilitates the assembly of site-specific DNA-binding proteins, such as p53 and nuclear factor kappa B (NF-κB), at their cognate binding sites within chromatin (Naghavi et al. 2003). Subsequently, HMGB1 was proved to serve as a cytokine that mediates late lethal systemic inflammation via its extracellular release from activated macrophages/monocytes and cells undergoing necrosis (Palumbo et al. 2004; Scaffidi et al. 2002; Wang et al. 1999). Furthermore, recent studies indicated that the overexpression of HMGB1 inhibit apoptosis of cancer cells and related to KIT mutation and genes related to tumor growth and invasion (Volp et al. 2006; Choi et al. 2003). Increased expression of HMGB1 with its receptor, receptor for advanced glycation end products (RAGE), was associated with the proliferation and metastasis of many tumor types, including breast cancer (Palumbo et al. 2004), hepatocellular carcinoma (Scaffidi et al. 2002), melanoma (Wang et al. 1999), Prostate cancer (Ishiguro et al. 2005), gastric cancer (Volp et al. 2006), and colorectal cancer (Choi et al. 2003). However, there is no report about the possible role of increased expression of HMGB1 in progression of CRC.
Here, we investigated the correlation of HMGB1 with clinicopathologic features, including the survival of patients with CRC. Our results provide strong evidence that overexpression of HMGB1 correlated with tumor progression and poor prognosis in CRC. Moreover, our results also suggested that HMGB1 was involved in progression of CRC by promoting invasion and migration of CRC cells. In our studies, HMGB1 was suggested as a new prognostic factor for CRC patients.
Materials and methods
Cell lines
Human colorectal cancer cell lines SW480, SW620, Lovo, DLD-1, HCT-116, and HT-29 from Cell Bank in Chinese Academy of Sciences (Shanghai, China) were cultured in RPMI 1640 medium (Gibco, Grand Island, NY) supplemented with 10% fetal bovine serum (FBS, Hyclone, Logan, UT) and 100 U/ml penicillin/streptomycin (Gibco, Grand Island, NY). All cell lines were maintained in humidified atmosphere with 5% CO2 at 37°C.
Patients and tissue specimens
Formalin-fixed and paraffin-embedded samples of primary CRC (n = 192) were obtained after surgical removal of the tumor from Department of Pathology, the First Affiliated Hospital of Jilin University, China, from 2002 to 2006. All patients were confirmed by histological diagnosis. Histological classification and clinicopathological staging were performed according to the General Rules for Clinical and Pathological Studies on Cancer of the Colon, Rectum, and Anus along with the International Union against Cancer Classification. For the use of these clinical materials for research purposes, prior patient’s consent and approval from the Ethics Committee of Jilin University were obtained. Clinical information about the samples is described in detail in Table 1. The patients included 123 males and 69 females, ranging in age from 28 to 90 years (mean 58.4 years). The median follow-up time for overall survival was 54.8 months for patients still alive at the time of analysis, and ranged from 1 to 102 months. Additional, 95 normal colonic mucosa samples taken from tumor-adjacent tissues were studied as control.
RNA extraction and real-time PCR
Total RNA from cell lines was extracted using Trizol reagent (Invitrogen, Carlsbad, CA,USA) according to the manufacturer’s instructions. DNase I-treated total RNA (1 μg) from each sample was to synthesize cDNA using Superscript II reverse transcriptase (Invitrogen, Carlsbad, CA, USA). The reaction mixture with RNA, 0.5 μg Oligo (dT) and nuclease-free water (the total volume 12 μl) were heated at 65°C for 5 min and then chilled on ice. The other components were added as follows: 4 μl of 5× first strand buffer, 2 μl of 0.1 M DTT, 1 μl of 20 mM dNTPs, and 1 μl of ribonuclease inhibitor. The samples were incubated at 42°C for 2 min, followed by addition of 200 U of superscript II and incubated at 42°C for 50 min. The reaction was inactivated at 70°C for 5 min. Real-time PCR was performed using Mx3000P real-time PCR System (Stratagene, La Jolla, CA, USA) and SYBR Premix Ex TaqTM (Takara, Dalian, China) following the manufacture’s protocol as previous described (Jeon et al. 2004). The annealing temperature was 55°C. The following primers were used for amplifying HMGB1 fragment from cDNA samples of CRC cell lines to confirm the HMGB1 expression: sense primer, 5′-ATATGGCAAAAGCGGACAAG-3′; antisense primer, 5′-GCAACATCACCAATGGACAG-3′. β-Actin was amplified as an internal control using sense primer, 5′-TAAGGAGAAGCTGTGCTACG-3′and antisense primer, 5′-GACTCGTCATACTCCTGCTT-3′. The relative quantification was given by the Ct values, determining the reactions for each target gene and internal control gene (β-actin) in all samples. ∆Ct [∆Ct = Ct(HMGB1) − Ct(β-actin)] values were calculated for each cell line. Relative expression level was determined as 2−∆∆Ct, where ∆∆Ct = ∆Ct (sample) − ∆Ct (control). Here, SW480 cell was regarded as ‘‘control’’. The experiments were repeated in triplicates, and the mean fold changes and standard deviation are reported. All other quantities were expressed as an n-fold difference relative to SW480.
Western blot analysis
Cells were washed twice with cold phosphate-buffered saline and lysed on ice in RIPA buffer (1× PBS, 1% NP40, 0.1% SDS, 5 mM EDTA, 0.5% sodium deoxycholate, and 1 mM sodium orthovanadate) with protease inhibitors. Protein concentration was determined by the Bradford assay (Bio-Rad Laboratories, Hercules, CA, USA). Equal amounts of proteins were separated electrophoretically on 12% SDS/polyacrylamide gels and transferred onto polyvinylidene difluoride membranes (PVDF) (Amersham Pharmacia Biotech, Piscataway, NJ, USA). The membrane was probed with anti-HMGB1 rabbit polyclonal antibody (1:1000; Novus, Littleton, CO, USA). Expression of HMGB1 was determined with horseradish peroxidase-conjugated anti-rabbit immunoglobulin G (Pierce, Rockford, IL, USA) and enhanced chemiluminescence (ECL) (Pierce, Rockford, IL, USA). An anti-β-actin mouse polyclonal antibody (1:400; Santa Cruz, CA, USA) was used to confirm equal loading of proteins.
Immunohistochemistry and evaluation of staining
Immunohistochemistry (IHC) was performed using a Dako Envision System (Dako, Carpinteria, CA, USA) following the manufacturer’s recommended protocol to study protein expression in 192 human CRC tissues. Antibodies against HMGB1 (Novus, Littleton, CO, USA) at a dilution of 1:100 was used in the present study. For negative controls, the antibody was replaced by normal goat serum. The immunohistochemically stained tissue sections were reviewed and scored separately by two pathologists blinded to the clinical parameters. HMGB1 staining was assessed by a relatively simple, reproducible scoring method (Soumaoro et al. 2004). The staining intensity was scored as 0 (negative), 1 (weak), 2 (medium), and 3 (strong). Extent of staining was scored as 0 (0%), 1 (1–25%), 2 (26–50%), 3 (51–75%), and 4 (76–100%), according to the percentages of the positive staining areas of tumor cells or normal colonic cells in relation to the whole carcinoma area or entire section for the normal samples. The sum of the intensity and extent score was used as the final staining score (0 to 7) for HMGB1. This relatively simple, reproducible scoring method gives highly concordant results between independent evaluators and has been used in previous studies (Soumaoro et al. 2004; Masunaga et al. 2000). Tumors having a final staining score of 3 or higher were considered to be positive.
Plasmid generation
For construction of HMGB1 knockdown vectors in mammalian cells, two human siRNAs (S1: 5′-GCAAGTATTCGGTGCTATA-3′; S2: 5′-GCTCAAGGAGAATTTGTAA-3′) targeted HMGB1 were selected, and human scrambled siRNA sequence (5′-GCTCTGGAGCAGTTCCGATATC-3′) possessing limited homology to human genes was served as a negative control. The shRNA were synthesized and subcloned into the pGPU6/GFP/Neo shRNA Vector (Genepharma, Shanghai, China).
Transient transfections regulate the expression of HMGB1 gene
SW620 cells were seeded onto six-well plates, and then, transient transfections were performed using the lipofection method with Lipofectamine (Invitrogen, Carlsbad, CA, USA) as a vehicle. Two HMGB1 pGPU6/GFP/Neo shRNA Vector were transiently transfected into the cells according to the manufacturer’s instructions. The plate was incubated for 48 h until it is ready for further assay. The pGPU6/GFP/Neo vector was used as controls for HMGB1-knockdown experiments.
Tumor cell invasion assay
Invasion assays were performed using the cell invasion chamber (Chemicon,Temecula, CA, USA) according to the protocol of the manufacturer. Briefly, warm serum-free media was added to the top chamber of the cell invasion chamber to rehydrate the ECM layer for 2 h at room temperature. Tumor cells in serum-free media (300 μl containing 1 × 105 cells) were added to the top chamber. The bottom chamber was prepared with 10% FBS as a chemoattractant. After 48 h incubation, the non-invasive cells were removed with a cotton swab. The cells that had migrated through the membrane and stuck to the lower surface of the membrane were fixed with methanol and stained with hematoxylin. For quantification, the cells were counted under a microscope in five predetermined fields at ×200.
Statistical analysis
All statistical analyses were carried out using the SPSS 12.0 statistical software package. The Mann–Whitney U test was used to analyze the relationship between HMGB1 expression and clinicopathologic characteristics. Survival curves were plotted by the Kaplan–Meier method and compared by the Kaplan–Meier method and compared by the log-rank test. The significance of various variables for survival was analyzed by the Cox proportional hazards model in the multivariate analysis. Tumor cell invasion assay was tested using one-way ANOVA. P < 0.05 in all cases was considered statistically significant.
Results
Expression analysis of HMGB1 by real-time PCR and Western blot
To determine correlation of HMGB1 with CRC, semi-quantitative real-time PCR and Western blot analysis were performed to evaluate the expression levels of HMGB1 transcripts and protein in six CRC cell lines with different metastatic potential (HCT-116, HT-29, SW480, DLD-1 derived from primary lesion), Lovo, SW620 (derived from metastatic lymph node). Comparing with that in SW480 cell, expression level of HMGB1 mRNA was significantly down-regulated in DLD-1 cell line, and significantly up-regulated in Lovo and HCT-116 cell line, especially in SW620 cells line, which has higher metastatic potential among the CRC cell lines (Fig. 1a). We then examined the protein expression of HMGB1 in six CRC cells. It was also found that HMGB1 protein was highly expressed in SW620 cell lines versus the SW480 cell (Fig. 1b). The expression level of HMGB1 mRNA in six CRC cells was consistent with that of protein on the whole.
Immunohistochemical analysis
In the present study, we focused on the correlation of HMGB1 variable expression with human CRC, so we analyzed HMGB1 protein level in an independent set of 192 paraffin-embedded, archival primary CRC tissues and 95 normal colorectal mucosa tissues by immunohistochemical staining. As shown in Fig. 2, HMGB1 protein was mainly localized to the nuclei and cytoplasm of carcinoma cells. Overexpression of HMGB1 was found in 107 of 192 (55.7%) CRC tissues. HMGB1 staining was weaker in normal adjacent colorectal mucosa epithelial cells than in CRC tissues. The ratio of HMGB1 overexpression in normal tissues was 36.8% (35/95).
Increased HMGB1 protein expression in human clinical samples of CRC. The samples were collected and subjected to immunohistochemical staining with antibody to HMGB1 (DAB staining and hematoxylin counterstaining). a Low expression of HMGB1 in normal colorectal mucosal tissue (original magnification: ×100). b Low expression of HMGB1 in some colorectal tumor tissue samples (original magnification: ×200). c, d High expression of HMGB1 in CRC samples (original magnification: ×200 and ×400, respectively)
Relationship between clinicopathologic features and HMGB1 expression in CRC
The relationship between clinicopathologic features and HMGB1 expression in CRC was summarized in Table 1. No significant associations were found between HMGB1 expression and age, gender, tumor site, tumor size, and tumor differentiation grade of CRC patients (P > 0.05). However, HMGB1 expression was positively correlated with tumor invasion (P = 0.048), lymph node metastasis (P = 0.011), distant metastasis (P = 0.031), and Duke’s stage (P = 0.029) of CRC patients.
Survival analysis and the log-rank test
Using Kaplan–Meier analysis method, we observed that the expression of HMGB1 in CRC was significantly correlated with overall survival (log-rank test statistic = 7.388, P = 0.007, Fig. 3). The median overall survival time were 74 and 45 months, respectively, in patients with low and high HMGB1 expression. The log-rank test showed that the survival time was significantly different between groups with positive and negative expressions of HMGB1, indicating that the high level of HMGB1 was correlated with a shorter survival times.
To determine weather the expression of HMGB1 was an independent prognostic factor of outcomes, multivariate survival analysis, which included lymph node metastasis, distant metastasis, Duke’s stage and HMGB1 expression, was done. Results showed that the expression of HMGB1 protein was recognized as an independent prognostic factor of outcomes of CRC patients (Table 2). Thus, our findings revealed that HMGB1 protein expression level had a significant correlation with prognosis of CRC.
HMGB1 expression and cell invasion in human CRC
To further determine whether HMGB1 expression plays a role in human CRC cell invasion, a RNA interference technique was used to knockdown endogenous HMGB1. After introduction of HMGB1 shRNA into SW620 cells, HMGB1 protein was shown to be reduced 48 h after transfection and non-specific shRNA had no substantial effect on endogenous HMGB1 expression (Fig. 4a). Because the effect of HMGB1 siRNA S1 on silencing HMGB1 protein was superior to HMGB1 siRNA S2, we used HMGB1 siRNA S2 to perform our experiments. In the cell invasion assay, HMGB1 knockdown cells exhibited significantly reduced invasive tendencies compared with cells treated with non-specific siRNA (P < 0.05; Fig. 4b). Therefore, disruption of endogenous HMGB1 expression resulted in suppression of cell invasion in CRC cells.
Effect of HMGB1 on SW620 cells invasion. a Effect of HMGB1 siRNA on endogenous HMGB1 expression in SW620 cells. b Effect of decreased HMGB1 expression on cell invasive ability by an in vitro invasion assay. After 48 h, SW620 cells that migrated through the filters were counted in five randomly selected fields. Each value represents the average of triplicate determinations expressed as the mean ± SE. The asterisks denote data that is significantly different from the control and non-specific siRNA (P < 0.05; ANOVA test with LSD post hoc test)
Discussion
In the present study, we show that HMGB1 was overexpressed at both transcriptional and translational levels in metastatic CRC cell lines (Lovo and SW620), compared with primary CRC cell lines (HCT-116, HT-29, SW480, DLD-1). Furthermore, using an immunochemical staining approach, we found that approximately 55.7% (107 of 192 cases) of CRC tissues overexpressed HMGB1 protein. HMGB1 overexpression was significantly associated with tumor invasion (P = 0.048), lymph node metastasis (P = 0.011), distant metastasis (P = 0.031), and Duke’s stage (P = 0.029) of CRC patients, but not with patient gender, age tumor site, tumor size, and differentiation grade.
Further, we showed that high expression of HMGB1 protein may serve as a potential prognostic factor for CRC patients by univariate and multivariate analyses. Our results indicated that HMGB1 protein expression was inversely correlated with overall survival. The CRC patients with a higher expression of HMGB1 protein had a shorter survival time. In multivariate analyses, a high expression of HMGB1 protein was a significant predictor of poor prognosis for CRC patients. This result indicated that CRC might be a potential important prognostic factor for late-stage CRC patients. Further studies are needed to verify these findings to establish HMGB1 as one of the reliable clinical predictors of outcome in CRC and to clarify its role in carcinogenesis by functional analysis.
Recently HMGB1 expression was also found to serve as an unfavorable prognostic factor in breast cancer, ovarian cancer, nasopharyngeal cancer, and gastric cancer (Wu et al. 2008; Chung et al. 2009; Meyer et al. 2008). Our study revealed HMGB1 as a prognostic factor in CRC. However, the possible mechanism and the exact mode of HMGB1 action during tumor metastasis and progression in CRC are still largely unknown.
The role of HMGB1 in tumor metastasis and its related mechanism has been a subject of great interest in recent years (Wu et al. 2008; Chung et al. 2009; Sharma et al. 2008; Czura et al. 2001; Palumbo et al. 2004; Volp et al. 2006). Several recent studies have demonstrated that the interaction of HMGB1 with its specific receptor RAGE activates several intracellular signal transduction pathways including MAPK (mitogen-activated protein kinases), Rac, Cdc42, and NF-κB (Lotze and Tracey 2005; Huttunen et al. 1999; Kuniyasu et al. 2003a, b). HMGB1 overexpression could promote cell motility, invasiveness, proliferation, and angiogenesis in cancer progression. Because HMGB1 is known as metastasis associated gene in other kinds of cancer, we speculated HMGB1 might play a role in CRC by promoting invasion and metastasis of CRC cells. So, an in vitro invasion assay was performed to investigate the effects of HMGB1 on invasion of CRC cells. We found that disruption of endogenous HMGB1 protein through a siRNA knockdown technique was shown to suppress substantially the invasion ability of SW620 cells. This result supports the role of HMGB1 in CRC might be associated with its effects on invasion, similar to the results observed in other cancers previously (Kuniyasu et al. 2003a, b).
In conclusion, our study demonstrated that the level of expression of HMGB1 was highly increased in CRC and HMGB1 might play an important role in CRC by promoting invasion and migration of CRC cells. Moreover, HMGB1 expression might serve as an unfavorable prognostic factor in CRC cancer patients. The findings in the present study may lead to the development of new approaches for effective diagnosis and therapy of CRC. However, these findings still need to be replicated, and further investigation in another patient population is required to verify these conclusions. More works are needed in the future study to clarify the mechanism by which HMGB1 is involved in the development and progression of CRC and its related pathway in CRC.
References
Choi YR, Kim H, Kang HJ, Kim NG, Kim JJ, Park KS, Paik YK, Kim HO, Kim H (2003) Overexpression of high mobility group box 1 in gastrointestinal stromal tumors with KIT mutation. Cancer Res 63:2188–2193
Chung HW, Lee SG, Kim H, Hong DJ, Chung JB, Stroncek D, Lim JB (2009) Serum high mobility group box-1 (HMGB1) is closely associated with the clinical and pathologic features of gastric cancer. J Transl Med 7:38
Czura CJ, Wang H, Tracey KJ (2001) Dual roles for HMGB1: DNA binding and cytokine. J Endotoxin Res 7:315–321
Gutman M, Fidler IJ (1995) Biology of human colon cancer metastasis. World J Surg 19:226–234
Huttunen HJ, Fages C, Rauvala H (1999) Receptor for advanced glycation end products (RAGE)-mediated neurite outgrowth and activation of NF-kappaB require the cytoplasmic domain of the receptor but different downstream signaling pathways. J Biol Chem 274:19919–19924
Ishiguro H, Nakaigawa N, Miyoshi Y, Fujinami K, Kubota Y, Uemura H (2005) Receptor for advanced glycation end products (RAGE) and its ligand, amphoterin are overexpressed and associated with prostate cancer development. Prostate 64:92–100
Jemal A, Siegel R, Ward E, Murray T, Xu J, Smigal C, Thun MJ (2006) Cancer statistics, 2006. CA Cancer J Clin 56:106–130
Jeon GA, Lee JS, Patel V, Gutkind JS, Thorgeirsson SS, Kim EC, Chu IS, Amornphimoltham P, Park MH (2004) Global gene expression profiles of human head and neck squamous carcinoma cell lines. Int J Cancer 112:249–258
Kuniyasu H, Chihara Y, Takahashi T (2003a) Co-expression of receptor for advanced glycation end products and the ligand amphoterin associates closely with metastasis of colorectal cancer. Oncol Rep 10:445–448
Kuniyasu H, Chihara Y, Kondo H (2003b) Differential effects between amphoterin and advanced glycation end products on colon cancer cells. Int J Cancer 104:722–727
Lotze MT, Tracey KJ (2005) High-mobility group box 1 protein (HMGB1): nuclear weapon in the immune arsenal. Nat Rev Immunol 5:331–342
Masunaga R, Kohno H, Dhar DK, Ohno S, Shibakita M, Kinugasa S, Yoshimura H, Tachibana M, Kubota H, Nagasue N (2000) Cyclooxygenase-2 expression correlates with tumor neovascularization and prognosis in human colorectal carcinoma patients. Clin Cancer Res 6:4064–4068
Meyer A, Staratschek-Jox A, Springwald A, Wenk H, Wolf J, Wickenhauser C, Bullerdiek J (2008) Non-Hodgkin lymphoma expressing high levels of the danger-signalling protein HMGB1. Leuk Lymphoma 49:1184–1189
Naghavi MH, Nowak P, Andersson J, Sonnerborg A, Yang H, Tracey KJ, Vahlne A (2003) Intracellular high mobility group B1 protein (HMGB1) represses HIV-1 LTR-directed transcription in a promoter- and cell-specific manner. Virology 314:179–189
Palumbo R, Sampaolesi M, De Marchis F, Tonlorenzi R, Colombetti S, Mondino A, Cossu G, Bianchi ME (2004) Extracellular HMGB1, a signal of tissue damage, induces mesoangioblast migration and proliferation. J Cell Biol 164:441–449
Scaffidi P, Misteli T, Bianchi ME (2002) Release of chromatin protein HMGB1 by necrotic cells triggers inflammation. Nature 418:191–195
Sharma A, Ray R, Rajeswari MR (2008) Overexpression of high mobility group (HMG) B1 and B2 proteins directly correlates with the progression of squamous cell carcinoma in skin. Cancer Invest 26:843–851
Soumaoro LT, Uetake H, Higuchi T, Takagi Y, Enomoto M, Sugihara K (2004) Cyclooxygenase-2 expression: a significant prognostic indicator for patients with colorectal cancer. Clin Cancer Res 10:8465–8471
Volp K, Brezniceanu ML, Bosser S, Brabletz T, Kirchner T, Gottel D, Joos S, Zornig M (2006) Increased expression of high mobility group box 1 (HMGB1) is associated with an elevated level of the antiapoptotic c-IAP2 protein in human colon carcinomas. Gut 55:234–242
Wang H, Bloom O, Zhang M, Vishnubhakat JM, Ombrellino M, Che J, Frazier A, Yang H, Ivanova S, Borovikova L, Manogue KR, Faist E et al (1999) HMG-1 as a late mediator of endotoxin lethality in mice. Science 285:248–251
Wu D, Ding Y, Wang S, Zhang Q, Liu L (2008) Increased expression of high mobility group box 1 (HMGB1) is associated with progression and poor prognosis in human nasopharyngeal carcinoma. J Pathol 216:167–175
You WC, Jin F, Devesa S, Gridley G, Schatzkin A, Yang G, Rosenberg P, Xiang YB, Hu YR, Li Q (2002) Rapid increase in colorectal cancer rates in urban Shanghai, 1972–97, in relation to dietary changes. J Cancer Epidemiol Prev 7:143–146
Acknowledgments
This work was supported by the Canada China University Linkage Program (CCULP) (2009) 010.
Conflict of interest statement
The authors of this article declared that no conflicts of interest were declared.
Author information
Authors and Affiliations
Corresponding author
Rights and permissions
About this article
Cite this article
Yao, X., Zhao, G., Yang, H. et al. Overexpression of high-mobility group box 1 correlates with tumor progression and poor prognosis in human colorectal carcinoma. J Cancer Res Clin Oncol 136, 677–684 (2010). https://doi.org/10.1007/s00432-009-0706-1
Received:
Accepted:
Published:
Issue Date:
DOI: https://doi.org/10.1007/s00432-009-0706-1