Abstract
Purpose
We compared high- and low-intensity eccentric cycling (ECC) with the same mechanical work for changes in muscle function and muscle soreness, and examined the changes after subsequent high-intensity ECC.
Methods
Twenty men performed either high-intensity ECC (1 min × 5 at 20% of peak power output: PPO) for two bouts separated by 2 weeks (H–H, n = 11), or low-intensity (4 min × 5 at 5% PPO) for the first and high-intensity ECC for the second bout (L–H, n = 9). Changes in indirect muscle damage markers were compared between groups and bouts.
Results
At 24 h after the first bout, both groups showed similar decreases in maximal isometric (70° knee angle, − 10.6 ± 11.8%) and isokinetic ( − 11.0 ± 8.2%) contraction torque of the knee extensors (KE), squat ( − 7.7 ± 10.4%) and counter-movement jump ( − 5.9 ± 8.4%) heights (p < 0.05). Changes in KE torque and jump height were smaller after the second than the first bout for both the groups (p < 0.05). Increases in plasma creatine kinase activity were small, and no significant changes in vastus lateralis or intermedius thickness nor ultrasound echo-intensity were observed. KE soreness with palpation was greater (p < 0.01) in H–H (peak: 4.2 ± 1.0) than L–H (1.4 ± 0.6) after the first bout, but greater in L–H (3.6 ± 0.9) than H–H (1.5 ± 0.5) after the second bout. This was also found for muscle soreness with squat, KE stretch and gluteal palpation.
Conclusion
The high- and low-intensity ECC with matched mechanical work induced similar decreases in muscle function, but DOMS was greater after high-intensity ECC, which may be due to greater extracellular matrix damage and inflammation.
Similar content being viewed by others
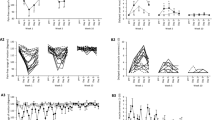
Avoid common mistakes on your manuscript.
Introduction
Lengthening muscle actions (eccentric actions) are performed when activated muscles are lengthened by an external force. It has been well documented that exercises consisting of unaccustomed eccentric actions induce muscle damage (Clarkson et al. 1992). Eccentric exercise-induced muscle damage may be indicated by histological alterations in myofilament structure such as Z-line streaming (Fridén et al. 1983; Armstrong 1990) and associated with delayed-onset muscle soreness (DOMS), prolonged decreases in muscle function, increased ultrasound echo-intensity, muscle swelling and increases in intramuscular proteins in the blood such as creatine kinase (CK) and myoglobin (Clarkson et al. 1992; Nosaka and Clarkson 1995). Repeating the same exercise for several weeks after the initial bout results in smaller changes in these markers when compared with the initial bout, which is referred to as the repeated bout effect (RBE) (Nosaka and Clarkson 1995; Hortobágyi et al. 1998; McHugh 2003).
While many factors affect the magnitude of muscle damage and RBE induced by eccentric exercise, exercise intensity appears to be a key factor; i.e., higher-intensity eccentric actions induce greater damage than lower-intensity ones (Nosaka and Newton 2002; Chen et al. 2007, 2012; Hasenoehrl et al. 2017; Tsuchiya et al. 2019). For example, Nosaka et al. (2002) compared the effects of 30 eccentric actions of the elbow flexors that were performed maximally to those performed at 50% of maximal voluntary isometric contraction (MVIC) torque by young men, and reported that changes in MVIC torque, muscle soreness and plasma CK activity were significantly greater following the maximal exercise. This was also observed in other studies in which maximal and submaximal intensities were compared after elbow flexor eccentric exercise (Chen et al. 2007, 2012; Hasenoehrl et al. 2017; Tsuchiya et al. 2019). However, the exercise mechanical work was not matched between conditions in these studies, so it is not explicitly clear whether the intensity or the mechanical work was the critical factor influencing the magnitude of damage and DOMS.
To the best of our knowledge only three studies have matched exercise mechanical work to examine the effect of eccentric action intensity on muscle damage. Gauche et al. (2009) compared the effects of 15 eccentric actions of the elbow flexors at 80% of maximal concentric torque to 30 eccentric actions at 40%, and found no difference in the MVIC torque changes after the exercises. Similarly, Hill et al. (2019) compared 36 eccentric actions of the elbow flexors at 80% of maximal eccentric torque to 72 eccentric actions at 40%, and also found no difference in the changes in MVIC torque after exercise. In the knee extensors, Paschalis (2005) compared the effects of 120 maximal eccentric actions to a condition where submaximal (50% of maximal eccentric torque) eccentric actions were performed until equal mechanical work was accomplished. The results revealed no difference in DOMS between conditions, but did show significantly greater changes in MVIC torque and plasma CK activity after the maximal-intensity exercise. When considered together, the current evidence indicates a lack of difference in muscle damage between higher- and lower-intensity eccentric exercises when exercise intensity is submaximal (Gauche et al. 2009; Hill et al. 2019), but that damage may be greater after work-matched maximal-intensity exercise than work-matched submaximal-intensity exercise (Paschalis et al. 2005). Nonetheless, more studies are required to explicitly test this hypothesis.
It should be noted that the total mechanical work of exercise imposed in previous studies was small when compared to eccentric cycling that involves the completion of a large number of eccentric actions at a relatively low intensity. Although eccentric cycling is generally performed at intensities less than 30% of maximal knee extension capacity (Peñailillo et al. 2015b), it induces moderate muscle soreness and function decreases already by 30 min of cycling in which ~ 1800 eccentric actions are performed (Yu et al. 2002; Peñailillo et al. 2013). It, therefore, appears that eccentric cycling is a good exercise model to study the effect of eccentric exercise intensity on muscle damage when the exercise bout consists of a larger number of submaximal eccentric actions, such as hiking, long distance running, and ball sports. It is also ideal that the total mechanical work of exercise (workload × cadence × time) can be easily matched between high- and low-intensity protocols. Using this paradigm, a recent study reported greater muscle soreness, function loss and plasma CK activity after high-intensity eccentric cycling (193 W) when compared to low-intensity (112 W) eccentric cycling in older adults (González-Bartholin et al. 2019). However, the study included four eccentric cycling preconditioning (i.e. familiarisation) sessions, and the same participants performed both exercise intensity conditions. Therefore, the muscle damage profiles would have been strongly affected by the repeated bout and/or preconditioning effects (Nosaka and Clarkson 1995; Chen et al. 2012). Consequently, it is of interest to compare the muscle damage responses to high- and low-intensity eccentric cycling without familiarisation or preconditioning, especially when given that previous studies showed smaller changes in, and faster recovery of, indirect markers of muscle damage after the second than the first eccentric cycling bout (Peñailillo et al. 2013, 2015b; Valladares-Ide et al. 2019).
Given the above, the present study compared high- and low-intensity eccentric cycling performed with the same total mechanical work for changes in indirect markers of muscle damage and DOMS. It was hypothesised that changes in muscle function and soreness would be similar between the two eccentric cycling conditions. The present study also included a second bout of eccentric cycling that was performed 14 days after the first bout, in which high-intensity eccentric cycling was always performed to determine whether low-intensity eccentric cycling in the first bout could confer a protective effect against damage and soreness from a subsequent high-intensity bout. It was hypothesised that the magnitude of changes in markers of muscle damage and soreness would be smaller after the second than the first bout regardless of the intensity used in the first bout.
Methods
Participants and study design
The sample size of the present study was estimated using G*Power (Version 3.1.9.2, Universitat Kiel, Germany) based on the study by Paschalis et al. (2005), who compared high- and low-intensity eccentric knee extensions, showing a greater decrease in MVIC torque after the high- than low-intensity eccentric exercise with an effect size of 1.34. With a power level of 0.8, and an alpha level of 0.05, it was found that a total of 18 participants were required, thus at least 9 participants were recruited in each group. Ethical approval from the Edith Cowan University Human Research Ethics Committee was sought before the study commencement.
All participants were healthy young men who had not performed eccentric exercise in the past 6 months and reported no history of orthopaedic lower limb injuries or any neurological disorders. Each participant signed a written informed consent form and answered medical questionnaires before participating in the study. The participants were randomly allocated to one of two groups, H–H and L–H, based on their peak power output during a maximal 10-s isokinetic concentric cycling bout at 60 rpm (PPO) on a cycle ergometer. The participants in H–H performed the same high-intensity eccentric cycling exercise in two bouts separated by 14 days, whereas L–H performed low-intensity eccentric cycling exercise in the first bout and high-intensity eccentric cycling exercise of equal mechanical work in the second bout 14 days later. As shown in Table 1, no significant between-group differences were found for any physical or physiological characteristics at baseline.
The dependent variables, in the order of measurement in the study, included plasma CK activity, knee extensor ultrasound echo intensity and muscle thickness, muscle soreness, squat (SJ) and counter-movement jump (CMJ), peak power output (PPO), knee extensor maximal voluntary isometric contraction torque (MVIC) at 70° and 20° of knee flexion (0° = full extension), rate of force development (RFD) during MVIC at 70°, and knee extensor isokinetic maximal voluntary concentric torque at 270°/s, 180°/s and 90°/s (MVCC270, MVCC180, MVCC90). These were measured before, immediately after, and 24, 48 and 72 h after each exercise bout. To warm-up for the performance tests, the participants completed a 2-min concentric cycling warm-up at 50 W before the jump tests. Five days before the initial bout, the participants completed a familiarisation session to experience all the measurements shown above, but they did not perform the eccentric cycling to avoid a possible protective effect against muscle damage.
Eccentric cycling
Using an eccentric cycle ergometer (Grucox Eccentric Trainer, Grucox, South Africa), participants completed a 2-min concentric cycling warm-up at 50 W after an appropriate seat position was determined by the investigator in relation to their leg length. In the first bout, participants in the H–H performed high-intensity eccentric cycling at 20% PPO for five sets of 1 min with a 1-min rest between sets, and those in L–H performed low-intensity eccentric cycling at 5% of PPO for five sets of 4 min with a 1-min rest between sets, to achieve an equal mechanical work. In all cycling sessions, the cadence was set at 60 rpm. These protocols were chosen based on our pilot studies showing that the same mechanical work was achieved with a distinct difference in intensity in a similar exercise fashion (interval exercise). In the second session that was performed 14 days after the first bout, the same PPO value was used, however H–H repeated the same exercise whereas L–H performed high-intensity eccentric cycling with mechanical work equal to that achieved in the low-intensity eccentric cycling session. This was accomplished by decreasing the duration of each set from 5 to 1 min but increasing the intensity from 5 to 20% PPO.
Each participant was given 1 min of cycling familiarisation immediately before both the initial and secondary exercise bouts, during which eccentric cycling was performed from 30 rpm reaching 60 rpm at the end of the minute for a target power output. The participants were instructed to resist the pedals using the lower limbs to perform smooth backward rotations while maintaining their target power, which was shown on a computer screen set in front of the ergometer. The pedal straps were removed from the ergometer to ensure the participants only pushed against the pedals and did not pull when the pedals were moving away from them to generate power using their knee and hip flexors.
Heart rate during cycling was continuously recorded by a heart rate monitor (Polar S810i, Polar Electro, Finland). Rate of perceived effort was assessed at the end of each set using a modified version of Borg’s category-ratio scale (Borg 1998), as it has been reported that measuring the effort during eccentric cycling is more accurate than exertion (Peñailillo et al. 2018). At the end of each set, the participants were asked to rate their physical effort required by their knee extensors to maintain the target power.
Maximal voluntary isometric (MVIC), concentric (MVCC) contraction torque and rate of force development (RFD)
An isokinetic dynamometer (System 3, Biodex Medical Systems, USA) was used for the measurements of MVIC and MVCC and RFD. During measurements, visual feedback of torque was displayed on a computer screen using software connected to the dynamometer (LabVIEW, National Instruments, Australia). MVIC torque was measured from the right knee extensors at knee joint angles of 70° and 20° (MVIC70 and MVIC20, 0° = full knee extension) after several submaximal contractions such as warm-up. Three 4-s maximal voluntary isometric knee extensions were performed at each joint angle, separated by 1 min of passive rest. Participants were advised not to perform any counter-movement motion before the muscle action, and were instructed to “exert force as fast and then as hard as possible” (Maffiuletti et al. 2016). The highest torque value was used for further analysis.
RFD has been identified as a more sensitive measure of exercise-induced muscle damage than MVIC (Peñailillo et al. 2015a). RFD at 70° knee angle was assessed in the time periods of 0–100 ms (RFD0–100) and 100–200 ms (RFD100–200), where the onset of force production was at 0 s. The onset of force production was identified as the point at which torque data exceeded the baseline by > 7.5 Nm (Peñailillo et al. 2015a). RFD was not measured within shorter time intervals because the data were not of sufficient reliability (e.g. Maffiuletti et al. 2016). If the torque decreased more than 5 Nm below the baseline immediately before torque onset, a counter-movement is considered to have occurred, and the trial was not considered for analysis. The contractions with the highest RFD values were selected for further analysis.
Maximal concentric knee extensor torque (MVCC) was also measured on the isokinetic dynamometer at the angular velocities of 270°/s (MVCC270), 180°/s (MVCC180) and 90°/s (MVCC90), in this order. Each contraction started at a knee angle of 90° before extending the knee to 20° before flexing again to 90° as fast and as hard as possible for four consecutive contractions. The three trials (sets) were separated by 1 min of passive rest. The highest torque value was used for further analysis.
Concentric cycling sprint peak power output (PPO)
Participants performed a PPO test on the cycle ergometer as described above. Power data during the sprint were recorded at a frequency of 40 Hz, and the maximal power achieved was calculated from the extracted data from the ergometer.
Squat jump (SJ) and counter-movement jump (CMJ)
SJ and CMJ tests were performed on a force platform (9290AD, Kistler Instruments, Switzerland). SJ was performed from a self-selected knee joint angle, and CMJ was performed from an upright (standing) position. The participant was asked to keep their hands on their hips throughout the jump, to land with their toes first followed by their heel, and to not bend their knees while in air. They were instructed to maintain the same technique for the jump tests, and to jump as high as possible. Each participant performed three jumps, and the peak jump height was selected for further analysis.
Muscle soreness
The magnitude of knee extensor soreness was assessed by palpation, stretch and movement of the knee extensors using a custom 0 (no soreness) – 10 (maximal soreness) scale model. Each participant laid supine on a massage table and the investigator palpated the proximal, middle and distal points of vastus medialis, vastus lateralis, and rectus femoris while the middle gluteus maximus was palpated with the participant in the prone position. The average values of the nine knee extensor palpation points were used for subsequent statistical analysis. The investigator then brought the right lower limb to full knee flexion to assess the muscle soreness during stretch. Participants were then asked to sit down on and stand up from a chair slowly (~ 3 s for each direction) for the movement-based assessment of muscle soreness.
Muscle ultrasound echo-intensity and thickness
Ultrasound images of vastus lateralis and intermedius were taken to measure muscle thickness and echo intensity using a real-time B-mode ultrasound apparatus (Aloka SSD-alpha10, Aloka Co., Japan). The ultrasound settings including focus, contrast and gain were kept the same between measurements. An ultrasound probe (60-mm, linear array) was placed on a marked site at the middle point between the lateral epicondyle and greater trochanter of the right leg, while the participant was lying on a massage table. Three transverse images of each muscle were obtained and then transferred to a computer for the assessment of muscle thickness and mean echo intensity of a greyscale histogram (0: black, 256: white) using an image analysis software (ImageJ, National Institute of Health, USA). The average value of the three images was used for subsequent statistical analysis.
Plasma CK activity
A venous blood sample was obtained at baseline, and 24, 48 and 72 h post-exercise, before any other testing was conducted. Two 6-mL blood samples were collected in EDTA tubes from an antecubital vein. Immediately after sampling, a 30-μL whole blood sample was used for plasma CK activity analysis using a Reflotron (Roche Diagnosis, Germany).
Statistical analyses
A two-way (group × time) repeated measure analysis of variance (ANOVA) was used to compare between groups for changes in the dependent variables over time, and between bouts in each group. The data were assessed for assumptions of normality by analysing the standardized residuals using a Shapiro–Wilk test, and for sphericity by Mauchly’s sphericity test. Ordinal data (muscle soreness and perceived effort values) were rank-transformed before analysis (Wobbrock et al. 2011). In the case of a significant interaction effect, a Holm’s sequential Bonferroni procedure was performed to identify possible differences between groups at each time point. The significance level was set at p ≤ 0.05. All statistical testing was performed using SPSS v25 (IBM, USA). Data are presented as mean ± standard deviation (SD).
Results
Eccentric cycling intensity, mechanical work and effort
Average eccentric cycling intensity was 154.0 ± 25.0 W for the first and second bouts in H–H, and was 36.0 ± 4.9 W in the first bout and 143.4 ± 22.6 W in the second bout in L–H. The total mechanical work (in joules, calculated by power × seconds) performed in the first bout was 46,200.0 ± 7,715.3 J in H–H and 43,140.0 ± 5,839.3 J in L–H without a significant (p = 0.340) difference between groups. During the second bout, the total mechanical work for H–H and L–H were 46,200.0 ± 7,715.3 J and 43,006.7 ± 6,791.5 J, respectively, without a significant difference between groups (p = 0.322) or between bouts in either group (p = 0.944).
Average heart rate was greater in H–H (130 ± 17 bpm) than L–H (108 ± 19 bpm) in the first bout (p < 0.001), and in L–H (134 ± 18 bpm) than H–H (120 ± 12 bpm) in the second bout (p < 0.01); a significant bout × time interaction effect was observed for heart rate in L–H (p < 0.001). Average perceived effort was greater in H–H (5.1 ± 2.2) than L–H (3.0 ± 0.9) in the first bout (p < 0.05), and was also greater in L–H (6.4 ± 0.5) than H–H (4.2 ± 0.4) in the second bout (p < 0.05); a significant bout × time interaction effect was observed for L–H (p < 0.001).
Muscle function
As shown in Fig. 1, most muscle function measures showed significant changes over time, but no significant differences between H–H and L–H were observed for the changes after the first or second bouts. MVIC70 torque was reduced immediately ( − 13.5 ± 8.6%) and 24 h after the first bout ( − 10.6 ± 11.8%) for both groups, and this was similar after the second bout ( − 9.5 ± 12.3%, − 9.1 ± 15.8%, respectively) (Fig. 1a), and this was also the case for MVIC20 torque. Similarly, no significant differences between groups were observed for the changes in MVCC90 torque after the first (e.g., 24 h post-exercise: − 11.0 ± 8.2%) and second ( − 7.8 ± 14.5%) bouts (Fig. 1b), which was also found for MVCC180 and MVCC270 torques. Between bouts, H–H showed a significant interaction effect (p < 0.05) only for MVCC90 torque, but no significant difference between bouts was found for L–H, or for any other measure.
Maximal voluntary isometric contraction torque at 70° (a mean ± SD), isokinetic (90°/s) concentric contraction torque (b), RFD 0–100 ms (c), RFD 100–200 ms (d), squat jump power (e), and counter-movement jump power (f) before (pre), immediately after (post), and 24–72 h after the first and second exercise bouts in H–H (filled circle) and L–H (unfilled circle). *Significant (p < 0.05) difference from the baseline for both groups. ANOVA results for interaction (bout × time) effect are shown in the legend for each bout. Results of comparisons between groups and between bouts are shown on the top of each figure
Regarding RFD, no significant between-group differences were observed for changes in RFD0–100 (Fig. 1c) or RFD100–200 (Fig. 1d) after the first or second bouts. No significant differences in the changes were evident between bouts in each group. No significant differences were found for the first bout in H–H and the second bout in L–H in which both groups performed the high-intensity eccentric cycling for the first time.
SJ decreased significantly from baseline at 24 h post-cycling for both groups ( − 7.7 ± 10.4%) and CMJ decreased from baseline at 24 ( − 5.9 ± 8.4%), 48 ( − 6.2 ± 11.1%) and 72 h after cycling ( − 5.2 ± 10.5%), without a significant difference between groups for the first bout. For the second bout, a significant group × time interaction effect was observed whereby the reductions in jump height from baseline were greater in L–H than H–H. When comparing the first and second bouts in each group, changes were significantly smaller for the H–H group in SJ and CMJ, and for the L–H group in CMJ.
Muscle soreness
Changes in muscle soreness during palpation were similar between the nine different knee extensor sites, thus the average of the nine sites is shown in Fig. 2. Muscle soreness increased at 24–72 h after the first and second bouts in both groups (p < 0.001), and a significant difference between the groups was evident. After the first bout, peak muscle soreness was greater in H–H (4.2 ± 1.0 on 1–10 scale) than L–H (1.4 ± 0.6), but after the second bout it was greater in L–H (3.6 ± 0.9) than H–H (1.5 ± 0.5). When comparing the first and second bouts in each group, a significant interaction effect was found in both groups such that the increases in muscle soreness were smaller after the second bout in H–H but greater after the second bout in L–H, which was smaller when compared with the first bout in H–H. Similar changes were observed for the gluteal muscle soreness with palpation (1st bout; peak H–H: 4.2 ± 3.0, L–H: 0.9 ± 1.9, 2nd bout; peak H–H: 1.2 ± 1.8, L–H: 2.4 ± 2.6), muscle soreness assessed during a squat (1st bout; H–H: 3.8 ± 0.1, L–H: 1.0 ± 0.1, 2nd bout; H–H: 1.0 ± 0.1, L–H: 2.4 ± 0.2) and stretching of the knee extensors (1st bout; H–H: 4.9 ± 2.8, L–H: 2.0 ± 1.4, 2nd bout; H–H: 1.9 ± 1.8, L–H: 3.6 ± 1.7).
Muscle soreness upon palpation (average value of the nine knee extensor sites ± SD) before (pre), immediately after (post), and 24–72 h after the first and second exercise bouts in H–H (filled circle) and L–H (unfilled circle). *Significant (p < 0.05) difference from the baseline for both groups. #Significant (P < 0.05) difference between groups. ANOVA results for interaction (bout × time) are shown in the legend for each bout. Results of comparisons between groups and between bouts are shown on the top of the figure
Plasma CK activity
As shown in Fig. 3, no significant group × time interaction was evident for the changes in plasma CK activity after the first and second bouts, although significant increases were found at 24 h post-exercise in the first bout (average peak CK activity: 248.2 ± 272.5 IU/L), and 24–48 h post-exercise in the second bout in both the groups. When comparing between bouts in each group, the changes were not statistically different for either group.
Plasma creatine kinase (CK) activity (mean ± SD) before (pre), and 24–72 h after the first and second exercise bouts in the H–H (filled circle) and L–H (unfilled circle) groups. *Significant (p < 0.05) difference from the baseline for both groups. ANOVA results for interaction (bout × time) are shown in the legend for each bout. Results of comparisons between groups and between bouts are shown on the top of the figure
Muscle ultrasound echo-intensity and thickness
As shown in Fig. 4, no significant changes in the variables were observed after either bout in either group in muscle thickness and echo intensity, and no group × time interactions were evident.
Ultrasound echo intensity (top) and muscle thickness (bottom) before (pre), immediately after (post), and 24–72 h after the first and second exercise bouts (mean ± SD) in the H–H (filled circle) and L–H (unfilled circle) groups. ANOVA results for interaction (bout × time) are shown in the legend for each bout. Results of comparisons between groups and between bouts are shown on the top of each figure
Discussion
This was the first study to compare between high- and low-intensity eccentric cycling of the same total mechanical work for changes in muscle function, muscle soreness and other indirect muscle damage markers after the initial exercise bout, and to examine the changes after a second bout of high-intensity eccentric cycling performed 14 days later. The primary finding was that changes in both muscle function and plasma CK activity were similar between the high- and low-intensity eccentric cycling bouts, which was consistent with the hypothesis that muscle damage evoked by eccentric exercise would not be related to exercise intensity when the exercise was performed submaximally. Nonetheless, muscle soreness was greater after high- than low-intensity cycling, indicating a different effect of exercise intensity on muscle soreness than other muscle damage markers. An additional and unexpected finding of the study was that changes in muscle function and plasma CK activity were similar between the first and second (repeated) bouts of ECC, although a decreased soreness response was observed in H–H whilst greater soreness was observed in the second (high-intensity) bout than the first (low-intensity) bout in L–H.
The present study used two groups to compare two intensities of eccentric cycling, with the average intensities being 154 W for the high-intensity and 36 W for the low-intensity groups. However, while there was a fourfold difference in intensity, the total work was similar between the groups, as planned. The average heart rate was approximately 20 bpm higher during the high- than low-intensity eccentric cycling, and subjective effort was approximately two points greater during high- than low-intensity cycling. Thus, given that eccentric exercise intensity in the current cohort could easily be greater than 500 W if performed maximally (Lipski et al. 2018), even the “high-intensity” eccentric cycling group performed the exercise at a relatively low intensity.
There were no differences observed in muscle function after high- versus low-intensity eccentric cycling (Fig. 1), which was consistent with findings of previous studies comparing work-matched submaximal high- and low-intensity eccentric elbow flexor exercise (Gauche et al. 2009; Hill et al. 2019). No previous study has compared the muscle damaging effects between different submaximal intensities of eccentric exercise. However, Paschalis et al. (2005) compared maximal and 50% of maximal eccentric knee extensor exercise matched for mechanical work, and reported a decrease in MVIC torque of 14% in the maximal exercise condition only. Paulsen et al. (2010) and Raastad et al. (2010) reported a 47% reduction in maximal concentric knee extensor torque after 300 (30 sets of 10) maximal eccentric (isokinetic) knee extensions, which was still 13% below baseline at 7 days post-exercise. It is interesting to note that the number of eccentric actions in the high-intensity cycling in the present study was also 300, but the magnitude of muscle strength decrease was much smaller (< 15%), and most muscle strength measures returned to baseline within 3 days post-exercise. Although the exercise mode was very different, this suggests that the intensity of eccentric actions is a factor influencing the magnitude of muscle function decrement when maximal exercise is performed.
The magnitude of the decreases in all muscle function measures (Fig. 1) and changes in plasma CK activity (Fig. 3) were similar between the high- and low-intensity eccentric cycling, although the intensity differed by fourfold. These findings suggest that exercise volume (i.e. number of eccentric actions) is a more important factor affecting the magnitude of decrease in muscle function than exercise intensity when the intensity is submaximal. This has been shown in the previous studies (Gauche et al. 2009; Hill et al. 2019) in which work-matched high- and low-intensity eccentric exercise of the elbow flexors were compared. Taken together, the results of the present study supported the hypothesis that there would be no difference in the magnitude of decrease in muscle function between higher- and lower-intensity submaximal eccentric exercise bouts when the eccentric action intensity was submaximal and the total mechanical work was matched.
It is of interest that more muscle soreness developed after the high- than low-intensity eccentric cycling, and peak muscle soreness in H–H (4.2 ± 1.0 out of 10) was three times that of the L–H (1.4 ± 0.6). These results contradict the findings of other studies comparing non-work-matched maximal and low-intensity eccentric elbow flexor exercise (Nosaka and Newton 2002) or work-matched maximal and lower-intensity eccentric knee extensor exercise (Paschalis et al. 2005), which did not show significant differences between the two intensities for DOMS. However, the results of the present study are consistent with previous studies examining non-work-matched submaximal eccentric elbow flexor exercise which reported that higher intensities evoked greater DOMS (Chen et al. 2007, 2012). Although DOMS does not appear to be severe after eccentric cycling, DOMS could be an issue for the prescription of the eccentric exercise. Moreover, DOMS was greater after high- than low-intensity eccentric cycling in the second bout (Fig. 2). It appears that the magnitude of DOMS is more closely associated with exercise intensity than the mechanical work, even when exercise mechanical work is matched. These results also highlight that the level of DOMS is not necessarily associated with the level of functional loss or other indirect markers of muscle damage (e.g., CK activity in the blood), as shown previously (Nosaka et al. 2002; Paschalis et al. 2005).
It has been documented that DOMS is more associated with damage and inflammation of the muscular connective tissue (i.e. endomysium, perimysium and epimysium) than the muscle fibres (Crameri et al. 2007; Lau et al. 2015; Sorensen et al. 2018). Moreover, muscle fibre strain, rather than force, appears to be the main determinant of the magnitude of histological damage following eccentric exercise (Lieber and Fridén 1993; Warren et al. 1993). Higher-intensity eccentric actions could impose greater mechanical strain on muscle fibres, and thus connective tissue disruption may be increased. Paulsen et al. (2010) reported the accumulation of inflammatory cells in the endomysium after 300 maximal eccentric knee extensions that induced only a small magnitude of muscle fibre damage (less than 1.4% of whole muscle fibres were infiltrated by the inflammatory cells). It seems possible that the intensity of eccentric actions is a mediator of the magnitude of muscle fibre strain, which could be the primary contributor to the development of DOMS due to connective tissue disruption. To explicitly test this hypothesis, future studies are needed to examine the relationship between DOMS, muscle fibre strain and connective tissue damage induced by eccentric actions.
Previous studies have reported recovery of MVIC torque to baseline within 1 day post-exercise, a peak muscle soreness reduction of 84%, and no significant increase in plasma CK activity after the second bout of eccentric cycling performed at 60% PPO than after the first bout that was performed 2 weeks before (Peñailillo et al. 2013). In that study, the strength loss lasted for 3 days, muscle soreness peaked at 25 mm in a 100-mm visual analogue scale, and peak plasma CK activity was 246.5 IU/L, while in the present study changes in many of the muscle function measures were not statistically different between bouts. Also, although changes in MVCC90, SJ, CMJ and muscle soreness were significantly smaller after the second than the first bout in H–H, the magnitude of the differences between bouts observed were not as large as those reported by Peñailillo et al. (2013). Moreover, no changes in muscle function, except CMJ (Fig. 1), were observed in L–H between the first and second bouts. Cumulatively, indicators of muscle damage and muscle soreness in the second bout of exercise in the present study were less reduced than those in other studies, suggesting that the lower-intensities of exercise used in the present study induced a smaller RBE.
Previous studies have concluded that a greater RBE is produced when muscle damage in the initial bout is greater (Chen et al. 2007, 2012). As discussed above, changes in muscle damage markers after the first eccentric cycling bout were small in the present study. It seems possible that the relatively small RBE observed in the present study was due to the relatively minor muscle damage produced by the first bout. There may be a lack of the repeated bout effect when submaximal eccentric exercise causes only a small amount of muscle damage after the first bout (Falvo et al. 2009). Therefore, a ‘ceiling effect’ might explain the relative lack of RBE in the present study.
While the muscle soreness was greater in the second than the first bout in L–H, it was still significantly smaller than the muscle soreness in the first bout of H–H (Fig. 2). This indicates that a moderate RBE for muscle soreness was induced by performing low-intensity eccentric exercise, which is consistent with previous reports of lower-intensity eccentric exercise conferring a protective effect on higher-intensity eccentric exercise (Chen et al. 2012, 2013). As discussed above, DOMS being more associated with connective tissue damage and inflammation, and it being induced more in high- than low-intensity eccentric cycling, probably explains the greater muscle soreness after the second than the first bout in L–H. Several mechanisms of the RBE have been proposed, with extracellular matrix remodelling being considered a likely player (Hyldahl et al. 2017). It would be interesting to investigate further whether the mechanisms underpinning the RBE differ for muscle soreness than for other symptoms of muscle damage (e.g., loss of muscle function), and then to compare changes in connective tissue damage markers between bouts.
Relative joint power contributions during the eccentric cycling were not assessed in the present study. It is, therefore, not known whether muscles crossing other joints may have performed significant eccentric work, and that this might have been different between groups. However, given that intensities were submaximal in both conditions, we assumed that the joint contributions would not differ significantly. Future studies should control for joint contributions to ensure that they do not differ significantly between intensities. Moreover, in the present study, we assessed muscle damage through indirect markers only. It would be of interest to examine histological responses in the muscle fibres and extracellular matrix that could potentially provide a clearer understanding of the effects of different intensities of eccentric exercise on the RBE.
The current findings have a number of practical implications. First, the magnitude of muscle damage was minor after eccentric cycling, but even low-intensity eccentric cycling could result in prolonged decreases in muscle function. Previous studies reported increases in muscle function and muscle volume after eccentric cycling training (LaStayo et al. 2000). Thus, eccentric cycling may be suitable for exercise prescription for all populations including older adults and frail individuals, but potential muscle damage induced by eccentric cycling should be cognizant. Second, DOMS was more significant after the high-intensity exercise, so individuals may “feel” greater pain in the days after exercise if higher initial intensity exercises are performed. Given this finding, it should be noted that muscle pain in the days after exercise may not provide a good indication of the level of muscle damage. Third, regardless of whether the bout was preceded by a high- or low-intensity initial bout, and despite there being no detectible differences in muscle damage markers between conditions, less DOMS was evoked by a subsequent high-intensity bout when the initial bout was performed at a high intensity. Thus, a single bout of lower-intensity eccentric cycling may not be sufficient to minimise DOMS after subsequent bouts. We recommend that intensity should be increased over several sessions in populations who require protection from soreness after training; this is an important consideration for exercise adherence (LaStayo et al. 2014).
In conclusion, muscle damage was not severe even after an unaccustomed bout of high-intensity eccentric cycling, and was not different between high- and low-intensity bouts when the exercise was submaximal and mechanical work-matched. Thus, mechanical work, rather than intensity, is probably a more significant factor influencing muscle damage under these conditions. Nonetheless, muscle soreness was greater after high- than low-intensity cycling; under these conditions DOMS does not seem to be a predictor of muscle damage. DOMS was greater when low-intensity rather than high-intensity exercise was performed in the initial bout. So the repeated bout effect for DOMS was intensity-dependent during the submaximal cycling exercise even though exercise mechanical work was matched.
Abbreviations
- CK:
-
Creatine kinase
- CMJ:
-
Counter-movement jump
- DOMS:
-
Delayed-onset muscle soreness
- H–H:
-
High–high group
- L–H:
-
Low–high group
- MVCC:
-
Maximal voluntary concentric contraction
- MVIC:
-
Maximal voluntary isometric contraction
- PPO:
-
Peak power output
- RBE:
-
Repeated bout effect
- RFD:
-
Rate of force development
- SJ:
-
Squat jump
References
Armstrong RB (1990) Initial events in exercise-induced muscular injury. Med Sci Sports Exerc 22:429–435. https://doi.org/10.1249/00005768-199008000-00002
Borg G (1998) Borg’s perceived exertion and pain scales. Human Kinetics, Champaign
Chen TC, Nosaka K, Sacco P (2007) Intensity of eccentric exercise, shift of optimum angle, and the magnitude of repeated-bout effect. J Appl Physiol 102:992–999. https://doi.org/10.1152/japplphysiol.00425.2006
Chen TC, Tseng W-C, Huang G-L et al (2013) Low-intensity eccentric contractions attenuate muscle damage induced by subsequent maximal eccentric exercise of the knee extensors in the elderly. Eur J Appl Physiol 113:1005–1015. https://doi.org/10.1007/s00421-012-2517-3
Chen TC-C, Chen H-L, Pearce AJ, Nosaka K (2012) Attenuation of eccentric exercise-induced muscle damage by preconditioning exercises. Med Sci Sports Exerc 44:2090–2098. https://doi.org/10.1249/MSS.0b013e31825f69f3
Clarkson PM, Nosaka K, Braun B (1992) Muscle function after exercise-induced muscle damage and rapid adaptation. Med Sci Sports Exerc 24:512–520. https://doi.org/10.1249/00005768-199205000-00004
Crameri RM, Aagaard P, Qvortrup K et al (2007) Myofibre damage in human skeletal muscle: effects of electrical stimulation versus voluntary contraction. J Physiol 583:365–380. https://doi.org/10.1113/jphysiol.2007.128827
Falvo MJ, Schilling BK, Bloomer RJ, Smith WA (2009) Repeated bout effect is absent in resistance trained men: An electromyographic analysis. J Electromyogr Kinesiol 19:e529–e535. https://doi.org/10.1016/j.jelekin.2008.10.010
Fridén J, Sjöström M, Ekblom B (1983) Myofibrillar damage following intense eccentric exercise in man. Int J Sports Med 4:170–176. https://doi.org/10.1055/s-2008-1026030
Gauche E, Couturier A, Lepers R et al (2009) Neuromuscular fatigue following high versus low-intensity eccentric exercise of biceps brachii muscle. J Electromyogr Kinesiol 19:e481–e486. https://doi.org/10.1016/j.jelekin.2009.01.006
González-Bartholin R, Mackay K, Valladares D et al (2019) Changes in oxidative stress, inflammation and muscle damage markers following eccentric versus concentric cycling in older adults. Eur J Appl Physiol 119:2301–2312. https://doi.org/10.1007/s00421-019-04213-7
Hasenoehrl T, Wessner B, Tschan H et al (2017) Eccentric resistance training intensity may affect the severity of exercise induced muscle damage. J Sports Med Phys Fitness 57:1195–1204. https://doi.org/10.23736/S0022-4707.16.06476-8
Hill EC, Housh TJ, Smith CM et al (2019) High vs low intensity fatiguing eccentric exercise on muscle thickness, strength, and blood flow. J Strength Cond Res. https://doi.org/10.1519/JSC.0000000000002632
Hortobágyi T, Houmard J, Fraser D et al (1998) Normal forces and myofibrillar disruption after repeated eccentric exercise. J Appl Physiol 84:492–498. https://doi.org/10.1152/jappl.1998.84.2.492
Hyldahl RD, Chen TC, Nosaka K (2017) Mechanisms and mediators of the skeletal muscle repeated bout effect. Exerc Sport Sci Rev 45:24–33. https://doi.org/10.1249/JES.0000000000000095
LaStayo P, Marcus R, Dibble L et al (2014) Eccentric exercise in rehabilitation: safety, feasibility, and application. J Appl Physiol 116:1426–1434. https://doi.org/10.1152/japplphysiol.00008.2013
LaStayo PC, Pierotti DJ, Pifer J et al (2000) Eccentric ergometry: increases in locomotor muscle size and strength at low training intensities. Am J Physiol Regul Integr Comp Physiol 278:R1282–1288. https://doi.org/10.1152/ajpregu.2000.278.5.R1282
Lau WY, Blazevich AJ, Newton MJ et al (2015) Changes in electrical pain threshold of fascia and muscle after initial and secondary bouts of elbow flexor eccentric exercise. Eur J Appl Physiol 115:959–968. https://doi.org/10.1007/s00421-014-3077-5
Lieber RL, Fridén J (1993) Muscle damage is not a function of muscle force but active muscle strain. J Appl Physiol 74:520–526. https://doi.org/10.1152/jappl.1993.74.2.520
Lipski M, Abbiss CR, Nosaka K (2018) Cardio-pulmonary responses to incremental eccentric and concentric cycling tests to task failure. Eur J Appl Physiol 118:947–957. https://doi.org/10.1007/s00421-018-3826-y
Maffiuletti NA, Aagaard P, Blazevich AJ et al (2016) Rate of force development: physiological and methodological considerations. Eur J Appl Physiol 116:1091–1116. https://doi.org/10.1007/s00421-016-3346-6
McHugh MP (2003) Recent advances in the understanding of the repeated bout effect: the protective effect against muscle damage from a single bout of eccentric exercise. Scand J Med Sci Sports 13:88–97. https://doi.org/10.1034/j.1600-0838.2003.02477.x
Nosaka K, Clarkson PM (1995) Muscle damage following repeated bouts of high force eccentric exercise. Med Sci Sports Exerc 27:1263–1269. https://doi.org/10.1249/00005768-199509000-00005
Nosaka K, Newton M (2002) Difference in the magnitude of muscle damage between maximal and submaximal eccentric loading. J Strength Cond Res 16:202–208. https://doi.org/10.1519/00124278-200205000-00006
Nosaka K, Newton M, Sacco P (2002) Delayed-onset muscle soreness does not reflect the magnitude of eccentric exercise-induced muscle damage. Scand J Med Sci Sports 12:337–346. https://doi.org/10.1034/j.1600-0838.2002.10178.x
Paschalis V, Koutedakis Y, Jamurtas AZ et al (2005) Equal volumes of high and low intensity of eccentric exercise in relation to muscle damage and performance. J Strength Cond Res 19:184–188. https://doi.org/10.1519/R-14763.1
Paulsen G, Crameri R, Benestad HB et al (2010) Time course of leukocyte accumulation in human muscle after eccentric exercise. Med Sci Sports Exerc 42:75–85. https://doi.org/10.1249/MSS.0b013e3181ac7adb
Peñailillo L, Blazevich A, Numazawa H, Nosaka K (2013) Metabolic and muscle damage profiles of concentric versus repeated eccentric cycling. Med Sci Sports Exerc 45:1773–1781. https://doi.org/10.1249/MSS.0b013e31828f8a73
Peñailillo L, Blazevich A, Numazawa H, Nosaka K (2015a) Rate of force development as a measure of muscle damage. Scand J Med Sci Sports 25:417–427. https://doi.org/10.1111/sms.12241
Peñailillo L, Blazevich AJ, Nosaka K (2015b) Muscle fascicle behavior during eccentric cycling and its relation to muscle soreness. Med Sci Sports Exerc 47:708–717. https://doi.org/10.1249/MSS.0000000000000473
Peñailillo L, Mackay K, Abbiss CR (2018) Rating of perceived exertion during concentric and eccentric cycling: are we measuring effort or exertion? Int J Sports Physiol Perform 13:517–523. https://doi.org/10.1123/ijspp.2017-0171
Raastad T, Owe SG, Paulsen G et al (2010) Changes in calpain activity, muscle structure, and function after eccentric exercise. Med Sci Sports Exerc 42:86–95. https://doi.org/10.1249/MSS.0b013e3181ac7afa
Sorensen JR, Skousen C, Holland A et al (2018) Acute extracellular matrix, inflammatory and MAPK response to lengthening contractions in elderly human skeletal muscle. Exp Gerontol 106:28–38. https://doi.org/10.1016/j.exger.2018.02.013
Tsuchiya Y, Ueda H, Ochi E (2019) Muscular recruitment is associated with muscular function and swelling following eccentric contractions of human elbow flexors. J Sports Med Phys Fitness 59:1097–1101. https://doi.org/10.23736/s0022-4707.18.09102-8
Valladares-Ide D, Peñailillo L, Collao N et al (2019) Activation of protein synthesis, regeneration and MAPK signaling pathways following repeated bouts of eccentric cycling. Am J Physiol Endocrinol Metab. https://doi.org/10.1152/ajpendo.00216.2019
Warren GL, Hayes DA, Lowe DA, Armstrong RB (1993) Mechanical factors in the initiation of eccentric contraction-induced injury in rat soleus muscle. J Physiol 464:457–475. https://doi.org/10.1113/jphysiol.1993.sp019645
Wobbrock JO, Findlater L, Gergle D, Higgins JJ (2011) The aligned rank transform for nonparametric factorial analyses using only ANOVA procedures. In: CHI 2011-29th Annual CHI Conference on Human Factors in Computing Systems, Conference Proceedings and Extended Abstracts. Vancouver, BC, Canada, pp 143–146
Yu J-G, Malm C, Thornell L-E (2002) Eccentric contractions leading to DOMS do not cause loss of desmin nor fibre necrosis in human muscle. Histochem Cell Biol 118:29–34. https://doi.org/10.1007/s00418-002-0423-1
Funding
No funding was received for this work.
Author information
Authors and Affiliations
Contributions
GM, AB and KN conceived and designed the study, and GM conducted the experiments. GM, OB, AF, WP, and TK collected and analysed the data. GM drafted the manuscript with AB and KN. All authors read, edited and approved the manuscript.
Corresponding author
Ethics declarations
Conflict of interest
The authors declare no conflict of interest. All participants signed an informed consent prior to partaking in the study.
Additional information
Communicated by Nicolas Place.
Publisher's Note
Springer Nature remains neutral with regard to jurisdictional claims in published maps and institutional affiliations.
Rights and permissions
About this article
Cite this article
Mavropalias, G., Koeda, T., Barley, O.R. et al. Comparison between high- and low-intensity eccentric cycling of equal mechanical work for muscle damage and the repeated bout effect. Eur J Appl Physiol 120, 1015–1025 (2020). https://doi.org/10.1007/s00421-020-04341-5
Received:
Accepted:
Published:
Issue Date:
DOI: https://doi.org/10.1007/s00421-020-04341-5