Abstract
Many microorganisms could naturally produce (R, R)-2,3-butanediol ((R, R)-2,3-BD), which has unique applications due to its special chiral group and spatial configuration. But the low enantio-purity of the product hindered the development of large-scale production. In this work, a synthetic constitutive metabolic pathway for enantiomerically pure (R, R)-2,3-BD biosynthesis was constructed in Escherichia coli with vector pUC6S, which does not contain any lac sequences. The expression of this artificial constructed gene cluster was optimized by using two different strength of promoters (AlperPLTet01 (P01) and AlperBB (PBB)). The strength of P01 is twice stronger than PBB. The fermentation results suggested that the yield of (R, R)-2,3-BD was higher when using the stronger promoter. Compared with the wild type, the recombinant strain E. coli YJ2 produced a small amount of acetic acid and showed higher glucose consumption rate and higher cell density, which indicated a protection against acetic acid inhibition. In order to further increase the (R, R)-2,3-BD production by reducing the accumulation of its precursor acetoin, the synthetic operon was reconstructed by adding the strong promoter P01 in front of the gene ydjL coding for the enzyme of (R, R)-2,3-BD dehydrogenase which catalyzes the conversion of acetoin to (R, R)-2,3-BD. The engineered strain E. coli YJ3 showed a 20 % decrease in acetoin production compared with that of E. coli YJ2. After optimization the fermentation conditions, 30.5 g/L of (R, R)-2,3-BD and 3.2 g/L of acetoin were produced from 80 g/L of glucose within 18 h, with an enantio-purity over 99 %.
Similar content being viewed by others
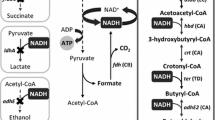
Avoid common mistakes on your manuscript.
Introduction
2,3-Butanediol (2,3-BD) is a valuable compound which has great potential applications in foods, cosmetics, pharmaceutical, fine chemical, and agrochemical industries (Celinska and Grajek 2009; Ji et al. 2011; Zeng and Sabra 2011). Due to its two chiral centers, the molecule of 2,3-BD exists in three stereoisomeric forms: (R, R)-, (S, S)-, and meso-forms (Ji et al. 2011). Among the three stereoisomers, the optical active (R, R)-2,3-BD has special application in asymmetric synthesis of valuable chiral specialty chemicals. For instance, (R, R)-2,3-BD can be used as the chiral directing group in the synthesis of (αS)-α-chloro boronic esters, which can then be easily hydrolyzed to crystalline boronic acids (Sadhu et al. 1984). Additionally, (R, R)-2,3-BD has a freezing point as low as −60 °C, making it promising for commercial use as an antifreeze (Celinska and Grajek 2009).
Many native microorganisms, such as Klebsiella pneumoniae, Klebsiella oxytoca, Enterobacter cloacae, and Serratia marcescens (Celinska and Grajek 2009; Ji et al. 2011; Kim et al. 2013; Xu et al. 2014), can ferment carbon source to 2,3-BD by homologous pathways involving three enzymes: α-acetolactate synthase (ALS), acetolactate decarboxylase (ALDC), and 2,3-BD dehydrogenase (2,3-BDH). Unfortunately, most of them produce a mixture of stereoisomers and none of the isomers can reach an enantio-purity over 99 %. Beyond that, the strains mentioned above belong to class 2 microorganisms, which make them unsuitable for industrial scale fermentation (Ji et al. 2011; Kim et al. 2013). Therefore, development of an industrially friendly and nonpathogenic host for the production of (R, R)-2,3-BD is highly desirable.
Recombinant Escherichia coli possesses strong potentials for 2,3-BD production due to its advantages including the ability to utilize several inexpensive carbon sources, the ease for genetic manipulations, and the well-studied metabolic pathways (Li et al. 2010). Moreover, the enantiomerically pure 2,3-BD isomers could be obtained by expressing the stereospecific 2,3-BDHs (Chen et al. 2014; Ui et al. 1986). Previously, scientists have successfully obtained enantiomerically pure (R, R)- and meso-forms of 2,3-BD by engineered E. coli (Ui et al. 1997; Ui et al. 2004; Yan et al. 2009).
In our previous reports, we constructed a synthetic metabolic pathway regulated by an inducible trc promoter for enantiomerically pure (R, R)-2,3-BD biosynthesis in E. coli MG1655 (Ji et al. 2015). All of the induced cultures got a considerable expression of the three target proteins, while the uninduced culture did not show obvious expression. However, once IPTG was added, even at a low level, the concentration and yield of (R, R)-2,3-BD were terribly affected. The phenomenon showed that the expression level even with the background expression was enough for the optimal (R, R)-2,3-BD production. Strong overexpression of target genes can cause growth inhibition (Lu and Jeffries 2007). Although inducible promoters allow for a continuous control of expression at the macroscopic level, practical applications of these systems are limited by hypersensitivity to inducer concentration, high inducer costs, and transcriptional heterogeneity at the single-cell level (Alper et al. 2005; Ji et al. 2015). Alternative approach is to create libraries of constitutive promoters with various strengths that allow tuning expression to particular levels (Lu and Jeffries 2007). In the present study, we had chosen two constitutive promoters, promoter AlperPLTet01 (P01) and promoter AlperBB (PBB), from Alper’s promoter library (Alper et al. 2005). The strength of promoter P01 is twice stronger than promoter PBB. The effects of using either strong (P01) or weak (PBB) promoters to drive the synthetic (R, R)-2,3-BD operon were compared. The results demonstrated that the yield of (R, R)-2,3-BD was higher when using the stronger promoter. Furthermore, the concentration of acetoin, which is the precursor of (R, R)-2,3-BD, was observed to be relatively high (up to 8.0 g/L). We therefore reconstructed the synthetic operon by adding the strong promoter P01 in front of the gene ydjL coding for the enzyme of (R, R)-2,3-BDH which catalyze the conversion of acetoin to (R, R)-2,3-BD. Finally, the titer of acetoin decreased by 20 %, and the yield of (R, R)-2,3-BD increased simultaneously. Using orthogonal design, the optimized fermentation conditions were developed. Under this fermentation conditions, 30.5 g/L of (R, R)-2,3-BD and 3.2 g/L of acetoin were produced from 80 g/L of glucose within 18 h, with an enantio-purity over 99 %.
Materials and methods
Strains, plasmids, and media
The bacterial strains and plasmids used in this study are listed in Table 1. The genes involved in the synthetic metabolic pathway were cloned from K. pneumoniae CICC 10011, and Bacillus subtilis 168. E. coli DH5α was used for general cloning procedures, while E. coli MG1655 was used for gene expression and as the host for (R, R)-2,3-BD production. The pUC6S vector was used for protein expression, which carries the ori and bla gene present on all pUC vectors, but does not contain any lac sequences (Vieira and Messing 1991).
Luria-Bertani (LB) medium was used for cultivation of E. coli, B. subtilis, and K. pneumoniae, with glucose supplement as the fermentation culture. Before fermentation, these media were autoclaved at 121 °C for 20 min, while glucose was separately autoclaved. Ampicillin was filtered with 0.22-μm filter and added to the medium when necessary.
Standard molecular techniques and plasmid construction
Plasmids and primers used in this study are listed in Table 1. Plasmid extraction, agarose gel electrophoresis, and other DNA manipulations were carried out by using standard protocols (Chong 2001; Lu and Jeffries 2007). The construction of budB, budA, and ydjL (GenBank accession number for budB, budA, and ydjL, respectively: KT724300, KT724301, KT724302) expression vectors under the control of P01 and PBB was generated via ClonExpress™ MultiS one-step cloning kit (Vazyme, China), which could assemble DNA molecules quickly relying on the use of overlaps. The mutants were generated as follows.
To reduce the background of undesired vector-only clones following assembly and transformation, pUC6S plasmid DNA was linearized by restriction digestion with HindIII and BamHI, and then extracted from an agarose gel following electrophoresis. The oligos that we used were designed. Adjacent oligos overlapped by 20 bp. The oligos at each end of the assembly contained a 26-bp overlap (with 6 bp of HindIII and BamHI sites, respectively) to the termini of vector pUC6S and restriction sites (HindIII and BamHI sites) not present in the assembled insert to allow the release of the synthesized product from pUC6S. The promoters P01 and PBB were synthesized by GENEWIZ (China) depending on the sequence published (Alper et al. 2005), and ligated into pUC57, generating pUC-P01, pUC-PBB. After being sequenced, the two plasmids were used as templates for PCR by using primers P1 to P4, generating P01# and PBB#. The primers P5 and P6 were used to amplify budB-budA-ydjL# from former constructed plasmid pTrc99a-budB-budA-ydjL (with ribosomal binding site (RBS) located upstream of each gene) (Ji et al. 2015). The budB-budA-ydjL#, vector pUC6S, promoter PCR fragment (P01# and PBB#, respectively) were mixed and assembled following the protocol (Vazyme, China). The electroporation, followed by a series of verification tests including plasmid extraction and restrictive digestion, was applied to transform the plasmids into E. coli MG1655. The finally confirmed recombinant strain harboring the plasmid pUC6S-P01-budB-budA-ydjL and pUC6S-PBB-budB-budA-ydjL were designated as YJ1 and YJ2, respectively.
The plasmid pUC6S-P01-budB-budA-P01-ydjL was constructed on the basis of pUC6S-P01-budB-budA-ydjL with the same method. The primers P7 and P8 were used to amplify P01-budB-budA#, primers P9 and P10 were used to amplify P01##, and primers P11 and P12 were used to amplify ydjL#. Adjacent oligos overlapped by 20 bp. The oligos at each end of the assembly contained a 26-bp overlap (with 6 bp of HindIII and BamHI sites, respectively) to the termini of vector pUC6S and restriction sites (HindIII and BamHI sites) not present in the assembled insert to allow the release of the synthesized product from pUC6S. pUC6S plasmid DNA was linearized by restriction digestion with HindIII and BamHI, then extracted from an agarose gel following electrophoresis. The generated plasmid pUC6S-P01-budB-budA-P01-ydjL then transformed to E. coli MG1655; the finally formed recombinant strain was designed as YJ3.
Seeds and fermentation procedure
For preparing seed inocula, strains were cultivated into 50-mL LB medium in 250-mL shake flasks for 24 h with agitation 200 rpm at 37 °C. For those strains carrying plasmids, ampicillin was added at a final concentration of 100 μg/mL.
For (R, R)-2,3-BD production in shake flask, the seed culture (10 %, v/v) was inoculated to the fermentation medium, with the initial glucose concentration of about 80 g/L. All the strains were cultured at 200 rpm, 37 °C. Fermentations continued until the glucose was exhausted.
For (R, R)-2,3-BD production in the 3-L fermenter, the seed culture (10 %, v/v) was inoculated to the fermenter with an operating volume of 2 L. The L9 (33) orthogonal table was employed to test the influence of main factors, including pH, agitation speed, and oxygen supply rate on (R, R)-2,3-BD fermentation. In all cases, the initial glucose concentration was about 80 g/L, and pH value was maintained at special value automatically by adding 6 M NaOH and 1.5 M H2SO4. Fermentation was ceased after 18 h.
Analytical methods
The biomass (OD600) was measured by a UV–visible spectrophotometer (Lambda-25, Perkin-Elmer, USA) with appropriate dilution. The concentration of residual glucose was determined by a biosensor equipped with the glucose oxidase electrode (SBA-40C, Institute of Biology, Shandong Academy of Sciences, China). The composition of fermentation broth ((R, R)-2,3-BD, meso-2,3-BD, acetoin, lactic acid, acetic acid, ethanol, and succinic acid) was determined by high-performance liquid chromatography (Summit P680 HPLC, Dionex, USA; Shodex RI-101 Refractive Index Detector, Showa Denko, Japan; Aminex HPX-87 H Ion Exclusion Column 300 mm × 7.8 mm, Bio-Rad, USA) upon the following conditions: sample volume 20 μL; mobile phase 5 mM H2SO4; flow rate 0.6 mL/min; column temperature 65 °C. For determination of different 2,3-BD stereoisomers, the previously described method of Yan et al. (2009) was used.
Enzyme activity assays
After the three strains were cultivated into the fermentation medium, samples at the lag (4 h) and stationary (12 h) phases of cell growth of each strain were obtained, then centrifuged at 12,000 rpm for 5 min, washed in 100 mM potassium phosphate buffer (pH 7.4), and suspended in the same buffer. Cells were disrupted with an ultrasonic cell breaking instrument. Cell debris was removed through centrifugation (12,000 rpm, 20 min). All operations were at 4 °C.
The ALS, ALDC, and 2,3-BDH assays were performed according to published procedures (Xu et al. 2014).
Real-time quantitative PCR
After the three strains were cultivated into the fermentation medium, samples at the lag (4 h) and stationary (12 h) phases of cell growth of each strain were obtained and RNA were isolated using the Rapid bacterial RNA extraction kit (Zoonbio Biotechnology, Nanjing, China). cDNA was obtained using the TUREscript cDNA Synthesis Kit (Zoonbio Biotechnology, Nanjing, China) and used for quantitative PCR analysis. Sybr Green Qpcr Mix (Zoonbio Biotechnology, Nanjing, China) and a LightCycler 3.0 system (Roche, American) were used for RT-qPCR experiment and for 2−△△Ct analysis. 16 s rRNA was used as reference. Primers used in the experiment are listed in Table 1.
Results
Construction of (R, R)-2,3-BD producers with different strength promoters
Three enzymes are required for (R, R)-2,3-BD production from pyruvate, including ALS, ALDC, and 2,3-BDH. In K. pneumoniae, the budB and budA genes encoding the ALS and ALDC, respectively, are responsible for acetoin production from pyruvate. In B. subtilis, ydjL gene encodes the (R, R)-specific 2,3-BDH and catalyzes (R, R)-2,3-BD formation from acetoin. To achieve effective (R, R)-2,3-BD production in E. coli, the genes mentioned above were cloned and assembled to produce enantiomerically pure (R, R)-2,3-BD. Under the designed gene assembly, the gene cluster was driven by two constitutively transcribed promoters, P01 and PBB (Alper et al. 2005). The promoter strength of P01 is twice stronger than PBB. The plasmid pUC6S (Vieira and Messing 1991), which carries the ori and bla gene present on all pUC vectors, but does not contain any lac sequences, was used as the vector in order to eliminate the influence of lactose operon. The plasmid pUC6S-P01-budB-budA-ydjL and pUC6S-PBB-budB-budA-ydjL were constructed as described in section “Standard molecular techniques and plasmid construction” and then electro-transformed into E. coli MG1655, generating the recombinant strain E. coli YJ1 and YJ2, respectively (Fig. 1).
Construction strategy used in this work. a PCR amplifies the budB, budA genes from Klebsiella pneumoniae CICC 10011, and the ydjL gene from Bacillus subtilis 168. b The promoter P01 and PBB are fused with target genes, with separated ribosomal binding sites (RBS) located upstream of each structure gene, and then inserted into the expression vector pUC6S. c Electro-transformed into E. coli MG1655
Fermentation characteristics of recombinant strains with different strength promoters
The recombinant strains, E. coli YJ1 and YJ2 were cultivated in LB medium supplemented with glucose for (R, R)-2,3-BD production, with the parent strain as control. Yields of (R, R)-2,3-BD, meso-2,3-BD and acetoin were determined in these E. coli strains. To better define the carbon flux, the levers of major by-products (acetic acid, lactic acid, succinic acid, and ethanol) were also determined. As shown in Fig. 2, whether under the control of promoter P01 or PBB, recombinant strains grew much faster than the parent strain. Additionally, the glucose consumption rate was quite different within the three cultures (Fig. 2). E. coli MG1655 showed difficulties in consuming glucose, with a total consumption of only about 12 g/L. Although E. coli YJ1, which was controlled by the weak promoter PBB, had a high rate of cell growth, with the highest OD600 reached up to 19.64, its glucose consumption rate was slow after the inoculation, resulting in a relatively poor (R, R)-2,3-BD concentration. Conversely, the strain E. coli YJ2, which controlled by the strong promoter P01, presented a faster glucose consumption and a significant formation of (R, R)-2,3-BD. In particular, E. coli YJ2 showed lower acetic acid production compared to E. coli YJ1. Carbon balance data also showed that E. coli YJ2 had the higher (R, R)-2,3-BD carbon ratio per glucose (Table 2).
Elimination of acetoin production
To improve the yield of (R, R)-2,3-BD, we added another strong promoter P01 in front of the ydjL gene to enhance its expression, generating the recombinant strain E. coli YJ3 (Fig. 1). As shown in Table 3 and Fig. 3, there were no significant differences between YJ2 and YJ3 in cell growth and glucose consumption. However, the amount of acetoin in strain YJ3 decreased by 20 %. Although the highest yield of (R, R)-2,3-BD did not increase remarkably, it did reduce the reversible reaction from (R, R)-2,3-BD to acetoin, for the concentration of (R, R)-2,3-BD in strain YJ2 and YJ3 was 13.4 g/L (30.9 g/L at 29 h) and 17.0 g/L (31.5 g/L at 29 h), respectively, after 41 h.
Enzyme activity and RT-qPCR assays
The activities of ALS, ALDC, and 2,3-BDH in the recombinant strains were determined (Fig. 4). Samples at the lag (4 h) and stationary (12 h) phases of cell growth of each strain were obtained. As shown in Fig. 4, all the recombinant strains showed ALS, ALDC, and 2,3-BDH activities. The variation of activities of these enzymes indicated that the combinatorial assembly of promoters could successfully modulate expression levels of genes within the (R, R)-2,3-BD operon. The specific enzyme activity of the budB, budA, and ydjL genes and the total protein of the YJ1, YJ2, and YJ3 strains at the lag and stationary phases of cell growth were obtained. As shown in Fig. 4, the total protein, the ALS and 2,3-BDH activities in strain YJ2 and YJ3 increased at the stationary phase. But the ALDC activities in these recombinant strains both reduced. Although the total protein, the ALDC, and 2,3-BDH activities in strain YJ1 showed the same trends in strain YJ2 and YJ3, the ALS activity in strain YJ2 at the stationary phase decreased compared to the lag phase. Strangely, although the (R, R)-2,3-BD operon in strain YJ1 were controlled by the weak promoter, the total protein, the ALS, ALDC, and 2,3-BDH activities were both higher than the other two strains, in which the operon were controlled by the strong promoter.
The transcription levels of the key genes (budB, budA, and ydjL) in (R, R)-2,3-BD production pathway in recombinant strains were investigated by RT-qPCR (Fig. 5). Samples at the lag (4 h) and stationary (12 h) phases of cell growth of each strain were obtained. Except the budB gene’s expression level in strain YJ1, the other two genes’ expression level in strain YJ1, YJ2, and YJ3 were both reduced at the stationary phase. The relative quantities of the budB and budA genes’ mRNA level in strain YJ1 were almost five times higher comparing to the strain YJ2 and YJ3, and the ydjL gene’s mRNA level in strain YJ1 was almost twice the level of the strain YJ2 and YJ3, during the stationary phase. This was the same as the enzymatic activity assays. The ydjL gene of the strain YJ3 showed increased expression level of the gene comparing to strain YJ2, which reflected the ydjL gene overexpression. The observed changes in gene transcription levels confirmed that overexpression of the ydjL gene in strain YJ3 resulted in the increase in carbon flux toward (R, R)-2,3-BD production.
Optimization of the fermentation conditions
E. coli YJ3 showed the best performance in (R, R)-2,3-BD production, and the use of constitutive promoter P01 made it convenient to operate and economically advantageous because no inducer was required. To improve the efficiency of (R, R)-2,3-BD production, fermentation conditions, including pH, agitation speed, and oxygen supply rate, were optimized using an orthogonal experimental design L9(33). Nine experiments were used to identify the best combination of three variables. The results of the orthogonal experimental design are shown in Table 4. These data showed that there was a wide range of (R, R)-2,3-BD concentration which varied from 8.8 to 30.5 g/L in the nine experiments. The results of range analysis are presented in Table 5. Results showed that range of factor B was 14.65, which ranked the first. Factor A was 3.84, which ranked second. Factor C was 3.21, which ranked the last. The bigger rank value of a factor represented greater effect on the final (R, R)-2,3-BD concentration. According to the range, the order of influence was agitation speed > pH > oxygen supply rate. The optimal condition for improving (R, R)-2,3-BD concentration was determined as A3B3C2. Thus, the optimal fermentation condition was determined as follows: pH 6.5, agitation speed 400 rpm, oxygen supply rate 1.5 vvm. At the optimized fermentation conditions, 30.5 g/L (R, R)-2,3-BD and 3.2 g/L acetoin were produced from 80 g/L of glucose within 18 h, with an enantio-purity over 99 %.
Discussion
As the native microbes generally have difficulties in producing (R, R)-2,3-BD at a high enantio-purity, heterologous expression of 2,3-BD operon with (R, R)-specific 2,3-BDH would be an efficient method to obtain enantiomerically pure 2,3-BD isomers. In this study, we have shown that the promoter strength plays a major role in (R, R)-2,3-BD production under stable conditions.
In order to achieve the balance of metabolic flux, two different kinds of plasmid had been constructed. In the plasmid pUC6S-P01-budB-budA-ydjL and plasmid pUC6S-PBB-budB-budA-ydjL, the synthetic metabolic pathway for (R, R)-2,3-BD biosynthesis was controlled by the constitutive promoter P01 and PBB, respectively. The two promoters were both picked from Alper’s promoter library and the strength of promoter P01 is twice stronger than promoter PBB. During the experiments, the parent strain E. coli MG1655 displayed a metabolic disorder, leading to difficulties in cell growth and glucose consumption, which were probably due to the accumulation of acetic acid (∼5.15 g/L). Under the same conditions, both recombinant strains obtained a much better cell growth and higher biomass density than E. coli MG1655. The production of acetic acid is related to the excessive carbon flux through the glycolytic pathway and the abundant acetic acid is harmful to cell growth (Sandoval et al. 2011). The introduction of (R, R)-2,3-BD pathway in E. coli will help to guide the excess pyruvate to the (R, R)-2,3-BD, which is less toxic, and finally release the inhibitory effect of acetic acid and promote the cell growth and glucose consumption (Xu et al. 2014). According to our speculation, the cell density is directly proportional to the yield of (R, R)-2,3-BD. However, an unexpectable phenomenon was observed when comparing the fermentation performance between the two recombinant strains. Strain YJ1 showed difficulty in consuming glucose and producing (R, R)-2,3-BD, but its cell density (19.64) was much higher than strain YJ2 (12.98). The enzymatic activity assay and the RT-qPCR results had showed that the expression level of all the three genes involved in (R, R)-2,3-BD production in strain YJ1 were higher than strain YJ2. We speculate that it is because of all the relevant genes in strain YJ1 were efficiently expressed, led to the carbon flux mainly satisfied the need of (R, R)-2,3-BD pathway, thus little acetic acid was produced and the cell growth was promoted. Previous studies showed that the basal level protein expression was sufficient to sustain flux for (R, R)-2,3-BD production and the overexpression of enzymes might cause protein burden, which could lead to a serious metabolic burden on the host (Ji et al. 2015; Snoep et al. 1995). For this reason, although the gene expression was especially high in strain YJ1, its glucose consumption and production were both limited.
Based on the results of the fermentation of these two recombinant strains, screening multiple promoters could improve the pathway function for (R, R)-2,3-BD production. The concentration of (R, R)-2,3-BD produced by strain YJ2, which was controlled by the strong promoter, was twice than that by the strain YJ1, which was controlled by the weak promoter. It illustrated that this artificial constructed (R, R)-2,3-BD pathway had its proper strength of promoter, we could choose a suitable promoter to further increase the production of (R, R)-2,3-BD.
Although the yield of (R, R)-2,3-BD in YJ1 and YJ2 were 0.5 and 0.8 mol/mol, respectively, the total yield of (R, R)-2,3-BD and acetoin was larger than 99 % of the theoretical maximum yield at the end of the fermentation (not shown). The high total yield of (R, R)-2,3-BD and acetoin indicated that promoting the conversion from acetoin to (R, R)-2,3-BD was the critical step for (R, R)-2,3-BD production, and the yield of (R, R)-2,3-BD could be increased by transforming a greater number of acetoin (Fu et al. 2014; Li et al. 2015). The major problem of using polycistronic vector is the much lower expression of the latter gene compared with that of the first gene next to the promoter. By simply adding a promoter sequence in front of the latter gene on a polycistronic vector, the mRNA transcripts of the latter gene can be generated independently of the “read-through” transcript from the first promoter (Kim et al. 2004). In order to enhance the conversion from acetoin to (R, R)-2,3-BD, the strain YJ3 was generated by adding the strong promoter P01 in front of the gene ydjL coding for the enzyme of (R, R)-2,3-BDH which catalyze the conversion of acetoin to (R, R)-2,3-BD. The enzymatic activity assay and RT-qPCR results both demonstrate an elevation in 2,3-BDH activity and ydjL expression level, which confirmed the viewpoint that the expression level of the latter gene in a polycistronic vector is much lower compared with that of the first gene next to the promoter (Kim et al. 2004). The two strains showed a similar rate of consumption of sugar and cell growth, but the concentration of acetoin in strain YJ3 reduced almost 20 % compared to the strain YJ2 (Table 3), which showed that overexpression ydjL gene did promote the transformation from acetoin to (R, R)-2,3-BD. The 2,3-BDH catalyze a reversible conversion between (R, R)-2,3-BD and acetoin (Dai et al. 2014), and the results illustrated that the overexpression of ydjL gene did reduce the reversible reaction from (R, R)-2,3-BD to acetoin, because the concentration of (R, R)-2,3-BD in strain YJ3 reduced much slower than in strain YJ2.
The recombinant strain E. coli YJ3 displayed a strong ability of consuming glucose and producing (R, R)-2,3-BD. After optimizing the fermentation conditions using an orthogonal experimental design L9(33), the nine experiments showed a wide range of variation in (R, R)-2,3-BD concentration. This variation indicated that the fermentation optimization was quite important in enhancing (R, R)-2,3-BD productivity. After the optimization, strain YJ3 produced 30.5 g/L (R, R)-2,3-BD and 3.2 g/L acetoin in the batch culture, with a yield of 0.4 g/g and a productivity of 1.7 g/(L·h), and the enantio-purity was larger than 99 %. This is another example showing the advantage of constructing a synthetic metabolic pathway by optimizing the constitutive promoter strength to fulfill the non-natural metabolite accumulation. The idea developed in this paper could be applied to the other similar industrial biotechnological processes to achieve high product concentration.
References
Alper H, Fischer C, Nevoigt E, Stephanopoulos G (2005) Tuning genetic control through promoter engineering. Proc Natl Acad Sci U S A 102:12678–12683
Celinska E, Grajek W (2009) Biotechnological production of 2,3-butanediol—current state and prospects. Biotechnol Adv 27:715–725
Chen C, Wei D, Shi JP, Wang M, Hao J (2014) Mechanism of 2,3-butanediol stereoisomer formation in Klebsiella pneumoniae. Appl Microbiol Biotechnol 98:4603–4613
Chong L (2001) Molecular cloning—a laboratory manual, 3rd edition. Science 292:446
Dai JJ, Cheng JS, Liang YQ, Jiang T, Yuan YJ (2014) Regulation of extracellular oxidoreduction potential enhanced (R, R)-2,3-butanediol production by Paenibacillus polymyxa CJX518. Bioresour Technol 167:433–440
Fu J, Wang Z, Chen T, Liu W, Shi T, Wang G, Y-j T, Zhao X (2014) NADH plays the vital role for chiral pure D-(−)-2,3-butanediol production in Bacillus subtilis under limited oxygen conditions. Biotechnol Bioeng 111:2126–2131
Ji X-J, Huang H, Ouyang P-K (2011) Microbial 2,3-butanediol production: a state-of-the-art review. Biotechnol Adv 29:351–364
Ji X-J, Liu L-G, Shen M-Q, Nie Z-K, Tong Y-J, Huang H (2015) Constructing a synthetic metabolic pathway in Escherichia coli to produce the enantiomerically pure (R, R)-2,3-butanediol. Biotechnol Bioeng 112:1056–1059
Kim KJ, Kim HE, Lee KH, Han W, Yi MJ, Jeong J, Oh BH (2004) Two-promoter vector is highly efficient for overproduction of protein complexes. Protein Sci 13:1698–1703
Kim D-K, Rathnasingh C, Song H, Lee HJ, Seung D, Chang YK (2013) Metabolic engineering of a novel Klebsiella oxytoca strain for enhanced 2,3-butanediol production. J Biosci Bioeng 116:186–192
Li Z-J, Jian J, Wei X-X, Shen X-W, Chen G-Q (2010) Microbial production of meso-2,3-butanediol by metabolically engineered Escherichia coli under low oxygen condition. Appl Microbiol Biotechnol 87:2001–2009
Li L, Li K, Wang Y, Chen C, Xu Y, Zhang L, Han B, Gao C, Tao F, Ma C, Xu P (2015) Metabolic engineering of Enterobacter cloacae for high-yield production of enantiopure (2R,3R)-2,3-butanediol from lignocellulose-derived sugars. Metab Eng 28:19–27
Lu C, Jeffries T (2007) Shuffling of promoters for multiple genes to optimize xylose fermentation in an engineered Saccharomyces cerevisiae strain. Appl Environ Microb 73:6072–6077
Sadhu KM, Matteson DS, Hurst GD, Kurosky JM (1984) (R,R)-2,3-Butanediol as chiral directing group in the synthesis of (Alpha-S)-Alpha-chloro boronic esters. Organometallics 3:804–806
Sandoval NR, Mills TY, Zhang M, Gill RT (2011) Elucidating acetate tolerance in E. coli using a genome-wide approach. Metab Eng 13:214–224
Snoep JL, Yomano LP, Westerhoff HV, Ingram LO (1995) Protein burden in Zymomonas mobilis: negative flux and growth control due to overproduction of glycolytic enzymes. Microbiology 141:2329–2337
Ui S, Masuda T, Masuda H, Muraki H (1986) Mechanism for the formation of 2,3-butanediol stereoisomers in Bacillus polymyxa. J Ferment Technol 64:481–486
Ui S, Okajima Y, Mimura A, Kanai H, Kudo T (1997) Molecular generation of an Escherichia coli strain producing only the meso-isomer of 2,3-butanediol. J Ferment Bioeng 84:185–189
Ui S, Takusagawa Y, Sato T, Ohtsuki T, Mimura A, Ohkuma M, Kudo T (2004) Production of L-2,3-butanediol by a new pathway constructed in Escherichia coli. Lett Appl Microbiol 39:533–537
Vieira J, Messing J (1991) New pUC-derived cloning vectors with different selectable markers and DNA replication origins. Gene 100:189–194
Xu Y, Chu H, Gao C, Tao F, Zhou Z, Li K, Li L, Ma C, Xu P (2014) Systematic metabolic engineering of Escherichia coli for high-yield production of fuel bio-chemical 2,3-butanediol. Metab Eng 23:22–33
Yan Y, Lee C-C, Liao JC (2009) Enantioselective synthesis of pure (R,R)-2,3-butanediol in Escherichia coli with stereospecific secondary alcohol dehydrogenases. Org Biomol Chem 7:3914–3917
Zeng A-P, Sabra W (2011) Microbial production of diols as platform chemicals: recent progresses. Curr Opin Biotechnol 22:749–757
Acknowledgments
This work was financially supported by the National Science Foundation for Distinguished Young Scholars of China (No. 21225626), the National Natural Science Foundation of China (Nos. 21376002, 21476111), the Jiangsu Provincial Natural Science Foundation of China (No. BK20131405), and the Priority Academic Program Development of Jiangsu Higher Education Institutions.
Conflict of interest
The authors declare that they have no competing interests.
Author information
Authors and Affiliations
Corresponding authors
Rights and permissions
About this article
Cite this article
Tong, YJ., Ji, XJ., Shen, MQ. et al. Constructing a synthetic constitutive metabolic pathway in Escherichia coli for (R, R)-2,3-butanediol production. Appl Microbiol Biotechnol 100, 637–647 (2016). https://doi.org/10.1007/s00253-015-7013-3
Received:
Revised:
Accepted:
Published:
Issue Date:
DOI: https://doi.org/10.1007/s00253-015-7013-3