Abstract
The efficiency of current methods for industrial production of the enzyme nuclease P1 is limited. In this study, we sought to improve fermentation methods for the production of nuclease P1. An immobilized fermentation system using an activated carbon filter sponge as a carrier was used for the production of nuclease P1. In an airlift internal loop reactor (ALR), the fermentation performance of three different fermentation modes, including free-cell fermentation, repeated-batch fermentation, and semi-continuous immobilized fermentation, were compared. The fermentation kinetics in the fermentation broth of the three fermentation modes, including dissolved oxygen (DO), pH value, cell concentration, residual sugar concentration, and enzyme activity, were tested. The productivity of semi-continuous immobilized fermentation reached 8.76 U/mL/h, which was 33.3 and 80.2 % higher than that of repeated-batch fermentation and free-cell fermentation, respectively. The sugar consumption of free-cell, repeated-batch, and semi-continuous immobilized fermentations was 41.2, 30.8, and 25.9 g/L, respectively. These results showed that immobilized-cell fermentation by using Penicillium citrinum with activated carbon filter sponge in an ALR was advantageous for nuclease P1 production, especially in the semi-continuous immobilized fermentation mode. In spite of the significant improvement in nuclease P1 production in semi-continuous immobilized fermentation mode, the specific activity of nuclease P1 was almost equal among the three fermentation modes.
Similar content being viewed by others
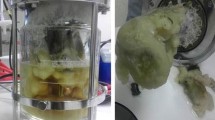
Explore related subjects
Discover the latest articles, news and stories from top researchers in related subjects.Avoid common mistakes on your manuscript.
Introduction
Nuclease P1 (IUBMB Enzyme Nomenclature: EC 3.1.30.1) is one of the most widely used single-strand-specific nucleases. The main function of this enzyme is to hydrolyze nucleic acids into four 5′-mononucleotides, for which it is widely used in the pharmaceutical and food industries (Kuninaka 2008; Gerald and Tilak 1990; Ying et al. 2006). The interest in nuclease P1 is growing because of the wide application of these 5′-nucleotides. Malt root extraction and Penicillium citrinum-based fermentation are used as the main production methods of nuclease P1 (He 2010). However, malt root extraction is disadvantageous for industrial production owing to the high content of impurities (Deoda and Singhal 2003). Nuclease P1 produced by P. citrinum shows high RNA hydrolytic efficiency. This is currently the main production mode of nuclease P1 because of the relatively simple fermentation conditions. Solid-state fermentation and submerged fermentation are also commonly used to produce nuclease P1. However, the disadvantages of these methods, such as high content of impurities, occupation of a large area, low productivity of solid-state fermentation, and the high cost of submerged fermentations, restrict their use for the production of nuclease P1 (Xia 1998; Wang and Wang 2003; Wang 1999).
Compared to free-cell fermentation, immobilized fermentation can effectively improve the performance of fermentation and lower the cost of production (Roberto et al. 1991). Fungal cells immobilized on a solid surface by physical methods, including physical adsorption and entrapment method, have been shown to have desirable characteristics for the production of secreted proteins (Talabardon and Yang 2005; Hui et al. 2010). These characteristics include prolonged enzyme production, higher productivity, and increased operational stability because of improved oxygen transfer and product secretion from the biofilm, which reduces viscosity and facilitates downstream processing (Lan et al. 2013). It is therefore conceivable that the use of immobilized organisms may provide a solution towards a reduction in the cost of producing nuclease P1. The interest in the use of immobilized cells for nuclease P1 production has grown over the last few years. Different mycelia immobilization strategies adopting various carriers such as lacunaris polyester, corncob powder, sodium alginate, polyvinyl alcohol, and polyurethane inert carrier have been used for nuclease P1 production (Xia 1998; Wang and Wang 2003; Song et al. 2008; Zhu et al. 1996). However, complex process control, poor performance on repeated use of the carrier, and complicated downstream processing have limited the utility of these materials for P. citrinum immobilization for nuclease P1 production. Therefore, the development of a more natural and efficient immobilized carrier for the production of nuclease P1 is needed. We identified an activated carbon filter sponge, possessing a large specific surface area, high porosity, and good adsorption properties, as an optimal material for immobilization of P. citrinum for high-yield production of nuclease P1.
In this study, three different P. citrinum fermentation modes, free-cell, repeated-batch, and semi-continuous immobilized fermentations, were used for nuclease P1 production. The fermentation performance of the three modes has been compared in our study by using the same bioreactor with activated carbon filter sponge as the immobilized carrier of P. citrinum. Activated carbon filter sponge immobilization was efficient for enhancing the stability and vitality of microorganism in the fermentation. The process of activated carbon filter sponge immobilization is simple, low cost, and environmentally benign, making it an attractive system for nuclease P1 production. However, its use in an immobilized culture for nuclease P1 production has not been studied before, necessitating systematic investigation and research.
Materials and methods
Microorganism and inoculum
Penicillium citrinum CGMCC NO. 2014, a strain producing high yields of nuclease P1, was mutated by low-energy ion beam implantation in our laboratory. The strain was maintained at 4 °C on malt agar medium. For the production of spores, the strain was cultured on malt agar slants at 30 °C for 6 days. Spores were then harvested, suspended in sterile distilled water, and adjusted to the desired concentration by counting in a Neubauer chamber to inoculate the fermentation cultures described below.
Carrier preparation
The activated carbon filter sponge used for the immobilization of P. citrinum was purchased from Shanghai Yan Ya Environmental Protection Technology Co. Ltd. It had a density of 680 kg m−3 and had 20 pores per inch with the average porosity of 93 %. Carbon content of the sponge was at least 45 %. After cutting into cubic pieces with a side length of 5 mm, the activated carbon filter sponge was alternately eluted with 1 M NaOH solution and 1 M HCl. The sponge was then washed with distilled water until the pH value reached 7 and was dried at 60 °C for 24 h. Finally, activated carbon filter sponge was restricted in the draft tube with stainless steel wire mesh, mixed with the medium at an appropriate dosage, and sterilized at 121 °C for 20 min.
Media and culture conditions
The culture was cultivated on malt agar medium at 30 °C for 6 days before it was inoculated into the fermentation medium. The fermentation medium composition was (per liter) the following: 50 g of glucose, 5 g of peptone, 0.5 g of KH2PO4, 0.5 g of K2HPO4·3H2O, 0.4 g of CaCl2, 0.4 g of ZnSO4·7H2O, 0.4 g of MgSO4, and 5 g of wheat bran (only in free-cell fermentation). The initial pH was adjusted to 6.5 with 5 M NaOH before the medium was sterilized. Fermentation was carried out at 30 °C in a 15-L airlift internal loop reactor (ALR) containing 12 L of the medium. The ALR was supplied by Huihetang Bioengineering Equipment Co. Ltd. (Shanghai, China), which had a diameter of 15 cm. All media were sterilized at 121 °C for 20 min. The fermentation solution containing nuclease P1 was centrifuged at 8000g at 4 °C for 10 min, and the supernatant was used as the nuclease P1 enzyme solution for further analysis.
Free-cell fermentation and immobilized fermentation
Free-cell fermentation was carried out in a 15-L ALR containing 12 L of the medium described above. After autoclaving at 121 °C for 20 min, the bioreactor was inoculated with 100 mL of a spore suspension (106/mL) and then operated at 30 °C with aeration at 2 vvm, and without any pH control. The dissolved oxygen level and pH of the medium were monitored by using the dissolved oxygen electrode and pH electrode, respectively. To prevent excessive foaming, antifoam was added as needed. Broth samples were taken at 6-h intervals after the first 12 h for immediate determination of A600nm. Samples were then centrifuged at 8000g at 4 °C for 10 min, and the supernatants were used for determination of nuclease P1 activity, residual sugar quantification, and protein concentration determination. Duplicate experiments for each sample were conducted.
Repeated-batch fermentation experiments were also carried out in a 15-L ALR containing 24 g of the sterilized immobilization material, which was restricted in the draft tube with stainless steel wire mesh, and 12 L of culture medium as previously described. The conditions of fermentation were the same as those used for free-cell fermentation. During the first cycle, the cells were immobilized on activated carbon filter sponge by natural adsorption. At the end of the first batch, the fermented broth removed from the bioreactor, leaving the carrier with immobilized mycelia for the second batch. After adding 12 L of fresh culture medium, the second batch was initiated under the conditions described above.
To further shorten the fermentation period and increase the enzyme productivity, semi-continuous fermentation by P. citrinum immobilized on the activated carbon filter sponge was conducted in an ALR. At the end of each batch, approximately 90 % of the fermented broth removed from the bioreactor, leaving 10 % of the broth and the carrier with immobilized mycelia for the next batch. Other conditions were same as in repeated-batch fermentation.
Analytical methods
Assay of nuclease P1 activity
Nuclease P1 activity was measured using the methods of He et al. (2011). Activity was assayed in terms of the amount of acid-soluble nucleotides produced by RNA hydrolysis, which is catalyzed by nuclease P1 (Fujishima et al. 1972; Fujimoto et al. 1974). The enzyme solution (0.1 mL) was incubated with substrate solution (1 mL of 5 % RNA (w/v), 0.9 mL of 0.2 M acetate buffer of pH 5.0, and 3 mM Zn2+) at 70 °C for 15 min. The reaction was stopped by adding 2.0 mL of ice-cold nucleic acid precipitator (0.25 % ammonium molybdate dissolved in 2.5 % perchloric acid (w/v)). The mixture was incubated in an ice bath for 10 min. The precipitated RNA was removed by centrifugation at 10,000g at 4 °C for 10 min. The supernatant was diluted 250-fold with distilled water. The absorbance of the diluted solution at 260 nm was read against a blank solution without enzymes. The activity (U/mL) of the obtained nuclease P1 was calculated according to the following formula:
where α is the dilution factor of the enzyme before the assay. One unit of enzyme activity is defined as the amount of enzyme that produces an increase of 1.0 in the optical density at 260 nm in 1 min.
The protein concentration was measured by the method described by Bradford (1976) using Coomassie Brilliant Blue G-250. The specific activity of nuclease P1 (U/mg) was calculated as the ratio of nuclease P1 activity to the protein concentration.
Assay of residual sugar
The residual sugar was determined by the commonly used 3,5-dinitrosalicylic acid method.
SDS-PAGE analysis of the enzyme solution
Electrophoresis was performed as described by Laemmli (1970). Samples were mixed 1:3 (v/v) with the 4× sodium dodecyl sulfate polyacrylamide gel electrophoresis (SDS-PAGE) loading buffer and loaded on a 12 % stacking gel and separating gel. Electrophoresis was run at a voltage of 80 V for 30 min and then at a voltage of 120 V for 2 h. Protein bands in the gel were visualized by Coomassie Brilliant Blue R-250 staining. The molecular mass of test proteins was compared with standard protein markers (myosin at 200 kDa, β-galactosidase at 116 kDa, phosphatase B at 97.2 kDa, bovine serum albumin at 66.4 kDa, ovalbumin at 44.3 kDa, carbonic anhydrase at 29 kDa, trypsin inhibitor at 20.1 kDa, lysozyme at 14.3 kDa, and aprotinin at 6.5 kDa), which were purchased from TaKaRa Biotech, China.
Analysis of the characteristics of the surface of the carrier
Micrographs were obtained using scanning electron microscopy (SEM) of activated carbon filter sponge with immobilized P. citrinum. Before examination, specimens were dried using a Labconco FreeZone vacuum freezing-drying device (Labconco Corporation, Fort Scott, Kansas, US), sputter-coated with gold, and examined using a scanning electron microscope (TM3000, Hitachi, Japan). Each sample was observed under magnifications of ×100, ×500, and ×1000.
Results
Free-cell fermentation in an ALR
Batch fermentation with free mycelia was carried out in an ALR for 86 h. Figure 1 shows the kinetics including dissolved oxygen (DO), pH value, cell concentration (optical density (OD)600nm), residual sugar concentrations, and enzyme activity in the fermentation broth. The DO decreased rapidly to 20 % within the first 42 h and then gradually went up to 29 % with rare change in biomass. The pH values during fermentation showed a decreasing trend and were not controlled throughout the fermentation. The pH values ultimately dropped to 4.12 and remained unchanged. Throughout the fermentation, the concentration of residual sugars declined to 8.8 g/L at the lowest, while the biomass increased throughout the fermentation. Enzyme activity reached the highest point of 418 U/mL at 86 h at the end of fermentation. The productivity of free-cell fermentation reached 4.86 U/mL/h. Biomass growth was accompanied by the consumption of glucose, and the secretion of nuclease P1 was associated with cell growth. However, the rate of increase in enzyme activity was slower than that of the biomass growth. While a maximum biomass was achieved, enzyme activity continued to increase. During fermentation, the broth was turbid and ropy because of the suspended wheat bran particles and mycelia, which led to the diffuse constraint of oxygen and substrate movements.
Repeated-batch fermentation in an ALR
Activated carbon filter sponge was selected as the immobilized carrier for nuclease P1 production. Figure 2 shows the kinetics of immobilized-cell fermentation in the ALR running for six cycles. The fermentation showed similar trends in DO, pH, cell concentration (OD600nm), residual sugar concentrations, and nuclease P1 production to those in free-cell fermentation. However, more residual sugar (averaging 19.2 g/L between six batches) and higher dissolved oxygen (approximately 54 %) were attained at the end of immobilized-cell fermentation. It was also observed that the DO decreased rapidly at the prophase of fermentation when mycelia quickly accumulated and then rose in the enzyme production stage (Fig. 2a). In addition, the cell concentration (OD600nm) in immobilized-cell fermentation was much lower than in the suspended mycelia fermentation, and there was a downward trend before reaching the maximum (Fig. 2a). Figure 3 shows SEM images of the activated carbon filter sponge carrier and immobilized P. citrinum. The rough surface of the activated carbon filter sponge carrier with increased surface area (Fig. 3a–c) was suitable for immobilizing mycelia and allowed the stable attachment of microorganisms, which promoted the formation of biofilm (Fig. 3d–f). At the end of the multi-batch fermentation, the enzyme activity and productivity reached 456 U/mL and 6.57 U/mL/h, respectively. Compared to the free-cell fermentation (Fig. 1), shorter fermentation period, relatively higher residual sugar, and higher productivity were achieved in immobilized-cell fermentation (Fig. 2b).
SEM images of activated carbon filter sponge carrier and immobilized P. citrinum. a, b, c Activated carbon filter sponge carrier without P. citrinum magnified ×100, ×500, ×1000, respectively. d, e, f Activated carbon filter sponge carrier with immobilized P. citrinum magnified ×100, ×500, ×1000, respectively
It should be noted that the less amount of suspended mycelia and the lack of wheat bran in the broth of the immobilized-cell fermentation reduced the viscosity of the broth, which facilitated mass transfer and downstream processing and allowed the mycelia in the activated carbon filter sponge to continue to grow and produce nuclease P1 for an extended period without losing productivity.
Semi-continuous immobilized fermentation in an ALR
As shown in Fig. 4, semi-continuous fermentation showed similar trends in DO, pH, cell concentration, residual sugar concentrations, and nuclease P1 production to those in repeated-batch fermentation. Nevertheless, the eventual pH values dropped to about 4.45 (Fig. 4b), which was higher than that of repeated-batch fermentation (Fig. 2b) and free-cell fermentation (Fig. 1) suggesting different metabolites might have been consumed and secreted by P. citrinum under different fermentation modes. To further evaluate the long-term stability of semi-continuous immobilized fermentation process, immobilized-cell fermentation was repeated for eight cycles over 397 h. The productivity reached 8.76 U/mL/h (average of eight batches), which was 80.2 and 33.3 % higher than that of free-cell fermentation and repeated-batch fermentation, respectively. In the early stage of each cycle, lag time was much shorter, cells accumulated more quickly, and the production of enzyme was earlier than in repeated-batch fermentation (Fig. 2). As shown in Fig. 5, the comparison of the enzyme productivity by repeated-batch fermentation and semi-continuous immobilized fermentation by P. citrinum in an ALR was analyzed in our study. As expected, decreased substrate consumption, shorter fermentation period (Fig. 4b), and higher productivity (Fig. 5) were obtained during semi-continuous immobilized fermentation. However, the use of semi-continuous fermentation with an immobilized culture for nuclease P1 production has not been well studied before.
Yield characteristics of immobilized fermentation
Nuclease P1 activity obtained from semi-continuous immobilized fermentation was 504 U/mL (average of eight batches), which was 21 and 11 % higher than that of free-cell fermentation and repeated-batch fermentation, respectively. This increase in activity may be due to the increase in yield of nuclease P1, or improvement in enzymatic properties, or both. As shown in Table 1, after the determination of protein concentration by Bradford’s method, the specific activities of nuclease P1 in free-cell mode, repeated-batch mode, and semi-continuous immobilized mode were 1592, 1619, and 1627 U/mg, respectively. Hence, the increase in nuclease P1 activity was likely due to higher yield rather than an improvement in enzymatic activity.
To analyze protein secretion during fermentation by P. citrinum, SDS-PAGE was used to determine the constituents of the enzyme solution. The migration of nuclease P1 with a molecular weight of approximately 43 kDa (Ying et al. 2006) was consistent with the reference. Bands representing nuclease P1 from immobilized-cell fermentation were much more distinct than those from free-cell fermentation (Fig. 6), particularly those from semi-continuous immobilized fermentation. However, the secretion of non-specific protein impurity increased as well. For comparison, the fermentation broth containing nuclease P1 was purified using the method of ammonium sulfate precipitation (Ying et al. 2006), and protein impurities were effectively removed. As shown in lanes 8 and 9, the number of protein impurity bands was significantly reduced in purified enzyme solution.
SDS-PAGE analysis of enzyme solutions (lanes 1 protein molecular mass markers, 2 free-cell fermentation, 3–4 repeated-batch fermentation, 5–7 semi-continuous immobilized fermentation, 8–9 purified enzyme solution after ammonium sulfate precipitation). The red arrow points to the bands of nuclease P1
Discussion
This study demonstrated the advantages of immobilized fermentation over conventional free-cell fermentation in an ALR for nuclease P1 production. Our results were especially robust for the semi-continuous immobilized fermentation process, which has been shown to exhibit higher productivity, lower substrate consumption, and higher product purity. The results also demonstrated that improved oxygen and mass transfer could be achieved with activated carbon filter sponge immobilization. Because of the low broth viscosity, easier downstream processing can be achieved and prolonged enzyme production by repeated-batch and semi-continuous immobilized fermentations is possible. In our experiments, the immobilized mycelia were attached to the activated carbon filter sponge, thereby forming a homogeneous biofilm, which can enhance the stability of microorganism against adverse conditions and maintain high and durable cell viability (Wang et al. 2012; Mussatto et al. 2012). Moreover, it was reported that the immobilized cells renew continuously through the proliferation–death dynamic balance at the interface (Na et al. 2000; Cao et al. 2003; Ory et al. 2004). The shorter fermentation period, relatively higher residual sugar, and higher productivity achieved in immobilized-cell fermentation as compared to free-cell fermentation may be attributed to the enhanced oxygen and mass transfer capacity of the immobilized fermentation system (Srivastava and Kundu 1999).
Our analysis suggested that different metabolites might have been consumed and secreted by P. citrinum under different fermentation modes. According to a previous study, intermediate metabolites such as pyruvic acid, lactic acid, and acetic acid can accumulate in the medium and lead to decreased pH if they do not oxidize completely, thereby restraining the synthesis of the enzyme (Shi et al. 2007). We observed that the eventual pH values during semi-continuous immobilized fermentation remained higher than that of repeated-batch fermentation. This illustrated that the catalysis in semi-continuous immobilized fermentation was more complete, indicating the superiority of enzyme production in semi-continuous immobilized fermentation mode by P. citrinum.
In the current study, OD decreased at first and then rose in all three fermentation modes, particularly in immobilized fermentation, which rebounded more robustly. Oxygen demand may be reduced during fermentation anaphase. Hence, aeration could be reduced during the later period of fermentation by P. citrinum, which could effectively reduce energy consumption. With these advantages, the semi-continuous immobilized fermentation process maintained a stable productivity and high enzyme yield for an extended period, making the process attractive for industrial production of nuclease P1.
This study provides new insights on decreasing energy consumption by reducing aeration during the later period of fermentation in ALR systems. These results are very promising and informative for large-scale production of nuclease P1. Since there is limited knowledge regarding the use of activated carbon filter sponge for P. citrinum immobilization, further study of its use for industrial fermentation is justified.
References
Bradford M (1976) A rapid and sensitive method for quantitation of microgram quantities of protein using the principle of protein-dye binding. Anal Biochem 72:248–254
Cao YL, Tian S, Zhao J, Qian C, Yang XS (2003) Cell immobilization for the use of wastewater treatment. Microbiology 30:77–81
Deoda AJ, Singhal RS (2003) 5′-Phosphodiesterase (5′-PDE) from germinated barley for hydrolysis of RNA to produce flavour nucleotides. Bioresour Technol 88:245–250
Fujimoto M, Kuninaka A, Yoshino H (1974) Purification of a nuclease from Penicillium citrinum. Agric Biol Chem 38:777–783
Fujishima T, Uchida K, Yushino H (1972) Enzyme production by molds in sponge culture. J Ferment Technol 50:724–730
Gerald R, Tilak W (1990) Yeast-derived products. In: Yeast Technology. AVI, New York, pp 369–412
He QT (2010) Breeding high-yield strain for nuclease P1 and its application. Nanjing University of Technology, Dissertation
He QT, Li N, Chen XC, Ye Q, Bai JX, Xiong J, Ying HJ (2011) Mutation breeding of nuclease p1 production in Penicillium citrinum by low-energy ion beam implantation. Korean J Chem Eng 28(2):544–549
Hui YS, Amirul AA, Yahya ARM, Azizan MNM (2010) Cellulase production by free and immobilized Aspergillus terreus. World J Microbiol Biotechnol 26:79–84
Kuninaka A (2008) Nucleotides and related compounds. In: Rehm HJ, Reed G (eds) Biotechnology, vol. 6. Wiley-VCH, Germany, pp 561–612
Laemmli UK (1970) Cleavage of structure proteins during the assembly of head of bacteriophage T4. Nature 277:680–685
Lan TQ, Wei D, Yang ST (2013) Enhanced cellulase production by Trichoderma viride in a rotating fibrous bed bioreactor. Bioresour Technol 133:175–182
Na K, Lee Y, Lee WJ, Huh Y, Lee J, Kubo M, Chung S (2000) Characterization of PCB-degrading bacteria immobilized in polyurethane foam. J Biosci Bioeng 90:368–373
Ory DI, Romero LE, Cantero D (2004) Optimization of immobilization conditions for vinegar production. Siran, wood chips and polyurethane foam as carriers for Acetobacter aceti. Process Biochem 39:547–555
Roberto C, Felipe MGA, Lacis LS, Silva SS, Mancilha IM (1991) Utilization of sugar cane bagasse hemicellulosic hydrolysate by Candida guilliermondii for xylitol production. Bioresour Technol 36:271–275
Shi LE, Ying GQ, Zhang XY, Tang ZX, Chen JS, Xiong WY, Liu HZ (2007) Medium optimization for 5′-phosphodiesterase production from Penicillium citrinum using response surface methodology. Food Technol Biotechnol 45(2):126–133
Mussatto SI, Prata MB, Rodrigues L´g R, Teixeira JA (2012) Production of fructooligosaccharides and b-fructofuranosidase by batch and repeated batch fermentation with immobilized cells of Penicillium expansum. Eur Food Res Technol 235:13–22
Song W, Zhang Q, Li HQ, Li GL (2008) Study on the immobilizing Penicillium citrinum cells for producing nuclease p1 using different multiple carriers. J Henan Univ Technol 29(3):51–54
Srivastava P, Kundu S (1999) Studies on cephalosporin-C production in an air lift reactor using different growth modes of Cephalosporium acremonium. Process Biochem 34:329–333
Talabardon M, Yang ST (2005) Production of GFP and glucoamylase by recombinant Aspergillus niger: effects of fermentation conditions on fungal morphology and protein secretion. Biotechnol Prog 21:1389–1400
Wang KM (1999) Nuclease P1 production by immobilized Penicillium citrinum cell. J Chin Cereals Oils Assoc 14(5):44–46
Wang KM, Wang YG (2003) Study on nuclease P1 produced by immobilized Penicillium citrinum cell. J Zhejiang Inst Sci Technol 15(2):90–93
Wang L, Wu DP, Tang PW, Fan XG, Yuan QP (2012) Xylitol production from corncob hydrolysate using polyurethane foam with immobilized Candida tropicalis. Carbohydr Polym 90:1106–1113
Xia LM (1998) Nuclease p1 production by immobilized Penicillium citrinum cells. Acta Microbiol Sinicasd 28(6):449–453
Ying GQ, Shi LE, Yi Y, Tang ZX, Chen JS (2006) Production, purification and characterization of nuclease p1 from Penicillium citrinum. Process Biochem 41:1276–1281
Zhu Y, Knol W, Smits JP, Bol J (1996) Medium optimization for nuclease P1 production from Penicillium citrinum in solid state fermentation using polyurethane foam as inert carrier. Enzyme Microb Technol 18:108
Acknowledgments
This work was supported by the National Outstanding Youth Foundation of China (21025625), the National High-Tech Research and Development Program of China (863) (2012AA021203), the National Basic Research Program of China (973) (2013CB733602), the Major Research Plan of the National Natural Science Foundation of China (21390204), The National Technology Support Program (2012BAI44G01), the National Natural Science Foundation of China, General Program (21376118), the National Natural Science Foundation of China, Youth Program (21106070), the Program for Changjiang Scholars and Innovative Research Team in University (IRT1066), Jiangsu Provincial Natural Science Foundation of China (SBK 201150207, BK2011031), and the Priority Academic Program Development of Jiangsu Higher Education Institutions (PAPD).
Author information
Authors and Affiliations
Corresponding authors
Rights and permissions
About this article
Cite this article
Zhao, N., Ren, H., Li, Z. et al. Enhancement of nuclease P1 production by Penicillium citrinum YL104 immobilized on activated carbon filter sponge. Appl Microbiol Biotechnol 99, 1145–1153 (2015). https://doi.org/10.1007/s00253-014-6163-z
Received:
Revised:
Accepted:
Published:
Issue Date:
DOI: https://doi.org/10.1007/s00253-014-6163-z