Abstract
Introduction and hypothesis
The aim of the study is to demonstrate the impact of the size of implanted mesh in relation to its immunohistochemical reaction implanted into animal models.
Methods
An experimental study utilizing 54 female Sprague Dawley (SD) rats was divided into five groups: control, sham, and study groups (mesh-small [M-S], mesh-medium [M-M], mesh-large [M-L]). The M-S group used a mesh size of 0.2 × 0.2 cm, the M-M group a mesh size of 0.5 × 0.5 cm, and the M-L a mesh size of 0.7 × 1.0 cm. The sham group underwent vaginal dissection with no mesh implantation. The rats were sacrificed using isoflurane overdose on days 7 and 30. The mesh with the surrounding vaginal and bladder wall tissues were removed and processed for histochemical and western blot analysis.
Results
There is a significant increase in IL-1 and TNF-α immunoreactivity in the M-M and M-L groups on day 7 when compared with the sham group with p values of 0.001 and < 0.001 respectively. M-L showed significantly higher immunoreactivity to TNF-α persisting until day 30. All study groups presented a significantly higher immunoreactivity to MMP-2 and NGF on day 7. However, reactivity to NGF does not persist to day 30 in all groups. Immunoreactivity to CD 31 on days 7 and 30 appears significantly greater in the M-M and M-L groups, with the reaction in the M-L group continuing until day 30.
Conclusion
Mesh size is directly proportional to the inflammatory reaction in the host tissue. The prolonged inflammatory process leads to delayed tissue remodeling and angiogenesis, which could delay mesh–tissue integration.
Similar content being viewed by others
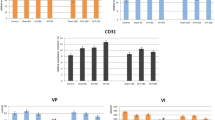
Avoid common mistakes on your manuscript.
Introduction
In 1996, the US FDA (Food and Drug Administration) approved the first sling mesh for the treatment of female SUI (stress urinary incontinence); 6 years later, the first surgical mesh for pelvic organ prolapse (POP) was cleared [1]. Since then, the fate of urogynecological meshes has seen a dramatic rise and fall, with highly publicized mesh-related complications, including infection, chronic pelvic pain, dyspareunia, and extrusion/erosion, ultimately culminating in a 2019 FDA order prohibiting the distribution of transvaginal meshes (TVMs). In the same year, FIGO released a review of statements on the use of synthetic mesh for POP [2], which advocated consideration of second-line TVM procedures only after careful patient selection and thorough counseling on the risk–benefit ratio. As Mangir et al. aptly highlighted, this lag of the regulatory landscape behind advancements in biomedical tissue engineering products remains central to TVM controversies, from which a need for rigorous testing of new candidate materials has been born [3].
It has been well established, however, that the risk of mesh complications differs significantly between slings for SUI and TVM for POP, with the former possessing a lower and less severe course of complications [4]. There remains a wide range of TVM complication rates reported in the existing literature [5], with the PROSPECT randomized prospective trial pegging it at 12% [6]. Risk factors for mesh complications include mesh material and size, surgical technique, implanted site, as well as infection [7]. Polypropylene mesh has been found to be associated with the most favorable host response compared with other material types [8]. The host response to the implanted mesh revolves around inflammation, wound healing, and tissue remodeling. Cytokines such as interleukin-1 (IL-1) and tumor necrosis factor-alpha (TNF-α) are closely intertwined with activated macrophages that migrate to the implant site, and play crucial roles in the early inflammatory response to tissue injury and the presence of a foreign body [9, 10]. Nerve growth factor (NGF) is a neurotrophin that plays a role in cutaneous wound healing and tissue repair [11]; specific to urogynecology, NGF has been implicated in overactive bladder [12, 13], with high levels following TVM implantation in rat models being associated with lower urinary tract dysfunction [14, 15]. Angiogenesis is vital to wound healing, and the expression of CD-31 on platelets, monocytes, granulocytes, and endothelial cells facilitates this process [16]. Matrix metalloproteinases (MMPs) are heavily involved in extracellular matrix (ECM) remodeling, with MMP-2 being a key tissue remodeling enzyme that is involved in wound repair by promoting fibroblast migration to the wound site [17]. An imbalance between MMP-2 and its inhibitor, however, results in a pro-inflammatory state, as increased MMP-2 levels can cause excessive ECM degradation that leads to impaired wound healing—this has been shown in both animal [18] and human wound studies [19].
The size of implanted mesh for sling surgeries for the treatment of SUI is much smaller than TVM for POP—as such, we hypothesize that the underlying pathophysiology of this may be related to a lower dose–response inflammatory reaction, which inherently affects wound healing. The primary aim of our study is thus to investigate the impact of the size of vaginally implanted mesh on immunohistochemical host response in a rat model, in the hope of elucidating the possible mechanism behind mesh-related complications of TVM in the treatment of POP.
Materials and methods
This is an experimental study design approved by Chang Gung Hospital Institutional Animal Care and Use Committee (IACUC No. 2016093003), and funded by Chang Gung Memorial Hospital (CMRPG2G0381).
Fifty-four Sprague–Dawley rats 12.8 ± 1.2 weeks old and weighing 302.1 ± 25.1 g were used. They were divided into five groups: control, sham, and three study groups (Mesh-Small [M-S], Mesh-Medium [M-M], Mesh-Large [M-L]). The sham group underwent vaginal dissection with no mesh implanted. Polypropylene meshes measuring 0.2 × 0.2 cm, 0.5 × 0.5 cm, and 0.7 × 1.0 cm were implanted in M-S, M-M, and M-L groups respectively.
Surgical procedure
General anesthesia was administered using isoflurane in an animal laboratory. After pre-operative antibiotic prophylaxis (cefazolin) had been given, the rat’s vagina was exposed using a Lone Star retractor (Cooper Surgical), followed by hydrodissection with 0.5 to 1.0 cc normal saline along the anterior vaginal wall. A 1-cm midline incision was then made, and the lateral dissection performed between the bladder and vaginal planes was similar for all. This was the extent of surgery for the sham group. For the study groups, polypropylene mesh (Gynemesh; Gynecare, Somerville, NJ, USA) pieces of different sizes (described above) were then inserted into the opened space. Polyglactin 5–0 suture (Vicryl) was used to close the vaginal incision, and subcutaneous Buprenex (0.1 mg/kg) was injected for analgesia at the end of the procedure.
Immunochemical analysis
Euthanasia of the rats via isoflurane overdose and excision of the implanted mesh with surrounding vaginal and bladder wall tissues were performed on days 7 and 30. Half of each tissue sample was prepared in formalin-fixed, paraffin-embedded tissue sections for immunochemistry and the other half of each tissue sample was homogenized for Western blot analysis. The harvested tissue was immediately fixed in 4% formaldehyde for 4 h, and dehydrated by a series of graded ethanol solutions before being embedded in paraffin. The embedded tissue was then sectioned onto glass slides. Immunochemistry was performed on formalin-fixed, paraffin-embedded tissue sections using a standard protocol as described previously [15].
Tissue slides were deparaffinized with xylene and washed in serial dilutions of ethanol. 3% hydrogen peroxidase (H2O2) was used to block endogenous peroxidase activity, after which appropriately diluted primary antibody (rabbit anti-NGF antibody [1:750; anti-NGF/TA300799/OriGene], rabbit anti-CD31 polyclonal antibody [1:200; PA5–24411/Invitrogen], rabbit MMP-2 polyclonal antibody [1:500; TA330021/OriGene], rabbit anti-IL-1-beta polyclonal antibody [1:200; TA336742/OriGene], and rabbit anti-TNF-alpha polyclonal antibody [1:300; PA5–19810/Thermo]) were applied. The slides were washed with phosphate-buffered saline (PBS) at each step. Appropriately diluted biotinylated secondary antibody (1:200; SIG-A0545/Sigma) was applied, followed by chromogenic detection using DAB as the substrate. The slides were counterstained in hematoxylin, and dehydrated with ethanol and xylene prior to mounting and examination via an optical microscope.
Western blot analysis
The samples were homogenized in a lysis buffer (PRO-PREP™ solution, iNtRON Biotechnology) and incubated for 20 min on ice to induce cell lysis [15]. The lysis was centrifuged at 13,000 rpm (4°C) for 10 min, and the supernatant transferred to a fresh 1.5-ml tube. The protein content of the supernatant was estimated using the Bradford method. The samples (30 μg per lane) were mixed with sample buffer containing 10% mercaptoethanol (Sigma). The mixtures of lysates and sample buffers were heated at 100°C for 10 min and applied to a 10% sodium dodecyl sulfate polyacrylamide gel for electrophoresis. The proteins were electrophoretically transferred onto nylon membranes and nonspecific bindings blocked for 1 h at room temperature with 10% (w/v) milk. After repeated washing with TBS containing 0.1% (v/v) Tween 20 (TBST), the membranes were incubated overnight at 4°C with the antibody at 1:10,000 dilution (anti-NGF/TA300799/OriGene; anti-IL-1 antibody/TA336742/OriGene; anti-MMP2 antibody/TA336592/OriGene; anti-TNF antibody/PA5–19810/Thermo). After rinsing in the TBST three times, each of 10 min duration, the membranes were incubated with goat anti-rabbit lgG horseradish peroxidase conjugate antibody (SIG-A0545, Sigma, 1:10,000). The membrane was then incubated in chemiluminescence reagent for 5 min and exposed to high performance chemiluminescence film. The film was developed and used to measure optical density. The optical density of the band was quantified by using the UN-SCAN-IT™ gel and graph digitizing software. The UN-SCAN-IT Graph Digitizer software converted graph images to their underlying data and the magnitude of change in immunochemical analysis was obtained. β-actin was used as the internal control.
Outcome measures
The primary outcome measure was the density of inflammatory reaction produced by IL-1, TNF-α, NGF, MMP-2, and CD-31 around the implanted area.
Statistical analysis
Using the same mesh size implanted in previous studies [14, 15] in our M-M group, three study groups were designed to incorporate a smaller and larger mesh size in the M-S and M-L groups respectively. As this was a pilot study, sample size calculation was not performed. Descriptive statistics were used in the analysis of the results of NGF, IL-1, TNF-α, MMP-2, and CD-31, with all data expressed as mean ± standard deviation (SD). The differences among groups and pair-wise comparisons for continuous parametric variables were analyzed using one-way ANOVA and independent samples t tests for NGF, IL-1, TNF-α, MMP-2, and CD-31 results. ANOVA and post hoc Sidak test were applied for comparison. p < 0.05 was considered statistically significant. All statistical methods were performed using the commercial software SPSS, version 17 (SAS Institute, Cary, NC, USA).
Results
All 54 rats survived the surgery with no post-operative complications (such as wound dehiscence or mesh exposure). Immunohistochemical analysis of the markers IL-1, TNF-α, MMP-2, NGF, and CD 31 and the magnitude of change in all five groups are shown in Table 1 and Fig. 1
The magnitude of change in immunochemical evaluation a IL-1, b TNF-α, c MMP-2, d NGF, and CD31 on day 7 and day 30 after transvaginal mesh surgery in Sprague–Dawley rats. M-S, 0.2 × 0.2 cm mesh implanted; M-M, 0.5 × 0.5 cm mesh implanted; M-L, 0.5 × 1.0 cm mesh implanted, normal-control, 7D Day 7, 30D Day 30. *Statistically significant when compared with sham. #Statistically significant when compared with sham M-S. +Statistically significant when compared with sham M-M
The density of inflammatory reaction was consistently and significantly highest in the M-L group across all the markers, followed by M-M, M-S, and finally the sham group. Analysis of inflammatory cytokines IL-1 and TNF-α showed no difference between sham and M-S at days 7 and 30, and a significantly increased immunoreactivity for M-M and M-L groups at day 7 compared with both sham and M-S, whereas this pattern resolved at day 30 for M-M, M-L, demonstrating persistence. Inter-study group comparison showed a proportionate increase in immunoreactivity for both cytokines at day 7 for M-M and M-L compared with M-S; this again resolved at day 30 for M-M with persistence in M-L. Although there was a difference in immunoreactivity for TNF-α between M-M and M-L groups at days 7 and 30, this was not observed for IL-1.
In terms of MMP-2, there was a significantly increased immunoreactivity for all three study groups compared with sham at day 7, which resolved in the M-S group at day 30, but persisted in the M-M and M-L groups. Inter-study group comparison showed a proportionate increase in immunoreactivity at both time points from small to large mesh sizes.
Immunoreactivity to NGF at day 7 was directly proportionate to the size of the mesh implanted, with a significantly increasing trend from small to large mesh sizes; this demonstrated resolution for all three study groups at day 30.
At days 7 and 30, there was no difference in CD-31 between sham and M-S, and a significantly increased immunoreactivity for M-M and M-L compared with the sham group. Inter-study group comparison showed no difference between M-S and M-M at both time points, whereas M-L showed a persistent increase in immunoreactivity compared with M-S and M-M at day 30.
Our findings of immunohistochemical analysis were corroborated by the Western blot results, with darker and thicker lines, as shown in Figs. 2 and 3.
Western blot analysis in IL-1, TNF-α, MMP-2, NGF, and CD31 on day 7 and day 30 after transvaginal mesh surgery in SD rats. a Control. b Sham-7D, surgery alone at day 7; c M-S-7D, 0.2 × 0.2-cm mesh implanted at day 7; d M-M-7D, 0.5 × 0.5-cm mesh implanted at day 7; e M-L-7D, 0.7 × 1.0-cm mesh implanted at day 7; f Sham-30D, surgery alone at day 30; g M-S-30D, 0.2 × 0.2-cm mesh implanted at day 30; h M-M-30D, 0.5 × 0.5-cm mesh implanted at day 30; i M-L-30D, 0.7 × 1.0-cm mesh implanted at day 30
Immunochemistry staining IL-1, TNF-α, MMP-2, NGF, and CD-31 of urogenital tissues at days 7 and 30 post-mesh implantation (×100 magnification). a1 Day 7 post-implant in the M-S group; a2 day 30 post-implant in the M-S group; b1 day 7 post-implant in the M-M group; b2 day 30 post-implant in the M-M group; c1 day 7 post-implant in the M-L group; c2 day 30 post-implant in the M-L group; d1 day 7 post-implant on Sham group; d2 day 30 post-implant on Sham group; f Control group. *Brown spots signify antibody staining
Discussion
The complications of TVM have been well publicized and include infection, dyspareunia, mesh erosions, and chronic pelvic pain [4,5,6]. With increasing public skepticism and the marked decline of TVM usage in many markets following the FDA order, there is an urgent need for robust investigation of factors contributing to these mesh complications in physiologically relevant animal models.
To the best of our knowledge, this is the first rat model study comparing the differences in short- and mid-term inflammatory host response resulting from various sizes of polypropylene mesh implanted in the vagina. Earlier studies compared different types of synthetic and biologic graft materials and found that different foreign body responses were evoked following implantation in the vagina and abdomen of rabbits and sheep [20,21,22,23,24]. Manodoro et al. [25] showed that mesh insertion into the rectovaginal septum of sheep was associated with exposure and contraction with a possible association with mesh size, but lacked data on immunohistochemical analysis. More recent publications investigating mesh-related host response have utilized the same mesh sizes among their study groups and include studies involving mesh implantation into abdominal subcutaneous tissue of rats, with Prudente et al. demonstrating increased inflammatory activity in the early post-implant phase [26], and Bronzatto and Riccetto comparing standard- and light-weight mesh implantation and showing increased expression of IL-1, MMP-2, and MMP-3 at day 30, possibly representing a longstanding inflammatory response [27]. Using an investigational protocol similar to our current study, Lo et al. previously compared host response between collagen-coated and non-coated polypropylene mesh pieces in the vagina of rats, and found that the presence of collagen-coating triggered greater inflammation [24].
As such, our study showed a consistently greater inflammatory host response to increasing sizes of mesh implanted, which was not unexpected. An important and interesting insight that our results shed light on, however, is that the magnitude and duration of inflammatory reaction differs disproportionately depending on the size of mesh used: in the M-S group for example, there appeared to be no difference in inflammatory reaction compared with sham surgery (other than transiently elevated MMP-2, which resolved at day 30), suggesting that the host may not perceive any additional insult other than the tissue injury sustained when small pieces of mesh are implanted. Although both medium- and large-sized meshes evoked greater inflammatory response in the early post-implantation phase, as demonstrated by increased immunoreactivity to pro-inflammatory cytokines IL-1 and TNF-α, this resolved by the 1st post-operative month in the former in contrast with the latter group, which showed persistent mid-term inflammatory reaction at day 30.
Tissue remodeling occurred in all three mesh study groups, with significantly increased immunoreactivity to MMP-2. The effect of mesh size on the duration of remodeling and angiogenesis was reflected by the M-S group once again showing resolution at day 30, combined with the fact that there was no difference in CD-31 compared with sham surgery throughout the study period. Medium- and large-sized meshes, on the other hand, demonstrated evidence that this process was still on-going at 1 month post-implantation, with M-L showing the greatest magnitude of change. Together with the findings of IL-1 and TNF-α, this suggests that large-sized meshes have a propensity to incite a large inflammatory host response with a protracted wound healing process.
The initial increased immunoreactivity to NGF in all three mesh study groups had resolved at day 30, suggesting that the role it plays might be largely relevant in the early post-implantation phase: the association with lower urinary tract dysfunction at day 10 reported in a previous study [14] may hence be transient. Although urodynamic studies were beyond the scope of our current study, this remains an uncharted area for future exploration.
The strengths of our study include that of an experimental study, which was performed in a controlled environment and was specifically designed to simulate TVM placement in female POP surgeries. We also used a good overall sample size, although we acknowledge that the subdivision into groups with different mesh sizes and subsequent analysis at short- and mid-term intervals may have diluted the strength of our findings. Our limitation is that of a short study period, which may be inadequate to fully elucidate the complexities of the entire wound healing process.
Conclusion
In conclusion, our results suggest that increasing sizes of mesh do not incite a directly proportionate, dose–response host inflammatory response; instead, there may well be a threshold of mesh size beyond which the pathophysiological balance between wound healing with optimal host recovery versus persistent pro-inflammatory state with a prolonged process of tissue remodeling/repair (i.e. impaired wound healing) is upset. This is critical in today’s urogynecology mesh climate. What is then the ideal proportioned TVM size that is large enough to ensure good long-term cure for POP and yet evoke an appropriate non-excessive patient inflammatory response resulting in the lowest possible risk of mesh-related complications? With many questions yet unanswered following the FDA’s advisory, larger scale animal studies on long-term host response to vaginally implanted mesh are urgently needed to delve further into this important variable of mesh size, alongside other known risk factors for mesh-related complications.
References
US Food and Drug Administration (FDA). Urogynecologic surgical mesh: update on the safety and effectiveness of vaginal placement for pelvic organ prolapse. Silver Spring, MD:FDA; 2011.
Ugianskiene A, Davila GW, Su TH, FIGO Urogynecology and Pelvic Floor Committee. FIGO review of statements on use of synthetic mesh for pelvic organ prolapse and stress urinary incontinence. Int J Gynaecol Obstet. 2019;147(2):147–55.
Mangir N, Roman S, MacNeil S. The changing regulatory landscape for biomedical implants and its relationship to withdrawal of some vaginal mesh products. Curr Opin Urol. 2019;29(4):414–8.
Abbott S, Unger CA, Evans JM, Jallad K, Mishra K, Karram MM, et al. Evaluation and management of complications from synthetic mesh after pelvic reconstructive surgery: a multicenter study. Am J Obstet Gynecol. 2014;210(2):163.e1–8.
Abed H, Rahn DD, Lowenstein L, Balk EM, Clemons JL, Rogers RG, et al. Incidence and management of graft erosion, wound granulation, and dyspareunia following vaginal prolapse repair with graft materials: a systematic review. Int Urogynecol J. 2011 Jul;22(7):789–98.
Glazener CM, Breeman S, Elders A, Hemming C, Cooper KG, Freeman RM, et al. Mesh, graft, or standard repair for women having primary transvaginal anterior or posterior compartment prolapse surgery: two parallel-group, multicentre, randomised, controlled trials (PROSPECT). Lancet. 2017;389(10067):381–92.
Patel H, Ostergard DR, Sternschuss G. Polypropylene mesh and the host response. Int Urogynecol J. 2012;23(6):669–79.
Kelly M, Macdougall K, Olabisi O, McGuire N. In vivo response to polypropylene following implantation in animal models: a review of biocompatibility. Int Urogynecol J. 2017;28(2):171–80.
Ito Y, Kaneko N, Iwasaki T, Morikawa S, Kaneko K, Masumoto J. IL-1 as a target in inflammation. Endocr Metab Immune Disord Drug Targets. 2015;15(3):206–11.
Anderson JM, Rodriguez A, Chang DT. Foreign body reaction to biomaterials. Semin Immunol. 2008;20(2):86–100.
Kawamoto K, Matsuda H. Nerve growth factor and wound healing. Prog Brain Res. 2004;146:369–84.
Liu HT, Chen CY, Kuo HC. Urinary nerve growth factor levels in overactive bladder syndrome and lower urinary tract disorders. J Formos Med Assoc. 2010;109(12):862–78.
Sheng W, Zhang H, Ruth KH. Could urinary nerve growth factor be a biomarker for overactive bladder? A meta-analysis. Neurourol Urodyn. 2017;36(7):1703–10.
Lo TS, Lin YH, Chu HC, Cortes EFM, Pue LB, Tan YL, et al. Association of urodynamics and lower urogenital tract nerve growth factor after synthetic vaginal mesh implantation on a rat model. J Obstet Gynaecol Res. 2017;43(1):173–8.
Lo TS, Lin YH, Uy-Patrimonio MC, Chu HC, Hsieh WC, Chua S. Dissecting of the paravesical space associated with lower urinary tract dysfunction—a rat model. Sci Rep. 2020;10(1):1718.
La Fleur M, Underwood JL, Rappolee DA, Werb Z. Basement membrane and repair of injury to peripheral nerve: defining a potential role for macrophages, matrix metalloproteinases, and tissue inhibitor of metalloproteinases-1. J Exp Med. 1996;184(6):2311–26.
Nguyen T, Mobashery S, Mayland C. Roles of matrix metalloproteinases in cutaneous wound healing. In: Alexandrescu (ed) Wound healing: new insights into ancient challenges. 2016. https://doi.org/10.5772/64611.
Liang R, Zong W, Palcsey S, Abramowitch S, Moalli PA. Impact of prolapse meshes on the metabolism of vaginal extracellular matrix in rhesus macaque. Am J Obstet Gynecol. 2015;212(2):174.e1–7.
Ayuk SM, Abrahamse H, Houreld NN. The role of matrix metalloproteinases in diabetic wound healing in relation to photobiomodulation. J Diabetes Res. 2016;2016:2897656.
Hilger WS, Walter A, Zobitz ME, Leslie KO, Magtibay P, Cornella J. Histological and biomechanical evaluation of implanted graft materials in a rabbit vaginal and abdominal model. Am J Obstet Gynecol. 2006;195(6):1826–31.
Pierce LM, Rao A, Baumann SS, Glassberg JE, Kuehl TJ, Muir TW. Long-term histologic response to synthetic and biologic graft materials implanted in the vagina and abdomen of a rabbit model. Am J Obstet Gynecol. 2009;200(5):546.e1–8.
Endo M, Urbankova I, Vlacil J, Sengupta S, Deprest T, Klosterhalfen B, et al. Cross-linked xenogenic collagen implantation in the sheep model for vaginal surgery. Gynecol Surg. 2015;12(2):113–22.
Feola A, Endo M, Urbankova I, Vlacil J, Deprest T, Bettin S, et al. Host reaction to vaginally inserted collagen containing polypropylene implants in sheep. Am J Obstet Gynecol. 2015;212(4):474.e1–8.
Lo TS, Lin YH, Yusoff FM, Chu HC, Hsieh WC, Uy-Patrimonio MC. The immunohistochemical and urodynamic evaluation towards the collagen-coated and non-coated polypropylene meshes implanted in the pelvic wall of the rats. Sci Rep. 2016;6:38960.
Manodoro S, Endo M, Uvin P, Albersen M, Vláčil J, Engels A, et al. Graft-related complications and biaxial tensiometry following experimental vaginal implantation of flat mesh of variable dimensions. BJOG. 2013;120(2):244–50.
Prudente A, Favaro WJ, Latuf Filho P, Zanettini Riccetto CL. Host inflammatory response to polypropylene implants: insights from a quantitative immunohistochemical and birefringence analysis in a rat subcutaneous model. Int Braz J Urol. 2016;42(3):585–93.
Bronzatto E, Riccetto CLZ. Pro-inflammatory cytokines and metalloproteinase activation in polypropylene mesh implant in rat subcutaneous tissue. Int Braz J Urol. 2018;44(4):819–25.
Acknowledgements
This study was supported by Chang Gung University Hospital Research Grants CMRPG2G0381, and approved by Chang Gung Hospital’s Institutional Animal Care and Use Committee IACUC No. 2016 093003.
Financial disclaimer
This study was supported by Chang Gung University Hospital Research Grants CMRPG2G0381.
Author information
Authors and Affiliations
Contributions
T.S. Lo: protocol/project development, data collection, data analysis, manuscript editing; Y.H. Lin: data collection/data analysis; S. Chua: data collection, data analysis and manuscript editing; H.C. Chu: laboratory handling, data collection; M.C. Uy-Patrimonio: manuscript writing; K.L. Ng: data analysis and manuscript editing.
Corresponding author
Ethics declarations
Conflicts of interest
No further conflicts of interest are claimed.
Additional information
Publisher’s note
Springer Nature remains neutral with regard to jurisdictional claims in published maps and institutional affiliations.
Rights and permissions
About this article
Cite this article
Lo, TS., Lin, YH., Chua, S. et al. Immunochemical analysis on polypropylene mesh: does mesh size make a difference?. Int Urogynecol J 32, 47–55 (2021). https://doi.org/10.1007/s00192-020-04399-x
Received:
Accepted:
Published:
Issue Date:
DOI: https://doi.org/10.1007/s00192-020-04399-x