Abstract
In past studies, it is reported that surface passivation helps to minimize surface leakage current in case of photodetectors. However, utilization of stack of passivation layers for Metal–Semiconductor–Metal (MSM) type detectors is studied in very few reports. In present study, we have investigated the effect of single passivation and stack of passivation layers on the performance parameters of AlGaN MSM detector as GaN or AlGaN layer exhibits higher surface state density, for three types of passivation layers and without passivation case also. Passivation layers like SiO2, Al2O3, and Al2O3/SiO2 (stack) have been utilized for investigation analysis. The comparison of performance parameters of passivated detectors and without passivation-based detector has been performed so that suitable selection of passivation can be done for enhanced performance. The considered performance parameters of detector are dark current density, electric field, recombination rate, and photocurrent-to-dark current density ratio. Comparison analysis shows that detector with Al2O3/SiO2 passivation stack provides lowest dark current density of 5e−08 A/cm2 at top surface of AlGaN layer as compared to other three types of detectors. Moreover, highest electric field, highest photocurrent-to-dark current density ratio, and lower recombination rate have been obtained with Al2O3/SiO2 passivated stack-based detector. Present work helps to select the type of passivation for AlGaN MSM detector so as to provide improved performance parameters and reliable UV detection for low noise applications.
Access provided by Autonomous University of Puebla. Download conference paper PDF
Similar content being viewed by others
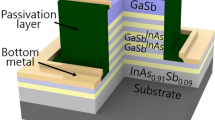
Keywords
1 Introduction
The MSM detector structure is preferred among other types of detector structures (like, p-n, PIN and Schottky barrier) due to easy fabrication processes and planar design flexibility [1, 2]. The performance of photodetector is highly dependent on the material properties of photon-absorbing semiconductor layer and geometry parameters [3].
AlGaN or GaN MSM detectors have become a promising solution for providing very low dark current/density due to wide band gap. These types of detectors are capable of providing high responsivity or photocurrent and thermally stable operation. Schottky contacts on AlGaN layer of MSM detector provide low dark current/density and low capacitance due to rectifying nature [2, 4]. MSM photodiodes have intrinsic ability of providing higher UV/visible ratio and linear optical power response [5].
The influence of surface passivation of AlGaN/GaN detectors has been analyzed by authors and demonstrated an improvement in various device performance parameters and characteristics [6,7,8]. GaN or AlxGa1−xN material layer exhibits high surface density states; thus, surface passivation with insulating materials like SiO2, Al2O3, and SiNx helps to reduce undesirable effects of surface states and saturation current [9, 10].
For efficient UV light detection, photodetector with low dark current or dark current density is required so as to enhance UV light to dark current ratio and noise. Therefore, surface passivation and anti-reflecting coatings are generally required for photodetectors. In this paper, effect of different types of passivation layers and utilization of passivation stack on the performance parameters of detector has been studied.
This section is followed by device structure description and simulation methodology heading. Afterward, results and discussions have discussed. Finally, conclusion of present research work is presented.
2 Device Structure Description and Simulation Methodology
The geometry of AlGaN MSM structure has been designed using Silvaco Atlas tool. Both x and y dimensions of selected MSM structure are 50 μ as shown in Fig. 1. The thickness of both Al0.5Ga0.5N and AlN layers is taken 500 nm. AlN layer is used as buffer layer between AlGaN and Sapphire substrate to minimize the crystal constants mismatch [11]. The Schottky contacts on AlGaN layer have been deposited by utilizing gold metal electrodes for getting higher Schottky Barrier Height (SBH). Moreover gold (Au) provides best transmittance in UV range as compared to platinum, nickel, etc. [12]. The thickness of four metal fingers or Schottky contacts is 20 nm. The width (W) and spacing (S) between electrodes are taken as 5 and 10 µm. The spacing between metal electrodes is taken twice so as to minimize reflection of incoming light and to absorb higher amount of incident light [13, 14]. However, transit time of photo-generated carriers can be slightly affected which could be overcome by applying high Reverse Bias voltage [4].
Table 1 shows doping levels of semiconductor layers and Sapphire substrate of proposed Al0.5Ga0.5N/AlN/Sapphire detectors.
The geometry parameters are taken same for all MSM detectors considered under investigation. These parameters include device dimensions, thickness of all layers, doping levels, number of metal electrodes (fingers), and their dimensions. In Fig. 1, dark blue color layer is representing Sapphire, Green color layer is AlN, and peach colored layer is of AlGaN material that is known photo-absorbing layer. That’s why detector under investigation is written AlGaN/AlN/Sapphire type MSM detector. On the top of AlGaN layer, four sky blue colored metal electrodes are shown. In between these electrodes, Al2O3 passivation layer of red color is shown. The thickness of passivation layer is considered same as the thickness of metal electrodes.
In Fig. 2, the device dimensions and name of all structure layers are same as specified in Fig. 1. However, in between metal electrodes, Al2O3/SiO2 surface passivation stack is shown. In this stack, SiO2 passivation can be seen by sky blue color and on it Al2O3 passivation of red color can be seen. The thickness of both the passivation layers in stack is 10 nm each. The metal electrodes are always interconnected to be termed as anode and cathode terminals. Then, reverse bias voltage is applied to both the electrodes so that photodetector converts incident light energy to electrical current. Thus, transport of current in metal–semiconductor contact is due to the movement of majority carriers [15].
Previous studies show that with the use of passivation layer, performance parameters of detector can be enhanced [16]. However, detector surface passivation using passivation layers stack can demonstrate more excellent improvement in performance as compared to single layer passivation [17].
Adequate list of material parameters associated to Al0.5Ga0.5N, AlN, and Sapphire have been utilized in Atlas code. These include bandgap at 300 K, electron affinity, permittivity, absorption coefficient (wavelength dependent), optical, and lattice constants. The material parameters for Al0.5Ga0.5N and AlN layer are taken from several references [18,19,20]. Gold Schottky contact with work function 5.1 eV has been defined to get Schottky Barrier Height of 1.8 eV at metal–semiconductor interface. Past studies show that barrier height of Schottky contact gets increased due to surface modification that helps to reduce dark current [21, 22]. Physical models have defined using model and impact statements in the program code. Physical models related to carrier statistics, mobility, recombination, and impact ionization have been defined in the Atlas code. Fermi Dirac statistics have been used so that certain properties of highly doped material can be accounted. Effective density of states related to electrons and holes has been defined for semiconductor materials. Trap density of active material is assumed to be ideal. Default mobility model parameters for Al0.5Ga0.5N, GaN, and AlN material have been utilized in program code are based on low field and are taken from [23]. The electron–hole pairs get generated in active layer when wavelength of incident light corresponds to band gap of semiconductor layer. The wavelength of incident light for Al0.5Ga0.5N/AlN/Sapphire detector is taken as 270 nm which leads to band gap of 4.4 eV which is mentioned in Atlas-Silvaco program code. The power intensity of UV light is taken 1 W/cm2 for all detectors under simulation work.
3 Results and Discussions
Four Al0.5Ga0.5N/AlN/Sapphire MSM detectors with surface passivation layer have been designed and simulated for extracting performance parameters. Thickness of single passivation layer is taken same as that of thickness of metal electrodes. However, for passivation stack, thickness of each passivation is half of metal electrode thickness. First, MSM detector is without passivation layer. Second, detector is passivated with conventional SiO2 material-based passivation. Third, MSM detector is Al2O3 material-based passivation layer and fourth is based on Al2O3/SiO2 passivation stack. The cost of Al2O3 has reported to be low that makes it suitable for various cost-effective light detection applications [24].
Table 2 represents the dielectric values of some commonly utilized insulating materials that are preferred for surface passivation of AlGaN detectors.
Some of the materials like β-Ga2O3, SiNx are reported non-suitable for the passivation of AlGaN type detectors. The reason is that β-Ga2O3 passivation requires high temperature oxidation which can cause damage to AlGaN layer. SiNx passivation causes etching process trouble during fabrication processes [7].
The performance parameters of unpassivated, single passivation layer-based Al0.5Ga0.5N/AlN/Sapphire MSM detectors have been compared with Al2O3/SiO2 passivation stack-based detector. The comparison of performance parameters of four MSM detectors is given in Table 3.
In Table 3, the average value of dark current density in MSM detector without passivation layer is 8e−08 A/cm2. This value can be approximated with the dark current density mentioned in [26] which states that larger finger spacing provides lower dark current as compared to equal finger width and spacing-based MSM detector. Moreover, the dark current density plots of detector structures with passivation show less noise as compared to detector which is without passivation. The dark current density plots of unpassivated and Al2O3 passivation-based MSM detectors are shown in Fig. 3a, b. In simulation work, the horizontal cut-line has been drawn in Al0.5Ga0.5N layer at 100 nm from the top surface to extract these plots.
The dark current or leakage current gets reduced due to surface passivation [22] as the density of energy states gets reduced. Therefore, electrical characteristics of device get improved [27]. In Table 3, lowest dark current density of 5e−08 A/cm2 and highest photocurrent-to-dark current density ratio have been obtained for Al2O3/SiO2 passivation stack-based MSM detector. The reason for lower current density can be explained as the reduction of surface states of AlGaN that lead to minimization of electronic state density at Al2O3/SiO2/AlGaN interface [28]. Therefore, device performance parameters get improved.
As specified in [23], photocurrent is a measure of photo-absorption rate in the active semiconductor layer and can be taken as current density. In present simulation work, similar photocurrent value has been obtained at 20 V for all detector structures in I-V characteristics plots. Figure 4 shows I-V characteristic plot of Al2O3/SiO2 passivation stack-based detector in Reverse bias varying from 0 to 20 V. Red line shows dark current which is very low as compared to photocurrent. Green color line shows photocurrent which starts to increase from 15 V threshold voltage. The maximum value of photocurrent at 20 V is approximately 8.5 mA.
The electric field near the electrodes is highest in MSM detector which is based on Al2O3/SiO2 passivation layer stack. It is mentioned in [14] that higher value of electric field helps to provide higher carrier velocity. Since electron and hole Shockley–Read–Hall recombination lifetimes for AlGaN layer is fixed in each detector under investigation, therefore we obtained least dark current density in this type of MSM detector.
In spectral response, we obtained same cut-off wavelength for all detectors. The cut-off wavelength is 400 nm in case of Al2O3 passivated detector which is shown in Fig. 5.
Results obtained in present work are helpful for analyzing the electrical behavior of AlGaN MSM detectors with and without passivation layer. Therefore, we can state that surface passivation has very less effect on photocurrent and spectral response but significant impact on the dark current, electric field and recombination rate in photo-absorbing layer of MSM photodetector.
4 Conclusion
In preset work, effect of passivation layer and passivation stack has been analyzed for AlGaN MSM detector. The performance parameters of passivated detectors have been compared with unpassivated detector. Passivated detectors using SiO2, Al2O3, Al2O3/SiO2 stack layers have been investigated for performance parameters like dark current density, electric field, recombination rate, and photocurrent-to-dark current density ratio. It is concluded that detector passivated with stack exhibits lowest dark current density and highest photocurrent-to-dark current density ratio as compared to unpassivated and SiO2, Al2O3-based detectors. Therefore, it is important to select appropriate type of passivation material foe enhanced and reliable operation in efficient UV detection applications.
References
Monroy E, Calle F, Munoz E, Omnes F (1999) AlGaN metal–semiconductor–metal photodiodes. Appl Phys Lett 74(22):3401–3403. https://doi.org/10.1063/1.123358
Muñoz E, Monroy E, Calle F, Omnès F, Gibart P (2000) AlGaN photodiodes for monitoring solar UV radiation. J Geophys Res Atmos 105(D4):4865–4871. https://doi.org/10.1029/1999JD900939
Fernández S, Naranjo FB, Sánchez-García MÁ, Calleja E (2020) III-nitrides resonant cavity photodetector devices. Materials 13(19):4428. https://doi.org/10.3390/ma13194428
Xie C, Lu XT, Tong XW, Zhang ZX, Liang FX, Liang L et al (2019) Recent progress in solar‐blind deep‐ultraviolet photodetectors based on inorganic ultrawide bandgap semiconductors. Adv Funct Mater 29(9):1806006. https://doi.org/10.1002/adfm.201806006
Zou Y, Zhang Y, Hu Y, Gu H (2018) Ultraviolet detectors based on wide bandgap semiconductor nanowire: a review. Sensors 18(7):2072. https://doi.org/10.3390/s18072072
Gassoumi M, Gaquiere C, Maaref H (2011) Surface passivation effects on AlGaN/GaN high electron mobility transistors with SiO2. Sensor Lett 9(6):2175–2177. https://doi.org/10.1166/sl.2011.1787
Lee CJ, Park H (2016) Surface passivation method for GaN UV photodetectors using oxygen annealing treatment. 센서학회지 25(4):252–256. https://doi.org/10.5369/JSST.2016.25.4.252
Lu W, Kumar V, Schwindt R, Piner E, Adesida I (2002) A comparative study of surface passivation on AlGaN/GaN HEMTs. Solid-State Electron 46(9):1441–1444. https://doi.org/10.1016/S0038-1101(02)00089-8
Luo B, Mehandru R, Kim J, Ren F, Gila BP, Onstine AH et al (2002) Comparison of surface passivation films for reduction of current collapse in AlGaN/GaN high electron mobility transistors. J Electrochem Soc 149(11):G613. https://doi.org/10.1149/1.1512675
Enisherlova KL, Goryachev VG, Rusak TF, Kapilin SA (2016) Study of the effect of passivation layers on capacitance of AlGaN/GaN heterostructures. Modern Electron Mater 2(4):131–137. https://doi.org/10.1016/j.moem.2016.12.006
Wang G, Xie F, Lu H, Chen D, Zhang R, Zheng Y et al (2013) Performance comparison of front-and back-illuminated AlGaN-based metal–semiconductor–metal solar-blind ultraviolet photodetectors. J Vac Sci Technol B 31(1). https://doi.org/10.1116/1.4769250
Monroy E, Omnès F, Calle FJSS (2003) Wide-bandgap semiconductor ultraviolet photodetectors. Semicond Sci Technol 18(4):R33. https://doi.org/10.1088/0268-1242/18/4/201
Kaur H, Kaur HJ, Hooda MK (2022) Investigating the effect of number of metal electrodes on performance parameters of AlGaN MSM photodetectors. Int J Smart Sens Intell Syst 15(1). https://doi.org/10.2478/ijssis-2022-0015
Averine SV, Chan YC, Lam YL (2001) Geometry optimization of interdigitated Schottky-barrier metal–semiconductor–metal photodiode structures. Solid-State Electron 45(3):441–446. https://doi.org/10.1016/S0038-1101(01)00017-X
Huang Y, Yang J, Zhao D, Zhang Y, Liu Z, Liang F, Chen P (2023) A study on the increase of leakage current in AlGaN detectors with increasing Al composition. Nanomaterials 13(3):525. https://doi.org/10.3390/nano13030525
Liu HY, Liu GJ, Huang RC, Sun WC, Wei SY, Yu SM (2017) In situ growth of Al 2 O 3 as a passivation and antireflection layer on TiO2-based MSM photodetectors. IEEE Sensors J 17(16):5087–5092. https://doi.org/10.1109/JSEN.2017.2724061
Murugapandiyan P, Nirmal D, Hasan MT, Varghese A, Ajayan J, Fletcher AA, Ramkumar N (2021) Influence of AlN passivation on thermal performance of AlGaN/GaN high-electron mobility transistors on sapphire substrate: a simulation study. Mater Sci Eng B 273:115449. https://doi.org/10.1016/j.mseb.2021.115449
Wang X, Jing Y, Zhu X, Liu C, Yun J, Zhang Z (2013) Theoretical study on the optical and electrical properties of Al x Ga 1-x N crystals. https://doi.org/10.4236/jmp.2013.43A063
Murthy V, Srivani A, Raghavaiah G (2017) Physical studies in III-nitride semiconductor alloys. Int J Thin Film Sci Technol 6:15–27
Gelinas RJ (2005) A novel approach to modeling tunnel junction diodes using Silvaco ATLAS software. Doctoral dissertation, Monterey, California. Naval Postgraduate School)
Nico V (2005) Analysis and modelling of MSM and Schottky barrier GaN UV detectors. Doctoral dissertation, MS thesis, Dept. Appl. Phys., Eindhoven Univ. Technol., Eindhoven, The Netherlands
Garg M, Naik TR, Pathak R, Rao VR, Liao CH, Li KH et al (2018) Effect of surface passivation process for AlGaN/GaN HEMT heterostructures using phenol functionalized-porphyrin based organic molecules. J Appl Phys 124(19). https://doi.org/10.1063/1.5049873
Manual AUS, Santa Clara CA (2016) ATLAS user’s manual
Bernát J (2005) Fabrication and characterisation of AlGaN/GaN high electron mobility transistors for power applications. Doctoral dissertation, Aachen, Techn. Hochsch., Diss.
Robertson J (2004) High dielectric constant oxides. Eur Phys J Appl Phys 28(3):265–291. https://doi.org/10.1051/epjap:2004206
Kaur H, Kaur HJ, Hooda MK, Dassi M (2023) Electrical performance analysis of Al0. 5Ga0. 5 N/AlN/Sapphire-based MSM UV detector for high photocurrent. J Optics 52(1):355–364. https://doi.org/10.1007/s12596-022-00904-1
Gupta A, Chatterjee N, Kumar P, Pandey S (2017) Effect of surface passivation on the electrical characteristics of nanoscale AlGaN/GaN HEMT. In: IOP Conf Series Mater Sci Eng 225(1):012095. https://doi.org/10.1088/1757-899X/225/1/012095
Yatabe Z, Asubar JT, Hashizume T (2016) Insulated gate and surface passivation structures for GaN-based power transistors. J Phys D Appl Phys 49(39):393001. https://doi.org/10.1088/0022-3727/49/39/393001
Author information
Authors and Affiliations
Corresponding author
Editor information
Editors and Affiliations
Rights and permissions
Copyright information
© 2024 The Author(s), under exclusive license to Springer Nature Singapore Pte Ltd.
About this paper
Cite this paper
Kaur, H., Hooda, M.K. (2024). Studying the Effect of Type of Surface Passivation Layer on Performance Parameters of AlGaN MSM Detector. In: Shaw, R.N., Siano, P., Makhilef, S., Ghosh, A., Shimi, S.L. (eds) Innovations in Electrical and Electronic Engineering. ICEEE 2023. Lecture Notes in Electrical Engineering, vol 1109. Springer, Singapore. https://doi.org/10.1007/978-981-99-8289-9_35
Download citation
DOI: https://doi.org/10.1007/978-981-99-8289-9_35
Published:
Publisher Name: Springer, Singapore
Print ISBN: 978-981-99-8288-2
Online ISBN: 978-981-99-8289-9
eBook Packages: EnergyEnergy (R0)