Abstract
Silver nanoparticles have been commonly used as an antibacterial agent and are often delivered in a burst release manner to the site of infection. However, a drawback of this release mode is the limited lasting duration of the antibacterial properties of the particles. Hence, in order to achieve a more effective and sustained protection against bacteria growth, this project aims to design and create smart antimicrobial materials based on silver-silica nanocapsules that can respond to an acidic environment to release Ag+ ions in a targeted, slow and sustained manner. In this project, the as-synthesized silica-silver nanocapsules were found to exhibit excellent colloidal stability, thus allowing for a homogenous distribution within different polymer matrix materials. Explored applications include the incorporation of the silver-silica nanocapsules into F127 hydrogel and poly(vinyl alcohol) (PVA) film so as to develop antibacterial biomaterials that can effectively prevent bacteria growth for a sustained period of time. In subsequent proof-of-concept studies, both the F127 hydrogel and PVA film were able to respond to acidic conditions for a gradual release of Ag+ ions. Interestingly, the as-released Ag+ ions from the PVA film were effectively entrapped within the polymer matrix, thereby demonstrating their promising potential to sterilize absorbed fluid from wound sites when applied as a wound dressing. On the other hand, the F127 hydrogel exhibited a slow and sustained release of Ag+ ions into the surrounding environment, hence affirming their capacity for topical administration in the form of lotions or creams for antibacterial purposes.
Access provided by Autonomous University of Puebla. Download conference paper PDF
Similar content being viewed by others
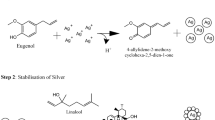
Keywords
- Silver nanocapsules
- Silver-silica nanocapsules
- Antimicrobial
- Sustained release
- Acid responsive
- Stimulated response
1 Background and Purpose of Research Area
Silver ions have been established to have antibacterial properties as they are able to destroy bacteria cells by damaging the cell envelope of bacteria and intracellular content [1]. So far, the antibacterial properties of silver has been utilized in many areas, such as in water purification [2] or in the treatment of burn victims [3]. Such silver ions are utilized in a burst release manner, where all the silver ions present are instantly exposed to their surroundings. However, high concentrations of either silver ions or metallic silver at once can be toxic to the human body [4]. On the other hand, a more controlled release will ensure that the amount of silver ions remain at a level below the toxic threshold of the body as the ions will be slowly exposed to their surroundings and used up accordingly.
Besides, a more limited exposure to silver will also allow for a larger reservoir of silver to be present without being toxic to the human body. Coupled with the design of a stimuli-responsive antibacterial material to allow for a more targeted and controlled release of silver ions, this can potentially increase the lasting duration of antibacterial properties. In this regard, it is noteworthy that certain types of bacteria can create an acidic medium by secreting acids [5], which can act as a stimuli for the release of silver ions. Therefore, this project aims to develop a smart antibacterial material that can respond to acidic conditions to release the silver ions in a sustained and controlled manner. This will hopefully improve current silver-based antibacterial materials by enabling the material to be more effective for a sustained protection against bacteria growth.
2 Engineering Goal of Project
To design and create smart antimicrobial materials based on silver-silica nanocapsules. The silver-silica nanocapsules (AgNCs) will act as a reservoir of silver ions stored in the form of metallic silver on the surface of the nanocapsules. Upon exposure to an acidic environment, the metallic silver will be converted into silver ions for enhanced antibacterial properties. Silver nanocapsules are chosen because they have been found to provide a sustained release of silver ions instead of a burst release when at sizes larger than 50 nm [6]. This aligns with the goal of the project for a sustained protection against bacteria. Their smaller size also allows for more silver to be stored due to a larger surface area to volume ratio.
3 Methods and Materials
3.1 Synthesis of Silica Nanocapsules
30 mg of Pluronic F127 (F127) was first dissolved in 900 µL of tetrahydrofuran (THF), followed by the addition of 40 µL of tetramethoxysilane (TMOS) to obtain a homogeneous mixture. The resulting solution mixture was then slowly injected into 10 mL of deionized water while stirred at 800 rpm, and left to stir for an additional 3 days at 300 rpm (For a schematic illustration of the synthesis protocol, refer to Appendix 1).
3.2 Synthesis of Silver Nanocapsules (AgNCs)
A templating strategy was explored in the synthesis of AgNCs, where the prepared hollow silica nanocapsules were encapsulated in metallic silver particles. Metallic silver particles were deposited onto the hollow silica nanocapsules templates via the Tollens reaction of silver mirroring. Herein, the silver complex [Ag(NH3)2]+ stock solution was first prepared by dissolving silver nitrate (200 mg) in deionized water (10 mL), to which ammonium hydroxide (400 µL) was added dropwise until the precipitate that formed redissolved. Next, the silica nanocapsules, glucose and silver complex [Ag(NH3)2]+ were mixed together and left to agitate. Finally, the conditions of the Tollens’ reaction were then optimized by varying the concentration and volume of the glucose solution, concentration of silica nanocapsules and the concentration of the silver complex [Ag(NH3)2]+ solution (Refer to Appendix 2 for details of the concentration variation).
3.3 Characterization Methods
Characterization of the AgNCs by UV-Vis spectroscopy was carried out to determine if silver has indeed formed on the surface of the silica nanocapsules. The AgNCs were then analyzed under the absorbance spectra in 400–4000 nm range with a Fourier Transform Infrared (FTIR) spectrophotometer to identity organic species in the AgNCs to confirm the silica still remained in the AgNCs. Dynamic light scattering (DLS) was used to determine the size of the nanocapsules as well as the critical micelle concentration. A scanning electron microscope (SEM) was used to determine the morphology of the AgNCs and the contents of the poly(vinyl alcohol) (PVA) film.Footnote 1 To test for the antibacterial properties of AgNCs, a culture of Pseudomonas aeruginosa ATCC 9027 strain was incubated with varying AgNC concentrations of 5, 10, 20 and 40% in respect to the cell medium. The culture was then left at room temperature for two days before quantifying for the bacteria growth in comparison to a bacteria culture containing no AgNCs (see footnote 1).
To test for the acid-responsive properties of AgNCs, the AgNC solution was first freeze-dried to obtain its dry powder form. Next, the AgNC powder was added to different types of acids at various pH. It was noted that 1 M nitric acid (HNO3) was able to completely breakdown AgNCs (dry powder form) into Ag+ ions as shown by the decolorization of the brown mixture into a clear solution (Refer to Appendix 3 for overnight results).
3.4 Preliminary Processing of Composite Antimicrobial Biomaterial Using PVA Film
Thin films incorporating AgNCs were created using 10% weight per volume (w/v) PVA. PVA powder of 4 different molecular weights were experimented: (i) 80% hydrolyzed, MW 9000–10,000, (ii) 87–89% hydrolyzed, MW 30,000–50,000, (iii) 99+% hydrolyzed, MW 1–30,000, and (iv) 99+% hydrolyzed, MW 85,000–124,000. For each type of PVA, 1 g of PVA was dissolved in 5 mL of deionized water by heating in an oven for 3 h at 95 °C. After cooling to room temperature, 5 mL of AgNC solution was added and left on an orbital shaker to be homogenized overnight. After homogenization, the mixture was spread over a petri dish to form a thin layer, after which it was placed in a freezer at −20 °C to freeze overnight. The samples were then taken out to thaw for 30 min, before freezing overnight again. The freeze-thaw cycle was repeated thrice.
To test if AgNCs can still release silver ions in acidic conditions while being embedded in PVA polymer matrix, the as-synthesized PVA films were cut into squares of size 2 cm × 2 cm, and then immersed into 10 mL of HNO3 with concentrations of 1, 0.5 and 0.1 M. The supernatants were then extracted and tested for silver ions using qualitative analysis. The composite antibacterial films were also subsequently observed for possible decolorization, which would indicate the conversion of metallic silver into silver ions, upon leaving to stand overnight.
3.5 Preliminary Processing of Composite Antimicrobial Biomaterial Using F127 Hydrogel
F127 is a FDA-approved thermoresponsive polymer with a sol-to-gel transition that can be triggered by the average body temperature of 37 °C. Hence it can be hopefully used as a gel to be applied on the body. 16%, 18%, 20% and 22 wt% F127 were prepared by weighing 0.8 g, 0.9 g, 1.0 g and 1.1 g of F127 respectively and topping up to 5 mL using deionized water, after which it was left in a fridge (4 °C) overnight for complete dissolution. To verify the sol-to-gel transition behavior of F127 hydrogel, the glass vial was inverted at both 25 and 37 °C. Visual inspection was then carried out to observe for any flow of liquid after being inverted.
Acid response to AgNCs-incorporated F127 hydrogel was tested by immersion in 1500 cm3 of 0.1 M HNO3 using a dialysis cassette of 10,000 MW cutoff, which would prevent the F127 hydrogel from passing through while allowing the Ag+ ions to diffuse out into the HNO3. Visual inspection of these hydrogels was then carried out to observe for color change to indicate the breakdown of AgNCs into Ag+ ions. At 12-h intervals over 60 h, small aliquots of the dialysis fluid were extracted to determine the released Ag content using Inductively Coupled Plasma Mass Spectrometry (ICP-MS), and the surrounding medium was also replaced by fresh HNO3. A control was also set up by replacing the HNO3 with distilled water under the same experimental conditions to prove that Ag+ ions were only released in acidic conditions.
4 Results and Discussion
4.1 Silica Nanocapsules Templates
The silica nanocapsule templates were monodisperse and on the nanoscale dimensions, with well-structured morphology (Fig. 1c). Its mechanical stability was attested by the DLS results, where the critical micelle concentration of the silica nanocapsules occurred at a higher dilution level than that of F127 (Fig. 1a, b). This showed that the silica nanocapsules were able to maintain their integrity and withstand high levels of dilution, thereby rendering it a good template to be adopted for the silver nanoparticle coating on it.
Characterization of F127 Micelles and Silica NCs. a Stability measurement of F127 micelle. b Stability measurement of silica NCs. c Transmission electron microscopy (TEM) image of silica NCs synthesized [13]
4.2 Optimization of the Synthesis Conditions of AgNCs
The optimum reaction conditions were determined from the brown color intensity of the supernatant obtained by the end of the reaction. The brown intensity gave an indication of the presence of metallic silver, which would be formed over the silica nanocapsule templates. Herein, the aim was to obtain a clear solution with a high brown color intensity, yet without the presence of any precipitation since it would otherwise indicate the severe aggregation of the silver nanoparticles.
Based on the solution color intensity obtained after varying the conditions of (i) glucose concentration (Fig. 2a); (ii) glucose amount (Fig. 2b); (iii) silica nanocapsule concentration (Fig. 2a); and (iv) silver complex concentration (Fig. 2c), the optimal combination was (i) 10 mg/mL glucose concentration, where (ii) 40 mg of glucose was dissolved in (iii) 3 mL silica nanocapsules, and (iv) 1 mL silver complex synthesized from 10 mg/mL silver nitrate.
Results of optimization of synthesis conditions of AgNCs after 2 days, best result(s) circled in red. a Variation of the concentration of silica nanocapsules and amount of glucose added, shown left to right, samples 1–9. b Variation in glucose concentration, shown left to right: g0–g5, last 2 bottles are controls with no glucose added and no silver complex added respectively. c Variation of silver complex solution shown from left to right, A1–A4, B1–B3
4.3 Properties of AgNCs
Formation of AgNCs was determined by UV-vis spectroscopy, where the surface plasmon absorbance maxima can be observed at around 420 nm from the UV-vis spectrum (Fig. 3a). This peak corresponds with what is expected of silver nanoparticles [7]. FTIR spectrum suggested that silica remained intact after its encapsulation with silver due to similar peaks (Fig. 3c). The AgNCs synthesized were uniform and spherical in shape, with a small particle size of around 100 nm (Fig. 3e). This was further supported by the DLS results, where the average hydrodynamic diameter of AgNCs was around 100 nm. DLS also showed a single peak, with little aggregation, which made the AgNCs ideal to be incorporated into polymers to form a homogeneous composite material (Fig. 3d). The as-synthesized AgNCs were also more stable than the silica nanocapsules as the former could withstand higher levels of dilution cycles before reaching its critical micelle concentration (Figs. 3b and 1b). This excellent colloidal stability could be attributed to the hydrophilic PEO chains of F127 in the AgNCs, which increased the water solubility and decreased non-specific protein adsorption.
The antibacterial property of AgNCs was measured by comparing bacteria growth in cell culture mediums with no AgNCs, as well as with AgNCs of various concentrations. Based on the results, it was evident that the AgNC had a dose-dependent antibacterial effect, albeit limited in its inhibition of the bacterial growth. Notably, it was only able to prevent 47.1% of bacteria growth after two day exposure to a 40% nanoparticle concentration (Fig. 3f). This was likely because the AgNCs had not been converted into free silver ions, which would otherwise have a stronger antibacterial effect [8]. Hence, this further justified the design a biomaterial that allowed a stimuli controlled release of Ag+ ions.
4.4 Incorporation of AgNCs into PVA Film to Form Composite Biomaterial
PVA was chosen as it is biodegradable, cheap to make and has low cytotoxicity, thus making it suitable for medical usage [9, 10]. It has also been widely used as a wound dressing due to its ability to provide a suitable environment for quick healing [11]. After 3 freeze-thaw cycles during the processing of PVA films, only PVA films synthesized using 99+% hydrolyzed PVA formed the films successfully (Refer to Appendix 4 for images of the films). Thus for the subsequent test for their acidic response behavior, only PVA films synthesized using 99+% hydrolyzed PVA, of MW 1–30,000 and MW 85,000–124,000 were used for experimentation.
The PVA films were observed to decolorize from brown to near colorless after immersing overnight in HNO3 for all 3 experimented concentrations (Fig. 4b). This indicated the successful conversion of AgNCs to Ag+ ions, stimulated by the acidic medium. Hence, HNO3 could be used as the basis for the design of antibacterial material that can respond to acidic conditions for a slow release of Ag+ ions for sterilization purposes.
However, qualitative analysis of the supernatant with sodium chloride yielded negative results as no white precipitate was observed, suggesting that instead of being dissociated into the surrounding aqueous acidic medium, the Ag+ ions were most probably trapped inside the PVA polymer matrix. Subsequent SEM analysis of the air-dried PVA films (Fig. 4a) confirmed that the AgNCs and any silver complex formed were indeed entrapped in the PVA polymer matrix.
This holds much potential in pharmaceutical applications. Since PVA films are known to have high water intake that allows them to absorb fluid from the wound site [11], the embedding of AgNCs in this polymer matrix kills bacteria and other microorganism that has been absorbed from the wound, preventing the growth of bacteria after being entrapped in the matrix. This keeps the wound sterile, thereby enhancing the property of PVA film as an ideal wound dressing.
4.5 Incorporation of AgNCs into F127 Hydrogel to Form Composite Biomaterial
F127 was chosen as it is a Food and Drug Administration (FDA) approved thermoresponsive polymer, which can be explored for various unique applications while being safe for medical use [11]. It is also regarded as an effective drug delivery material, thus making it ideal for medical usage [12]. As such, the incorporation of AgNCs into the F127 hydrogel would allow for a safe and effective delivery of the silver ions.
It was observed that the hydrogels formed using 18%, 20%, 22% and 24 wt% F127 could undergo the transition to solid hydrogel at room temperature (25 °C) (Refer to Appendix 5 for images of the hydrogels). Hence, these concentrations of F127 used were less ideal for bioapplication, as the intended sol-to-gel transition was to be induced at around 37 °C, the average body temperature. On the other hand, the hydrogel synthesized using F127 of 16 wt% turned to solid gel at 37 °C within 1–2 min, while transitioning reversibly back to liquid solution at 25 °C within 10 min (Refer to Appendix 5 for image of the hydrogel). This ensured that the F127 hydrogel acted as an effective reservoir of AgNCs that could be both stored and used conveniently.
However, after immersion into HNO3 maintained at 37 °C, the 16 wt% F127 gel reverted back to solution form, suggesting that F127 was unable to remain in gel form in extreme pH. In this regard, the F127 hydrogel could be treated as a lotion when exposed to extreme pH instead of a gel. From visual inspection (Fig. 5a), and analyzing the aliquots taken at regular 12-h intervals using ICP-MS, the F127 hydrogel was seen to be able to release silver ions at a slow, sustained rate (Fig. 5b), aligning with the goals of the project.
5 Results and Discussion
From the experiments conducted, it can be seen that the silver-silica nanocapsules were a stable antibacterial agent that could be easily incorporated into common polymers, to be further used in various applications. Its colloidal stability arose from the presence of hydrophilic PEO chains of F127 which enabled the AgNCs to have excellent antifouling behavior, and also avoided aggregation for a homogenous distribution within different materials. Explored applications include a thermoresponsive hydrogel or a bandage-like film, both of which were helpful in sterilization and to prevent bacteria growth. The incorporated AgNCs were also able to respond to an acidic stimuli within the materials chosen, thus allowing for a more controlled and sustained release of Ag+ ions for bactericidal purposes, greatly enhancing its antibacterial effects.
Due to time constraints, the PVA film and hydrogel were not tested with bacteria cultures under acidic conditions. Future work can involve testing for the effectiveness of the materials in preventing bacterial growth in actual bacterial cultures in an acidic medium.
Notes
- 1.
Done by supervisor.
References
Jung, W. K., et al. (2008). Antibacterial activity and mechanism of action of the silver ion in Staphylococcus aureus and Escherichia coli. Applied and Environment Microbiology, 74, 2171–2178.
The National Aeronautics and Space Administration (NASA). (2017). Water purification. https://ntrs.nasa.gov/archive/nasa/casi.ntrs.nasa.gov/20020083175.pdf. Accessed November 24, 2017.
Clement, J. L., & Jarret, P. S. (1994). Antibacterial silver. Metal-Based Drugs, 1, 467–482.
Drake, P. L., & Hazelwood, K. J. (2005). Exposure-related health effects of silver and silver compounds: A review. Annals of Occupational Hygiene, 49, 575–585.
Special Pathogens Laboratory. (2017). Acid producing bacteria. http://www.specialpathogenslab.com/acid-producing-bacteria.php. Accessed November 7, 2017.
Dobias, J., & Bernier-Latmani, R. (2013). Silver release from silver nanoparticles in natural waters. Environmental Science and Technology, 47, 4140–4146.
Saware, K., Sawle, B., Salimath, B., Jayanthi, K., & Abbaraju, V. (2014). Biosynthesis and characterisation of silver nanoparticles using Ficus benghalensis leaf extract. International Journal of Research in Engineering and Technology, 5, 867–874.
Jiraroj, D., Tungasmita, S., & Tungasmita, D. N. (2014). Silver ions and silver nanoparticles in zeolite A composites for antibacterial activity. Powder Technology, 264, 418–422.
Wang, L.-C., Chen, X.-G., Yu, L.-J., & Li, P.-W. (2007). Controlled drug release through carboxymethyl-chitosan/poly (vinyl alcohol) blend films. Polymer Engineering & Science, 47, 1373–1379.
Sharonova, A., et al. (2016) Synthesis of positively and negatively charged silver nanoparticles and their deposition on the surface of titanium. IOP Conference Series: Materials Science and Engineering, 116, 012009.
Oliveira, R. N., et al. (2014). Mechanical properties and in vitro characterization of polyvinyl alcohol-nano-silver hydrogel wound dressings. Interface Focus, 4, 20130049.
Nie, S., Hsiao, W. W., Pan, W., & Yang, Z. (2011). Thermoreversible Pluronic® F127-based hydrogel containing liposomes for the controlled delivery of paclitaxel: In vitro drug release, cell cytotoxicity, and uptake studies. International Journal of Nanomedicine, 6, 151–166.
Hsu, B. Y. W., et al. (2012). PEO surface-decorated silica nanocapsules and their application in in vivo imaging of zebrafish. RSC Advances, 2, 12392–12399.
Author information
Authors and Affiliations
Corresponding author
Editor information
Editors and Affiliations
Appendices
Appendices
1.1 Appendix 1: Synthesis of Silica Nanocapsules
See Fig. 6.
Diagram showing the formation of micelle and subsequently the silica nanocapsule [13]
1.2 Appendix 2: Variation of Reaction Conditions to Synthesize AgNCs
1.3 Appendix 3: Response of AgNCs to Acidic Conditions
See Fig. 7.
1.4 Appendix 4: Incorporation of AgNCs into PVA Film to Form Composite Material
See Fig. 8.
Results of the composite biomaterials formed from different types of PVA (shown left to right: (i) 80% hydrolyzed, MW 9000–10,000, (ii) 87–89% hydrolyzed, MW 30,000–50,000, (iii) 99+% hydrolyzed, MW 1–30,000, and (iv) 99+% hydrolyzed, MW 85,000–124,000), where only the latter two were formed successfully after 3 freeze-thaw cycles
1.5 Appendix 5: Sol-to-Gel Transition of F127 Hydrogel at Different wt%
Rights and permissions
Copyright information
© 2019 Springer Nature Singapore Pte Ltd.
About this paper
Cite this paper
Tan, Z.C.E., Zhang, C., Benedict, Y.W.H. (2019). Design of Smart Antimicrobial Materials Based on Silver-Silica Nanocapsules. In: Guo, H., Ren, H., Bandla, A. (eds) IRC-SET 2018. Springer, Singapore. https://doi.org/10.1007/978-981-32-9828-6_5
Download citation
DOI: https://doi.org/10.1007/978-981-32-9828-6_5
Published:
Publisher Name: Springer, Singapore
Print ISBN: 978-981-32-9827-9
Online ISBN: 978-981-32-9828-6
eBook Packages: Biomedical and Life SciencesBiomedical and Life Sciences (R0)