Abstract
The present work reports the experimental investigation of the phase change material (PCM) incorporated into the external wall claddings for achieving energy conservation in building through a passive cooling application. Three types of wall claddings of size 458 mm × 458 mm (1.5 ft × 1.5 ft) in dimension were developed in this experimental study. Lauric acid was utilized as the PCM to be incorporated into the wall claddings. Experimental results suggest that the lauric acid exhibited good latent heat potential, congruent phase change processes, and was thermally stable within operating temperature ranges. Furthermore, it was concluded that out of three cladding types being tested, the percentage drop of air temperature was more in composite wall cladding and the percentage drop of heat flux was more in aluminum box cladding with PCM and coarse aggregate. In total, the cladding incorporated with the PCM was found to be the potential candidate for the enhancement of energy efficiency in building through passive thermal storage and cooling load reduction.
Access provided by Autonomous University of Puebla. Download conference paper PDF
Similar content being viewed by others
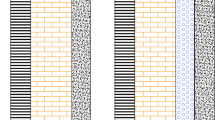
Keywords
1 Introduction
As the demand for comfort in buildings is increasing these days, energy consumption is also increasing substantially. Buildings are one of the major sectors which consume energy because of high heating and cooling load needs. Buildings account for 40% of global energy consumption as presented in Table 1 and contribute 40% of CO2 emissions. Residential sector consumes 27% of energy and contributes 17% of CO2 emissions [1].
According to Energy Information Administration, International Energy Outlook 2017 Reference case, the average annual energy consumption in buildings is expected to increase by 2.7% in India between 2015 and 2040 as depicted in Fig. 1.
For now, building’s energy demand is met by active systems to ensure indoor thermal comfort which leads to greenhouse gas emissions. In this era of energy crisis and environmental concerns, there is a great potential for the development of energy-saving and cost-effective technologies. One of the prominent technologies available in hand is the latent thermal energy storage (LTES), which uses the phase change material (PCM). The PCM while undergoing melting process would discharge the stored thermal energy when the surrounding temperature is high and would store the heat energy when the surrounding temperature is low and thereby, it solidifies. Melting and solidification occur at nearly isothermal conditions.
Most effective way of using LTES is by embedding PCM’s into the passive components of the buildings such as walls, windows, ceiling, floor, bricks, tiles, etc., [3,4,5,6,7,8,9,10]. This results in a reduction of heat transfer and indoor temperature fluctuations leading to reduction in energy consumption and CO2 emissions associated with heating and cooling [11,12,13,14,15]. Based on the literature review, there have been no studies reported so far on PCM being embedded in external wall claddings. This had driven the authors to perform this research study on different cladding materials suitable for external walls where PCM can be embedded for energy efficiency in buildings.
Cladding materials are the panels used to cover exterior or interior wall surfaces in order to protect the wall from environment and to ensure energy efficiency of the buildings [16]. There are diverse ranges of cladding materials available in the market which are evolving as an attractive option for commercial and residential sectors pertaining to their easy application, economical, and esthetic advantage. They are available in different shapes and sizes.
Claddings are needed to stop wind and rain from penetrating into walls, to provide acoustic and thermal insulation, to provide fire resistance, and to make the building opaque [17]. Halawa et al. [18] proposed two types of cladding materials namely opaque and glazed. While glazed ones are made up of transparent material, opaque ones include materials like stone, bricks, wood, metal, etc.
With a view to enhance the thermal comfort inside the building, the present investigation was targeted at developing an innovative cladding option and to check its efficacy with respect to its performance. Two cladding options, incorporated with PCM and one option without PCM, were being developed and were subjected to investigations with indigenously fabricated test setup to check the efficacy of the cladding options in providing the thermal comfort inside the building. The drop in temperature and heat flux due to the provision of cladding material was investigated with the test setup and the details of which are presented in the following sections.
2 Experimental Details
2.1 Materials and Methods
Three alternate wall cladding options were developed and tested during the current investigations. Details of each of these options are presented below.
2.1.1 Cement Concrete with Perlite as Cladding Option
As per the IS 10262-2009 guidelines on concrete mix proportioning, perlite concrete mix design process has been carried out with different proportions of mix ingredients. Target strength of M35 was chosen, suiting the cladding application. Final mix design was arrived at by optimizing with respect to the characteristic compressive strength of standard 15 cm × 15 cm × 15 cm cube specimens after 28 days of curing in water. The final mix design details are presented below for ready reference.
-
Cementitious and powder content: 548 kg/m3 with 50% cement, 45% GGBS, and 5% Perlite
-
Coarse aggregate: 480 kg/m3
-
Fine aggregate: 1120 kg/m3
-
Water/Cement ratio: 0.475
-
Polycarboxylate ether based sperplasticizer: 0.5% by weight of cement
-
Seven-day cube characteristic compressive strength: 26.81 MPa
-
28-day cube characteristic compressive strength: 45.57 MPa
With these optimized proportions, perlite concrete cladding blocks with dimensions of 458 mm (L) × 458 mm (B) × 12 mm (T) were prepared and cured for 28 days with a view to ensure the target strength of 35 MPa. A schematic of the block is presented in Fig. 2.
2.1.2 Aluminum Encased Loose Aggregate Plus PCM-Based Cladding Option
An aluminum sheet of 1.5 mm thickness was used to make an aluminum box cladding of 20.8 mm thickness with 17.8 mm hollow space in the interior of the box as depicted in Fig. 3. Lauric acid—C12H24O2—(PCM) and black granite coarse aggregate passing through 10 mm and retained on 6.3 mm sieve were filled into the hollow space of box as shown in Fig. 4.
The volume of aluminum box was found to be 4.3896 L. For every 1000 ml, volume of box 1.5 kg of coarse aggregate was filled based on the porosity calculations, 43% of volume of aluminum box is filled with lauric acid (PCM). After filling the materials into the box, the top side of the box was sealed by welding and checked for leakages.
2.1.3 Composite Wall Cladding Option
A composite wall cladding was developed by combining cement concrete with perlite (as discussed in Sect. 2.1.1) and aluminum encased loose aggregate plus PCM (as discussed in Sect. 2.1.2) and the same is depicted in the schematic presented in Fig. 5. The procedure of fabricating both the elements in this composite cladding is the same as that explained in the respective sections.
2.2 Characterization Techniques
Thermal properties such as the latent heat of fusion \((\Delta H_{\text{fusion}} )\) and melting onset temperature \((T_{\text{onset}} )\) were measured using the differential scanning calorimeter (DSC-60, SHIMADZU), wherein the PCM was sealed in aluminum pan and heated between 0 and 100 °C at a rate of 10 °C/min and cooled from 100 to 0 °C at the same rate. The experiment was carried out in the presence of a nitrogen gas atmosphere flowing at a rate of 50 mL/min. Thermogravimetric analysis (TGA) was performed in the range of ambient temperature to 500 °C in the presence of nitrogen stream to analyze the thermal stability of PCM.
2.3 Wooden Experimental Setup
A wooden setup of dimensions 1510 mm × 700 mm × 700 mm was developed to test the three types of claddings as described above. The wooden setup was partitioned into zone-1 and zone-2. Zone-1 contains six bulbs referring to the region or space “before cladding,” which would otherwise represent the exterior conditions of the test building as shown in Fig. 6. Zone-2 refers to the region or space “after cladding” which would otherwise represent the interior conditions of the test building. A slit of 70 mm was used to hold intact the cladding to be tested, which separates the zone-1 from zone-2. The gap present in between the cladding and wooden slit was filled with adequate insulation material as shown in Fig. 7 to prevent infiltration of air from zone-1 to zone-2 and any heat losses as well.
The bulbs in zone-1 represent the heat source to resemble the external environmental conditions. Six bulbs of 60 W, six bulbs of 100 W, six bulbs of 200 W were used in sets one after the other to test the three claddings for air temperature drop and heat flux drop. Two thermocouples as shown in Fig. 8 were placed 350 mm before cladding to measure the average air temperature in zone-1. Two thermocouples as shown in Fig. 9 were placed 350 mm after the cladding to measure the average air temperature in zone-2. Six thermocouples (three on each side) were placed on the cladding surfaces to monitor the average surface temperature.
Heat flux meter was placed in the wooden setup to measure the heat flux in zone-1 and in zone-2 as shown in Fig. 10. The cladding which has to be tested was placed in the slit and the top of the wooden box was closed as shown in Fig. 11. The entire test setup was connected to a data logger to measure the required temperatures.
3 Results and Discussions
Results obtained from the experiments being performed on the cladding materials are discussed below in terms of thermophysical properties of the PCM, percentage drop in the air temperature, and the heat flux after placing the cladding panels.
3.1 DSC Analysis for Thermal Energy Storage Properties of Lauric Acid
The DSC result pertaining to the melting and freezing curves of the lauric acid (PCM) is shown in Fig. 12. The phase change temperature and the latent heat enthalpy for the melting process were measured to be 42.7 °C and 186.58 kJ/kg, respectively, whereas for the freezing process, it was found to be 40.47 °C and 183.49 J/g, respectively. The absence of any solid-solid secondary peaks in the DSC results suggests that the lauric acid has undergone congruent phase transition processes [19,20,21]. These characteristics in turn were attributed to the good thermal storage properties which enabled the lauric acid to be possibly considered as a potential candidate for this exterior wall cladding application in test building.
3.2 TGA Analysis of Lauric Acid
The thermogravimetric analysis result as illustrated in Fig. 13 infers that the dominant mass loss of the lauric acid occurred through a continuous single-step process between 140 and 210 °C [22]. This mass loss was ascribed to the complete decomposition and evaporation of the organic compounds of the PCM. This analysis shows that the lauric acid was thermally stable up to 140 °C which was much on the higher side, while compared to the actual operating temperature range for the present exterior wall cladding application.
3.3 Percentage Drop in Air Temperature
The thermocouples connected to the data logger were used to measure the air temperature before and after the cladding regions for the three different claddings, which were tested as mentioned in Table 2. As the air temperature before the cladding was increased by using high wattage bulbs, drop in the temperature of the air after cladding was also increased [13].
The test results reveal that for the concrete with perlite cladding, the percentage drop of air temperature in “after-cladding region” when compared to the “before-cladding region” was accomplished to be 7.26%, 14.65%, and 17.64%, with 60 W, 100 W, and 200 W heat source, respectively. Likewise, for the aluminum box cladding, it was 3.20%, 11.02%, and 14.89% and for the composite wall cladding, it was 8.54%, 20.96%, and 20.76%, respectively.
Thus, the experimental measurements justified that when the composite wall cladding was utilized, the temperature drop of air was more on the building interior side. This clearly validated the heat storage effectiveness of the composite wall cladding material in terms of reducing the heat being transferred from the exterior (zone-1) to the indoor space (zone-2) of the test building.
3.4 Percentage Drop in Heat Flux
Heat flux meter was used to measure the heat flux in “before cladding” and “after cladding” regions for the as-developed three claddings as depicted in Table 3. For the concrete with perlite cladding, the percentage drop of the heat flux in the “after-cladding region” when compared to the “before-cladding region” was achieved to be 88.89%, 91.67%, and 97.17%, with 60 W, 100 W, and 200 W heat source, respectively. Likewise, for the aluminum box cladding, it was 98.61%, 98.61%, and 96.70% and for the composite wall cladding, it was 94.44%, 88.19%, and 94.81%, respectively. Hence, it was observed from the experimental results that the percentage drop in the heat flux was more when aluminum box cladding containing PCM and coarse aggregate was used.
4 Conclusions
In this research work, the phase change material (PCM) incorporated into the external wall claddings for achieving energy conservation in building through passive cooling application was experimentally investigated. In this study, one cladding material without PCM and the two other cladding materials with the PCM were developed, wherein the lauric acid was utilized as the PCM. In order for this PCM not to settle down at the bottom of the aluminum box, coarse aggregate was first filled in the aluminum box of second, third cladding and later melted PCM was poured into the box.
Based on the DSC test result, it was observed that the latent heat potential and the melting point of the PCM were found to be 186.58 J/g and 42.7 °C, respectively, which make the PCM suitable for this exterior wall cladding application in building. In addition, the TGA results also confirmed that the PCM was quite thermally stable within the operating temperature ranges. The as-developed claddings were then tested in a wooden experimental setup simulating the external and internal environmental conditions of a building.
The percentage drop in air temperature was found to be more in the composite wall cladding followed by concrete with perlite cladding and aluminum box cladding. Likewise, the percentage drop in the heat flux was found to be more in the case of the aluminum box cladding. Since there is a chance of aluminum box getting affected by corrosion when it is exposed to the environment, it is suggested to prefer composite wall cladding due to its ability to reduce the indoor air temperature and reduce heat flux as comparable to the aluminum box cladding.
In summary, the composite wall cladding incorporated with the PCM was found to be the potential candidate for the enhancement of energy efficiency through cooling load reduction and passive thermal storage in building. The feasibility and the energy-cost analysis for the as-developed claddings are essentially required in order to realize their utilization potential for the real-time application in buildings, and which are included into the scope of future work.
References
Soares N, Costa JJ, Gaspar AR, Santos P (2013) Review of passive PCM latent heat thermal energy storage systems towards buildings’ energy efficiency. Energy Build 59:82–103. https://doi.org/10.1016/j.enbuild.2012.12.042
Pérez-Lombard L, Ortiz J, Pout C (2008) A review on buildings energy consumption information. Energy Build 40:394–398. https://doi.org/10.1016/j.enbuild.2007.03.007
Guarino F, Athienitis A, Cellura M, Bastien D (2017) PCM thermal storage design in buildings: experimental studies and applications to solaria in cold climates. Appl Energy 185:95–106. https://doi.org/10.1016/j.apenergy.2016.10.046
Chen C, Guo H, Liu Y, Yue H, Wang C (2008) A new kind of phase change material (PCM) for energy-storing wallboard. Energy Build 40:882–890. https://doi.org/10.1016/j.enbuild.2007.07.002
Frederic K, Virgone J (2009) Experimental investigation of wallboard containing phase change material: data for validation of numerical modelling. Energy Build 41:561–570. https://doi.org/10.1016/j.enbuild.2008.11.022
Xu X, Zhang Y, Lin K, Di H, Yang R (2005) Modeling and simulation on the thermal performance of shape-stabilized phase change material floor used in passive solar buildings. Energy Build 37:1084–1091. https://doi.org/10.1016/j.enbuild.2004.12.016
Arkar C, Suzana D, Medved S (2018) Lightweight composite timber façade wall with improved thermal response. Sustain Cities Soc 38:325–332. https://doi.org/10.1016/j.scs.2018.01.011
Pasupathy A, Velraj R (2008) Effect of double layer phase change material in building roof for year round thermal management. Energy Build 40:193–203. https://doi.org/10.1016/j.enbuild.2007.02.016
Karthik P, Kumaresan V, Velraj R (2017) Passive cooling potential in buildings under various climatic conditions in India. Renew Sustain Energy Rev 78:1236–1252
Kant K, Shukla A, Sharma A (2017) Heat transfer studies of building brick containing phase change materials. Sol Energy 155:1233–1242
Ismail KAR, Castro JNC (1997) PCM thermal insulation in buildings. Int J Energy Res 21:1281–1296. https://doi.org/10.1002/(SICI)1099-114X(199711)21:14%3c1281
Lee KO, Medina MA, Raith E, Sun X (2015) Assessing the integration of a thin phase change material (PCM) layer in a residential building wall for heat transfer reduction and management. Appl Energy 137:699–706. https://doi.org/10.1016/j.apenergy.2014.09.003
Jin X, Medina MA, Zhang X (2014) On the placement of a phase change material thermal shield within the cavity of buildings walls for heat transfer rate reduction. Energy 73:780–786. https://doi.org/10.1016/j.energy.2014.06.079
Roth K, Westphalen D, Brodrick J (2007) PCM technology for building materials. ASHRAE J 49(7):129–131
Hasan MI, Basher HO, Shdhan AO (2018) Experimental investigation of phase change materials for insulation of residential buildings. Sustain Cities Soc 36:42–58. https://doi.org/10.1016/j.scs.2017.10.009
Latha PK, Darshana Y, Venugopal V (2015) Role of building material in thermal comfort in tropical climates—a review. J Build Eng 3:104–113. https://doi.org/10.1016/j.jobe.2015.06.003
Llorens J (ed) (2015) Fabric structures in architecture. Elsevier, Amsterdam
Halawa E, Ghaffarianhoseini A, Ghaffarianhoseini A, Trombley J, Hassan N, Baig M, Yusoff SY, Ismail MA (2018) A review on energy conscious designs of building façades in hot and humid climates: lessons for (and from) Kuala Lumpur and Darwin. Renew Sustain Energy Rev 82:2147–2161. https://doi.org/10.1016/j.rser.2017.08.061
Harish S, Orejon D, Takata Y, Kohno M (2015) Thermal conductivity enhancement of lauric acid phase change nanocomposite with graphene nanoplatelets. Appl Therm Eng 80:205–211. https://doi.org/10.1016/j.applthermaleng.2015.01.056
Jiesheng L, Yuanyuan Y, Xiang H (2016) Research on the preparation and properties of lauric acid/expanded perlite phase change materials. Energy Build 110:108–111. https://doi.org/10.1016/j.enbuild.2015.10.043
Suresh Kumar KR, Parameshwaran R, Kalaiselvam S (2017) Preparation and characterization of hybrid nanocomposite embedded organic methyl ester as phase change material. Sol Energy Mat Sol Cell 171:148–160. https://doi.org/10.1016/j.solmat.2017.06.031
Chen Z, Shan F, Cao L, Fang G (2012) Synthesis and thermal properties of shape-stabilized lauric acid/activated carbon composites as phase change materials for thermal energy storage. Sol Energy Mat Sol Cell 102:131–136. https://doi.org/10.1016/j.solmat.2012.03.013
Acknowledgements
The authors gratefully acknowledge DST, New Delhi, and BITS-Pilani, Hyderabad for providing financial support to carry out this research work under DST SERB Sanction No. ECR/2017/001146. The authors express their sincere thanks to the Editor and the anonymous reviewers for their constructive suggestions which helped to improve the manuscript. The authors also thank Mr. G. V. N. Trivedi and Mr. R. Naresh, BITS-Pilani, Hyderabad Campus for their support during experimentation.
Author information
Authors and Affiliations
Corresponding author
Editor information
Editors and Affiliations
Rights and permissions
Copyright information
© 2020 Springer Nature Singapore Pte Ltd.
About this paper
Cite this paper
Sravani, K., Parameshwaran, R., Vinayaka Ram, V. (2020). Experimental Study on PCM-Based External Wall Cladding for Energy Efficient Buildings. In: Kumar, H., Jain, P. (eds) Recent Advances in Mechanical Engineering. Lecture Notes in Mechanical Engineering. Springer, Singapore. https://doi.org/10.1007/978-981-15-1071-7_42
Download citation
DOI: https://doi.org/10.1007/978-981-15-1071-7_42
Published:
Publisher Name: Springer, Singapore
Print ISBN: 978-981-15-1070-0
Online ISBN: 978-981-15-1071-7
eBook Packages: EngineeringEngineering (R0)