Abstract
TiO2 nanofiber with different diameters was synthesized by calcining electrospun polyacrylonitrile (PAN) nanofibers embedded with titanium precursors. The TiO2 nanofibers was incorporated into sulfonated poly(ether ether ketone) (SPEEK) to preprare the proton exchange membrane (PEM). The incorporation of inorganic nanofiber can improve the performance of composite membrane. The conductivity of the SPEEK/TiO2-130 composite membrane is 1.15 times higher than that of the pristine SPEEK membrane at 100% relative humidity and 25 °C. In addition, the stable fiber skeleton suppresses the swelling coefficient of PEM. Compared to pristine SPEEK membrane, the SPEEK/TiO2 membranes exhibit higher comprehensive performance.
Access provided by CONRICYT-eBooks. Download conference paper PDF
Similar content being viewed by others
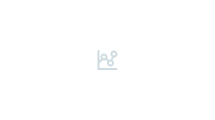
Keywords
Introduction
Direct methanol fuel cells (DMFCs) have attracted worldwide interest in the fields of automobiles, portable devices and residential power sources [1, 2]. Proton exchange membrane (PEM) is vital component which determine the performance and lifetime of DMFCs. The Nafion series membrane have high proton conductivity and excellent chemical stability but their utilization for DMFCs is hampered by the high cost. In the past two decades, many research groups have made continuing effort to find a ideal membrane material as substitute for Nafion. Sulfonated Poly(ether ether ketone) (SPEEK), a kind of low cost polymer material with excellent mechanical properties, exhibits great potential for DMFCs applications [3]. However, the conductivity of SPEEK is low owing to its narrower proton-conducting channels. This characteristic affect the overall DMFCs performance. It is worth noting that the incorporation of proton-conducting inorganic fillers for inter-connecting the proton-conducting channels within SPEEK is a facile and efficient approach to overcome this issue.
TiO2 nanofiber is one of the most advanced nano-material used as additive to the SPEEK matrix for membrane electrolyte [4]. But, there are problems associated with the preparation of TiO2 nanofiber. The conventional preparation methods of TiO2 nanofiber would use various hazardous chemicals. In this study, a single spinneret electrospinning technique was used to prepare TiO2 nanofiber [5]. The synthetic route is relatively simple, cost-effective and without any hazardous chemicals. We prepare a SPEEK/TiO2 nanofiber composite membrane and evaluate the membrane by investigating the water uptake (WU), dimensional stability, proton conductivity, and methanol permeability.
Experimental Section
Materials and Chemicals. PEEK powder was purchased from Victrex, Titanium (IV) oxide acetylacetonate (TiOacac) was purchased from Alfa Aesar. N,N-Dimethylformamide (DMF), dimethyl sulfoxide (DMSO) and H2SO4 (98%) were purchased from Beijing Chemical Reagent Factory.
-
Synthesis of SPEEK. 20 g of the PEEK was dissolved in 200 ml of concentrated H2SO4 preheated to 25 °C. The blended solution was heated to 45 °C and stirred for 3 h, then poured the sulfonated polymer solution into ice water to terminate the reaction. The polymer precipitate was washed with deionized water until the pH was neutral, then dried under vacuum at 80 °C for 24 h to get SPEEK.
-
Preparation of Inorganic Nanofiber. TiO2 nanofibers were prepared by a two-step process. Firstly, 0.5 g of TiOacac was dispersed into 3.0 g of DMF and 1.0 g of PAN was completely dissolved in 6.0 g of DMF under vigorous stirring. Then, mixed the solutions together and stirred until a homogenous solution was obtained. The single spinneret electrospinning technology was used to electrospun the blend solution. The PAN/TiOacac nanofiber with various diameters was prepared by changing electrospinning parameters. The light yellow color composite mat was obtained on a piece of aluminum foil. The TiO2 nanofibers were prepared by calcining electrospun composites mat in a muffle roaster. The composite mat was heated from 30 to 250 °C and thermally stabilized at 250 °C for 1 h and then heating to 550 °C and stabilized at this temperature for 5 h. The TiO2 nanofibers with various diameters (130, 160 and 220 nm) were fabricated, named as TiO2-130, TiO2-160 and TiO2-220, respectively.
-
Preparation and Characterization of The Blend Membranes. Various diameters of TiO2-130, TiO2-160 and TiO2-220 were impregnated in SPEEK ionomer to prepare SPEEK/TiO2 composite membrane by the following procedures. Certain weight of SPEEK and TiO2 nanofiber was dissolved in DMSO, then cast onto a glass dish and dried at 90 °C for 24 h under vacuum. The microstructures of the membranes were analyzed using a FEI F20 transmission electron microscope (TEM) equipped with energy dispersive X-ray spectroscopic analyzer (EDS) and a FEI Quanta 200F scanning electron microscope (SEM) operated at 30 kV.
-
Evaluation of Water Uptake (WU), Swelling Ratio (SR) and Proton Conductivity. The WUs and swelling rates of the membrane samples were determined by comparing the mass and dimensional changes of the membranes between the water-saturated and dry states, as reported in a previous study. The proton conductivity measurements were performed on a CHI660D electrochemical workstation. The membrane sample was soaked in deionized water at a certain temperature ranging from 30 to 60 °C, as reported in a previous study.
Results and Discussion
Characterization of TiO 2 Nanofiber. The PAN/TiOacac nanofiber with various diameters was prepared by changing electrospinning parameters (such as the distance of needle to the collector, diameter of the needle and charge density). The morphology of the prepared TiO2-128 nanofiber was observed by SEM and shown in Fig. 1. The surface of nanofibers are smooth without beaded. Furthermore, F1b shows the enlarged morphology, the result represents that the nanofibers are compact without void space.
The TEM (Fig. 2a–c) image illustrates the connections of the small particles forming the fiber structure. This structure is stable because the strong adsorption of particles.
STEM elemental mapping analysis of TiO2 nanofiber was detected and shown in Fig. 2d–f. The elemental Ti (yellow color points in Fig. 2e) and O (blue color points in Fig. 2f) are clearly observed in the nanofibers. The element mapping photos exhibit the uniform dispersion of all elements, indicating the precursor are dispersible well in the fibers.
-
Characterization of Membranes. The cross-section morphology of plain SPEEK and hybrid membranes are shown in Fig. 3. The nanofibers are evenly dispersed in the composite membranes without phase separation or defects when the content of TiO2 nanofibers is less than 1.0 wt%. Furthermore, TiO2 nanofibers keep fiber structure without obvious destruction in the inner part of the composite membranes. This result proves that SPEEK does not affect the shape and status of the nanofibers. However, when nanofiber content is increased up to 1.5 wt%, the heterogeneous morphology and defects be found due to the stacking and aggregation of nanofibers.
The elemental mappings were utilized to further detect the dispersion of TiO2 nanofiber in composite membrane. Figure 4 shows Ti, S and O elements in the SPEEK/TiO2 composite membrane. The characteristic elements distribute evenly and continuously on the cross-section of the membrane. In summary, the dense and homogeneous composite membranes can be successfully prepared.
-
WU, SR and Proton Conductivity. The WU and SR of all samples were measured at 25 and 50 °C (Fig. 5). Compare to pristine SPEEK, all SPEEK/TiO2 membrane exhibits higher WU. This may be contribute to hydrophilic TiO2 make hydrophilicity of membranes increase. With the decrease of TiO2 diameter, the WU of membranes increases from 7.4 wt% in SPEEK/TiO2-220 to 8.2 wt% in SPEEK/TiO2-130 membrane at 25 °C. This may be due to the hydrophilicity of TiO2 nanofiber decrease with the increasing nanofiber diameter.
The DR of the membrane also plays an important role in influencing the dimensional stability of PEM [6]. The tendency of DR is substantially in accordance with that of WU. But, the increment decreases after the nanofibers are incorporated. Because the stable fiber skeleton improve the dimensional stability of the composite membrane.
Compare to pristine SPEEK membrane (σ = 60.9 mS/cm, 25 °C), optimal proton conductivity of 69.8 mS/cm−1 is obtained on SPEEK/TiO2-130. Hydrophilic TiO2 promotes the membrane to absorb more water which facilitates proton transfer via “vehicle” mechanism. Moreover, TiO2 nanofiber interconnect the ionic clusters and subtle regions in the SPEEK matrix, creating continuous proton transfer network to improve conductivity.
Conclusions
In this work, the various diameter of TiO2 nanofibers were successfully prepared and incorporated into SPEEK to prepare SPEEK/TiO2 composite membranes. The performances of the composite membranes are compared in terms of the diameter of incorporated nanofiber. The SPEEK/TiO2-130 membrane has the highest conductivity of 69.8 mS/cm. All SPEEK/TiO2 composite membranes show higher proton conductivities and better dimensional stability than the pure SPEEK membranes.
References
S.D. Bhat, A.K. Sahu, A. Jalajakshi, S. Pitchumani, P. Sridhar, C. George, PVA-SSA-HPA mixed-matrix-membrane electrolytes for DMFCs. J. Electrochem. Soc. 157, B1403–B1412 (2010)
C.-C. Yang, Y.J. Li, T.-H. Liou, Preparation of novel poly(vinyl alcohol)/SiO2 nanocomposite membranes by a sol–gel process and their application on alkaline DMFCs. Desalination 276, 366–372 (2011)
J.-C. Tsai, H.-P. Cheng, J.-F. Kuo, Y.-H. Huang, C.-Y. Chen, Blended Nafion®/SPEEK direct methanol fuel cell membranes for reduced methanol permeability. J. Power Sources 189, 958–965 (2009)
Y. Jun, H. Zarrin, M. Fowler, Z. Chen, Functionalized titania nanotube composite membranes for high temperature proton exchange membrane fuel cells. Int. J. Hydrogen Energy 36, 6073–6081 (2011)
K. Ketpang, B. Son, D. Lee, S. Shanmugam, Porous zirconium oxide nanotube modified Nafion composite membrane for polymer electrolyte membrane fuel cells operated under dry conditions. J. Membr. Sci. 488, 154–165 (2015)
S. Zhong, C. Sun, Y. Gao, X. Cui, Preparation and characterization of polymer electrolyte membranes based on silicon-containing core-shell structured nanocomposite latex particles. J. Power Sources 289, 34–40 (2015)
Acknowledgements
This study was supported financially by the National Key Research and development Plan (Grant no: 2016YFC0303700); National Science and Technology Major Project (Research Index: 201505017-002).
Author information
Authors and Affiliations
Corresponding author
Editor information
Editors and Affiliations
Rights and permissions
Copyright information
© 2018 Springer Nature Singapore Pte Ltd.
About this paper
Cite this paper
Dong, C., Hao, Z., Zhou, Q. (2018). Enhancement of Proton Conductivity of Polymer Electrolyte Membrane Enabled by Electrospun Nanofibers. In: Han, Y. (eds) Advances in Energy and Environmental Materials. CMC 2017. Springer Proceedings in Energy. Springer, Singapore. https://doi.org/10.1007/978-981-13-0158-2_1
Download citation
DOI: https://doi.org/10.1007/978-981-13-0158-2_1
Published:
Publisher Name: Springer, Singapore
Print ISBN: 978-981-13-0157-5
Online ISBN: 978-981-13-0158-2
eBook Packages: EnergyEnergy (R0)