Abstract
Sterility mosaic disease (SMD) of pigeonpea (Cajanus cajan (L.) Millsp) first reported in 1931, is an economically most important viral disease, which is endemic to India, Nepal, Bangladesh and Myanmar. SMD was long suspected to be a viral disease, however its causal agent, pigeonpea sterility mosaic virus (PPSMV) was discovered only in 2000. In 2013, the full genome of PPSMV was sequenced and based on the genome organization the virus was assigned to the genus Emaravirus. In 2015, association of another distinct emaravirus in SMD affected pigeonpea was discovered and it was named as pigeonpea sterility mosaic virus 2. As per the latest ICTV classification, these two pigeonpea infecting emaraviruses are renamed and recognised as two different virus species, Pigeonpea sterility mosaic emaravirus 1 and Pigeonpea sterility mosaic emaravirus 2 under the family Fimoviridae of the order Bunyavirales. These two emaraviruses involved in SMD are transmitted in a semi-persistent manner by an eriophyid mite, Aceria cajani Channabassavanna (Acari: Arthropoda). These viruses and its eriophyid vector are highly specific to pigeonpea and its wild relatives. This chapter presents the review of the studies conducted on SMD of pigeonpea and PPSMVs in India.
Access provided by CONRICYT-eBooks. Download chapter PDF
Similar content being viewed by others
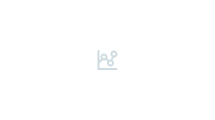
Keywords
1 Introduction
Sterility mosaic disease (SMD) of pigeonpea (Cajanus cajan (L.) Millsp) is caused by two distinct emaraviruses, pigeonpea sterility mosaic virus 1 (PPSMV-1) and pigeonpea sterility mosaic virus 2 (PPSMV-2) (Elbeaino et al. 2014, 2015; Patil and Kumar 2015). However, as per the latest ratification of emaravirus species by the International Committee on Taxonomy of Viruses (ICTV), these two pigeonpea infecting emaravirus species are named as Pigeonpea sterility mosaic emaravirus 1 and Pigeonpea sterility mosaic emaravirus 2. SMD, first reported in 1931 from Pusa in Bihar, is endemic to India, and its neighboring countries such as Bangladesh, Nepal and Myanmar, and is a major constraint for cultivation of pigeonpea resulting in an economic loss of ~300 million US$ in India (Mitra 1931; Nene 1995; Patil and Kumar 2015; Reddy et al. 1998). The nature of symptoms involved in SMD and the extent of yield losses is a manifestation of the pigeonpea genotype, environmental factors and the time of virus infection. Early virus infection (<45 days) can result in more than 95% yield loss, whereas late infections can lead to 26–97% yield losses (Kannaiyan et al. 1984). When the pigeonpea plants are infected by PPSMV during flowering period, the plants show excessive vegetative growth, with no/poor flowering and pod set and under congenial conditions the disease spreads like a plague and hence it is also referred as “Green Plague”. The SMD affected pigeonpea plants are also vulnerable to fungal diseases and infestation by spider mites. The characteristic symptoms of SMD are partial to complete cessation of flowering (sterility), enhanced vegetative growth, chlorotic rings or mosaic symptoms on the leaves and a reduction in their size, and stunting (Fig. 10.1, Jones et al. 2004). However the type of pigeonpea cultivar and the time of virus infection largely manifest the symptoms or its severity.
The viruses involved in SMD are transmitted by an eriophyid mite, Aceria cajani Channabassavanna (Acari: Arthropoda) but not through seed (Kulkarni et al. 2002; Kumar et al. 2000, 2002b, 2003). Both the virus and its vector are highly host specific and restricted to pigeonpea and some of its wild relatives (Kumar et al. 2008). In the past, multi-location field trials of different pigeonpea genotypes across India had indicated the presence of PPSMV variants (Kumar et al. 2008; Reddy et al. 1993). Some of the studies on PPSMV isolate from Coimbatore (Tamil Nadu state) and Bengaluru (Karnataka state) had shown cytopathological differences and also variation in the size of their nucleocapsid proteins (Kumar et al. 2000, 2003; Patil and Kumar 2015).
After the first report of SMD from India in 1931, it took nearly 85 years to unravel the complete sequence information of the PPSMV genome (Fig. 10.2; Mitra 1931; Elbeaino et al. 2014). Based on the genome organization and sequence information, PPSMV has been included in the genus Emaravirus and family Fimoviridae, in the order Bunyavirales (Mielke and Muehlbach 2007; Mühlbach and Mielke-Ehret 2011). The genus Emaravirus is one of the youngest and emerging genera of plant viruses with a negative sense segmented RNA genome, with four to eight RNA segments depending on the emaraviral species (Di Bello et al. 2015; Mielke-Ehret and Muhlbach 2012).
2 Vector, Virus Transmission and Host Range
PPSMVs involved in SMD are semi-persistently transmitted by the mite vector Aceria cajani (Channabasavanna) (Arthropoda: Acari: Eriophyidae) (Channa basavanna 1981; Kumar et al. 2001; Kulkarni et al. 2002). These eriophyid mite vectors are known to transmit all the emaravirus species and also tritimoviruses of Potyviridae family (Kumar et al. 2001). The eriophyid mites are microscopic arthropods which disperse by the wind currents. During the active stages of their life cycle, these eriophyid mites are obligate pests which infest large number of plant species, resulting in severe economic losses (Oldfield and Proeseler 1996). These mites are almost exclusively found on the symptomatic leaves of SMD affected plants, which inhabit on the lower surface of leaves; however mite feeding causes no visible damage. Studies by Kulkarni et al. (2002) demonstrated that the transmission efficiency of a single eriophyid mite (A. cajani) can be up to 53% and were able to accomplish 100% transmission when more than five mites were used. To acquire the virus, these eriophyid mites required a minimum acquisition access period of 15 min and minimum inoculation access period of 90 min for transmission of the virus and there was no need of any latent period (Kulkarni et al. 2002). Although PPSMV stays within the mites for about 6–13 h, PPSMV replication within the mite and transovarial transmission has not been found (Kulkarni et al. 2002). There was a significant increase in the proliferation of A. cajani in PPSMV infected pigeonpea plants, when compared to the healthy plants suggesting for a beneficial relationship between the virus and its vector (Reddy and Nene 1980). Due to the presence of a smaller sized stylet the eriophyid mites feed on the mesophyll epidermal cells or its adjacent layers on the lower-surface of the leaves and this also affects the transmission efficiency of PPSMV. It is difficult to study the eriophyid mites on the experimental host plants from Chenopodiaceae, Solanaceae and Cucurbitaceae, because of its stringent host specificity and without feeding on pigeonpea the mites can survive only for ~13 h (Kulkarni et al. 2002). The eriophyid mites can proliferate to high densities in a week’s time, once they are established on a susceptible pigeonpea genotype. These mites disperse passively, which is facilitated by the wind currents and the relative humidity has a profound impact on the population of mites (Kaushik et al. 2013; Singh and Rathi 1997). A temperature range of about 20–30 °C was found to be favourable for the multiplication of the eriophyid mites, whereas higher temperatures and heavy rains were not favourable. Sequence analysis of ITS region of rDNA did not reveal any significant variation among the populations of A. cajani, collected from diverse SMD endemic locations of India, Myanmar and Nepal, and this correlated with conservation of the morphological features of the eriophyid mites (Kumar et al. 2001; Latha and Doraiswamy 2008). These studies probably indicate that there are no biotypes of A. cajani and they don’t differ much in their ability to transmit PPSMV.
Some success has been obtained in transmission of PPSMV to experimental hosts, such as Nicotiana benthamiana, Nicotiana clevelandii, Chrozophora rottleri and Phaseolus vulgaris by sap inoculation, but the efficiency has been low (Kulkarni et al. 2003b; Kumar et al. 2002b, 2003). However, attempts to sap-transmit to the natural host, pigeonpea, have failed and the purified PPSMV preparations were also not infectious (Kumar et al. 2003). However, PPSMV could be experimentally transmitted to pigeonpea by grafting (Ghanekar et al. 1992; Kumar et al. 2002a, b). In natural conditions only a few wild species of Cajanus could support the infestation by the eriophyid mite vector (Kumar et al. 2007). Seed or pollen transmission has not been reported for any emaraviruses, including PPSMV (Divya et al. 2005; Mielke-Ehret and Muhlbach 2012), since the virus has been detected in seed coat alone, but not in the cotyledons. Leaf stapling, infector hedge and spreader row inoculation are the three widely used experimental methods for transmission of PPSMV (Nene and Reddy 1976a, b). The leaf stapling method is most commonly used for evaluation in pots and field, whereas for field screening both the infector hedge and spreader row methods are used.
3 Epidemiology of SMD
SMD is an endemic disease in most of the pigeonpea cultivating areas of India; however its incidence varies widely from one region to another and from one season to the other (Kumar et al. 2008). SMD is reported from the states of Karnataka, Andhra Pradesh, Telangana, Bihar, Maharashtra, Tamil Nadu, Chhattisgarh, Gujarat, Punjab, Uttar Pradesh and West Bengal (Kannaiyan et al. 1984; Narayana et al. 2000; Singh and Raghuraman 2011; Zote et al. 1991). Since PPSMV is not a seed transmitted virus, SMD is mostly introduced into the new fields through the viruliferous mites which pick up the virus either from the perennial pigeonpea or the volunteer plants. Hence, the incidence of SMD mainly depends on the vicinity of the new fields to the source of inoculum, weather conditions, susceptibility of pigeonpea genotype and the population of eriophyid mites.
Generally the irrigated pigeonpea are more vulnerable to early infection by PPSMV than the rain-fed pigeonpea, as it may provide a favourable environment to the vector mites (Dharmaraj et al. 2004). However the effect of weather parameters on the epidemiology of SMD is not well established and there are varying interpretations. Some of the potential sources of primary virus inoculum are the infected pigeonpea plants remaining in the farms after the harvest, and also the perennial pigeonpea and their wild relatives (Kumar et al. 2008; Narayana et al. 2000). Whereas, in rain-fed agriculture, the pigeonpea stubble left-over in the field after harvest, and the plants thriving around the water bodies, may have sufficient amount of green foliage to harbour the eriophyid mites (Dharmaraj et al. 2004). The left-over pigeonpea stubbles that sprout back to accumulate green foliage, particularly after the summer rains, serves as a primary source of virus inoculum, providing favourable conditions for multiple cycles of virus infection. However because of the vast diversity in the cropping patterns and seasons in India it is hard to recognize the primary sources of SMD inoculum (Patil and Kumar 2015).
4 Taxonomy, Genome Organization and Gene Functions
In 2000, a breakthrough was made in diagnosing the causal agent of SMD as a virus with a negative (-ve) strand RNA virus (Kumar et al. 2000). Electron microscopic studies of the virus particles indicated it to be of 8–11 nm in diameter, with undetermined length and showing characteristic features of both the genera Tenuivirus (Family: Phenuiviridae) and Orthotospovirus (Family: Tospoviridae) (Kumar et al. 2002a, b, 2003). Both orthotospoviruses and tenuiviruses are negative strand RNA viruses, which are the only plant infecting genera of the order Bunyavirales, majority of which infect animals (Kormelink et al. 2011). The viruses of the order Bunyavirales are classified into six genera, all of which are transmitted by the arthropod vectors. The virus particles (80–120 nm) are mostly bound by a double membrane, with a tripartite genome. The presence of envelop membrane is unique to orthotospoviruses, emaraviruses, and rhabdoviruses. The crux of the virus particles encompass the ribonucleoproteins which is essentially the viral genomic RNA rigidly associated with the nucleocapsid protein (NP), along with traces of viral RNA-dependent RNA polymerase (RdRp).
Electron micrographs of SMD affected pigeonpea and N. benthamiana plants revealed the presence of 100–150 nm DMBs and fibrous inclusions of varying sizes located next to the nucleus (Kumar et al. 2002a, b). The location of these emaravirus DMBs near the endoplasmic reticulum (ER) and Golgi cisterns suggesting that the these organelles are the sites of emaravirus particle morphogenesis, as reported for tospoviruses (Kikkert et al. 1999; Ishikawa et al. 2015). All the emaraviruses have segmented negative sense RNA genomes and are transmitted by the eriophyid mites. The European mountain ash ringspot-associated emaravirus (EMARaV) is the type species of this genus Emaravirus, consisting of four RNA segments (Mielke and Muehlbach 2007). The other species of the genus Emaravirus are Actinidia chlorotic ringspot-associated emaravirus (AcCRaV), Fig mosaic emaravirus (FMV), Rose rosette emaravirus (RRV), Redbud yellow ringspot associated emaravirus (RYRSaV), Raspberry leaf blotch emaravirus (RLBV), High plains wheat mosaic emaravirus (HPWMoV) and Blackberry leaf mottle-associated emaravirus (BLMaV) (Table 10.1) (Di Bello et al. 2015, 2016; Hassan et al. 2017; ICTV 2016; Laney et al. 2011; Mielke-Ehret and Muhlbach 2012; Mühlbach and Mielke-Ehret 2011; Patil and Kumar 2015; Tatineni et al. 2014; Zheng et al. 2017). Each RNA segment of the emaraviral genome typically contains only one open reading frame (ORF) encoding for a polymerase, glycoprotein, nucleocapsid, movement protein, and other proteins whose function is yet to be understood.
The first published sequence of pigeonpea sterility mosaic virus; later renamed as pigeonpea sterility mosaic virus 1 (PPSMV-1) was shown to contain five genomic RNA segments of varying sizes (Elbeaino et al. 2014, 2015). The largest of all is referred as RNA1, with 7022 nucleotides length, coding for RNA-dependent RNA polymerase (RdRp, 2295 amino acids); the other four segments are referred as RNA2 (2223 nt) coding for glycoprotein (GP, 649 amino acids); RNA3 (1442 nt) coding for nucleocapsid protein (NP, 309 amino acids); RNA4 (1563 nt) coding for a putative movement protein p4 (MP, 362 amino acids); and RNA5 (1689 nt) coding for p5 (474 amino acids), a protein with unknown function (Elbeaino et al. 2014). Following the first report of PPSMV-1 genome sequence, another novel emaravirus Pigeonpea sterility mosaic virus 2 (PPSMV-2) was also reported to be associated with the SMD of pigeonpea (Elbeaino et al. 2015). PPSMV-2 has a higher sequence similarity with FMV than the first published sequence of PPSMV-1 (Elbeaino et al. 2014, 2015). Subsequently, the first published PPSMV sequence was renamed as Pigeonpea sterility mosaic virus 1 (PPSMV-1) (Elbeaino et al. 2015), which was further ratified as Pigeonpea sterility mosaic emaravirus 1 (ICTV 2016). The sequence reports by Elbeaino et al. (2014, 2015) showed that the PPSMV-1 had only five segments, whereas the PPSMV-2 was associated with an additional RNA segment referred as RNA6, with a length of 1194 nt, coding for 27 kDa protein p6, with unknown function. The six RNA segments RNA1 to RNA6 of PPSMV-2 are of the size: 7009, 2229, 1335, 1491, 1833 and 1194 nucleotides, respectively (Patil et al. 2017).
All the six RNA segments of PPSMV-1 and PPSMV-2 show maximum sequence identity with the corresponding RNA segments of FMV and RRV (Fig. 10.4). The single ORF of the segment RNA1 of both the PPSMVs encode for RNA dependent RNA polymerase (RdRp), consisting of 2294 amino acids (aa) that accounts for a molecular mass of 267.9 kDa (Fig. 10.3). The RdRp has all the conserved motifs that are common to members of the Fimoviridae. The RdRp of PPSMV-1 and PPSMV-2 have amino acid (aa) sequence similarities in the range of 37–54% and 30.9–72.1% respectively, with the RdRp of other emaraviruses (RRV, FMV, RYRV, EMARaV, RLBV). The active site of the RdRp encoded by both the PPSMV-1 and PPSMV-2 has a core polymerase module along with the five conserved motifs A-E (Fig. 10.3). Of the five conserved RdRp motifs the two motifs that are involved in divalent-metal cation binding are: motif A (DASKWS, 1125–1130) and C (SDD, 1183–1185) and these are also parts of the palm domain of the replicase protein (Bruenn 2003). The motif B (QGNLNHLSS, 1210–1218) has the role of RNA binding, whereas the motif D (KK, 1276–1277) with a tertiary structure shows catalytic activity. The cap-snatching activity characteristic of the members of order Bunyavirales is present in the motif E (EFLST, 1312–1316) (Duijsings et al. 2001). Cap-snatching is a gene expression strategy of several RNA viruses, wherein the endonuclease property of RdRp helps in the cleavage of the capped-RNA leader sequences of host mRNA, which is eventually used to initiate viral transcription (Kormelink et al. 2011).
Schematic representation of the genome organization of the six PPSMV-1 and/or PPSMV-2 RNA segments (linear lines) and the open reading frames (ORFs) of each RNA segment are indicated (pink boxes). Expression products of ORFs of each RNA segment (p1, p2, p3, p4 and p5) and their estimated molecular weights (kDa) are indicated. Letters (a–f) represent the conserved motifs on the RdRp protein encoded by RNA-1; Gn and Gc indicate the N- and C-terminal of the glycoprotein of RNA-2; and N and C are N-terminal and C-terminal ends of proteins, respectively, nt, nucleotides (Modified from: Patil and Kumar 2015; Elbeaino et al. 2014, 2015)
Phylogenetic trees drawn with predicted amino acid sequences of RNA-dependent RNA polymerase (RdRp), glycoprotein precursor (Gp), nucleocapsid protein (NP) and movement protein (MP) of PPSMV-1 and PPSMV-2 isolates from Patancheru (Greater Hyderabad) with the orthologues of members of the genus Emaravirus, Tenuivirus, Cytorhabdovirus, Dichorrhabdovirus and Orthotospovirus. The accession numbers for each emaravirus isolates are given in the parenthesis. The analysis was carried out using the neighbour-joining algorithm and the Poisson-model in MEGA6, using 1000 bootstrap replicates. Bootstrap values are given on each node of each branch and the scale bar represents 0.2 substitutions per amino acid position. Tomato spotted wilt virus (TSWV) and Groundnut bud necrosis virus (GBNV) are orthotospoviruses; Rice stripe virus (RSV) and Rice hojablanca virus (RHBV) are tenuiviruses. The two Dichorhabdoviruses (unassigned negative-sense, ssRNA viruses), Coffee ringspot virus (CoRSV) and Orchid Fleck Virus (OFV); and a Cytorhabdovirus (Rhabdoviridae family) Lettuce necrotic yellow virus (LNYV) are used as members of out-group (Modified from: Patil and Kumar 2015)
The segment RNA2 of both PPSMV-1 and PPSMV-2 encode for glycoprotein precursors (GP) of 648 aa. The PPSMV-1 and PPSMV-2 GP share a sequence identity of 31–45% and 21.3–57.1%, respectively with the glycoprotein precursors of other emaraviruses. The glycoproteins form spike-like projections on the envelop membrane of emaraviruses and show the presence of three putative transmembrane helices and four putative glycosylation sites. Within the golgi complex, the precursor glycoprotein is cleaved into two functional glycoproteins: Gn (22.5 kDa) and Gc (51.8 kDa) at a predicted cleavage site. The PPSMV-1 RNA3 codes for a 34.6 kDa nucleocapsid (NP) protein, with aa identities comparable to NPs of FMV (44%), RRV (43%), EMARaV (35%), RYRSV (37%) and RLBV (27%). Whereas the PPSMV-2 encoded nucleocapsid protein shows highest sequence identity with FMV (Elbeaino et al. 2015). The three conserved amino acid motifs, NVLSFNK (134–140), NRLA (183–186) and GYEF (204–207) present within the nucleocapsid protein is thought to be involved in RNA-binding (Elbeaino et al. 2014, 2015). The RNA4 segment of PPSMV-1 encodes for a 40.8 kDa protein “p4”, with sequence homology ranging from 24% to 41% with p4 of other emaraviruses. Whereas the PPSMV-2 p4 shares a higher sequence identity of 75% and 61.2% with FMV and RRV encoded p4, respectively. The amino acid analysis of p4 protein indicates it to be involved in cell-to-cell movement of the virus, similar to the movement proteins (MP) encoded by RNA4 of RRV and RLBV (Yu et al. 2013; McGavin et al. 2012). The movement protein modifies the plasmodesmata interconnecting the plant cells to allow movement of viral genomes from one cell to the other, followed by their systemic transport through the plant vasculature. It may also be possible that P4 functions as a suppressor of gene silencing.
The RNA5 of both PPSMV-1 and PPSMV-2 encodes for a 473 aa long and 55 kDa protein “p5”. The PPSMV-1 encoded p5 shares a sequence identity of 33% with p5 of FMV, while the PPSMV-2 encoded p5 had a higher sequence identity with PPSMV-1 (61.1%), rather than FMV (43.1%). When the p5 encoded by RLBV was fused with the reporter gene green fluorescent protein, the fusion protein was localized as aggregated structures within the cytoplasm; however its function is yet to be understood (McGavin et al. 2012). The segment RNA6 of both PPSMV-1 and PPSMV-2 encodes for a 238 aa protein “p6” with a molecular mass of 27 kDa and with an unknown function (Elbeaino et al. 2015; Patil et al. 2017). The RNA6 and the p6 of both PPSMV-1 and PPSMV-2 show more than 96% sequence identity, while they share only 23.9% sequence identity with FMV (Elbeaino et al. 2015; Patil et al. 2017). The 5′ and 3′ termini of all the six RNA segments of both PPSMV-1 and PPSMV-2 possess untranslated regions (UTRs). The first 13 nts at both 5′ and 3′ termini of each emaravirus RNA segment highly conserved and complimentary, except for the two nucleotides at position 8 and 9 (U8-U9), which results in the formation of a panhandle structure, giving a pseudo-circular appearance to the ribonucleoprotein (RNPs). The terminal sequences of the genomic RNA segments are conserved among all the members of the same genus, but they differ among different genera (Elliott and Blakqori 2011).
Sequence variability studies among 23 isolates of PPSMV-1 and PPSMV-2, collected from ten different locations representing six states of India, showed that the isolates of both PPSMV-1 and PPSMV-2 are present across India and also occur as mixed infections (Patil et al. 2017). Detailed sequence analysis indicated the presence of recombination and reassortment among the corresponding RNA segments of both PPSMV-1 and PPSMV-2 isolates (Patil et al. 2017). The segment reassortments mostly involved the swapping of RNA4 segment among PPSMV-1 and PPSMV-2 (Patil et al. 2017). These studies also revealed that the sixth RNA segment (RNA6) previously reported to be associated with PPSMV-2 alone is also associated with the isolates of PPSMV-1 (Patil et al. 2017).
5 Diagnostics for PPSMV
Historically SMD diagnosis was based on characteristic mosaic symptoms (Fig. 10.1). However, virus isolation and characterization has paved for the development of serological and nucleic acid-based diagnostic methods. The first diagnostic test was developed using the polyclonal antibodies against the purified ribonuceloprotein particles of PPSMV (Kumar et al. 2003). These polyclonal antibodies were successfully employed for detection of PPSMV by the double antibody sandwich (DAS) and direct antigen coating forms of ELISA and Western immunoblotting (Kumar et al. 2002a, b, 2003). Using the same antibodies direct-immunobinding assay (DIBA) was developed to detect PPSMV in the viruliferous eriophyid mites (Latha and Doraiswamy 2008). Both DAS-ELISA and DIBA were sensitive for detection of PPSMV in the eriophyid mites. However a minimum of 10 mite extracts was required to get a weak signal by DIBA and a strong positive signal could only be obtained from an extract of 180 mites (Kulkarni et al. 2002). First RT-PCR based diagnostic assay was based on amplification about 250 nt RNA3 sequence of PPSMV (Kumar et al. 2003). Based on the current knowledge the primers developed by Kumar et al. (2003) were specific to PPMSV-1. Availability of full-length sequences of several emaraviruses led to the development of emaravirus-specific degenerate PCR primers for amplification of partial RdRp gene sequence (Elbeaino et al. 2013). Recently a diagnostic multiplex-RT-PCR technique for detection and differentiation PPSMV1 and PPSMV2 has also been developed (Patil et al. 2017). The terminal 13 nt sequences of each emaraviral RNA segment are similar and exhibit nucleotide complementarities and this could help in developing primers for amplification of full length RNA segments of emaraviruses by RT-PCR. Next generation sequencing (NGS) methods have also enhanced the speed at which unknown viruses are identified significantly reducing the costs and advancement in its performance. NGS was employed to get the first genome sequences of PPSMV-1 and PPSMV-2 (Elbeaino et al. 2014, 2015).
6 Concluding Remarks
SMD is known to occur in South East Asia alone, although pigeonpea is cultivated in almost all the continents. The virus vector, A. cajani, has been shown to occur in Southeast Asia, at least as far as Myanmar, but there is no information about its occurrence on pigeonpea in other continents. Based on available evidence that A. cajani fail to colonize virus resistant cultivars, it is plausible to conclude that A. cajani may not occur in continents/countries where SMD is not known to occur. Interestingly there are no reports of presence of any other emaraviruses other than PPSMV-1 and PPSMV-2 from the Indian subcontinent. Current knowledge is insufficient to understand the origin and evolution of PPSMVs. This subject gets more complicated considering the high sequence diversity between PPSMV-1 and PPSMV-2 genomes, and close affiliation of PPSMV-2 to Fig mosaic virus occurring in Europe, than with PPSMV-1 which appears to be distinct compared to other Emaraviruses reported in Europe and North America. Despite high diversity, both PPSMV-1 and PPSMV-2 can infect same host, can cause SMD, are transmitted by the same insect vector, can also co-infect the same plant and recent evidence suggests genomic segment reassortment between the two viruses (Patil et al. 2017) indicating some co-evolution. It is likely that more emaraviruses in Indian-subcontinent are waiting to be discovered and such discovery studies should focus on host plants of eriophyid mites as these arthropods seems to be the main vector of emaraviruses. Further studies on PPSMV sequence diversity from across India and search for alternative hosts using new diagnostics should help in understanding the evolution of PPSMV-1 and PPSMV-2. Although the PPSMV-1 and PPSMV-2 genome sequences have been unraveled, the proteins encoded by them are yet to be characterized and should help to understand the biology of this novel emaravirus. Studies on virus-host and virus-insect interactions are necessary to find out a durable solution to this menace. Significant advancement in reliable, economical and reproducible diagnostics of SMD is still awaited. Field testing diagnostic methods enables the end users to accelerate their decision making by generating results at the point of sampling of the virus infected plant material. Some of the recently developed diagnostic methods, such as the Lateral Flow Devices (LFDs), Loop-mediated isothermal Amplification (LAMP), and the microarrays offer unique advantages and are promising for diagnostics of emaraviruses (Boonham et al. 2014).
Although broad-based resistance to SMD has been reported in the wild species such as Cajanus scarabaeoides, and resistance in some C. scarabaeoides accessions was to vector and some accessions were resistant to PPSMV (Kulkarni et al. 2003a; Kumar et al. 2005; Sharma et al. 2012). Studies on inheritance of SMD resistance in different genetic backgrounds of pigeonpea against different PPSMV isolates has led to contrasting interpretations on genetics and inheritance of SMD resistance (Bhairappanavar et al. 2014; Srinivas et al. 1997; Kumar et al. 2005; Gnanesh et al. 2011; Ganapathy et al. 2009, 2012). Hence the previous reports on genetics and inheritance of SMD resistance are not very conclusive. Thus, more efforts are required to map the disease resistance loci by using molecular tools, both in the cultivated and/or wild-type plants. The pigeonpea genome sequence should help in identification and isolation of R genes (NBS-LRR genes) against PPSMV, using different tools and techniques (Singh et al. 2012; Varshney et al. 2012). Significant progress is required in pigeonpea tissue culture and transformation to realize virus resistant transgenic pigeonpea (Krishna et al. 2010). RNA-interference (RNAi) is one of the most successful technologies widely used to accomplish virus resistant transgenics and it has been used to control orthotospoviruses, which have -ve sense RNA genome (Patil et al. 2011; Peng et al. 2014). RNAi-based transgenic resistance could be a promising for control of PPSMV and hitherto this technology has not been employed for transgenic management of emaraviruses (Patil and Kumar 2015).
The discovery of the causal agent of SMD and unraveling the viral genome sequences are important milestones, however, it is a long way to achieve the thorough understanding of the biological significance of single and mixed infections of PPSMV-1 and PPSMV-2 and their impact on the host resistance, virus-vector-host interactions, and development and delivery of broad-based virus resistant pigeonpea genotypes to the farming community of India.
References
Bhairappanavar SB, Fakrudin B, Sambasiva Rao KRS (2014) Inheritance studies of sterility mosaic disease (SMD) resistance cross Gullyal White×BSMR736 in pigeonpea (Cajanus cajan (L.) Millsp.) Plant Gene Trait 5:27–32
Boonham N, Kreuze J, Winter S, van der Vlugt R, Bergervoet J, Tomlinson J, Mumford R (2014) Methods in virus diagnostics: from ELISA to next generation sequencing. Virus Res. 186:20–31
Bruenn JA (2003) A structural and primary sequence comparison of the viral RNA-dependent RNA polymerases. Nucleic Acids Res. 31:1821–1829
Channabasavanna GP (1981) Contributions to acarology in India. In: Channabasavanna GP, Mallik B, Ghopardé KD (eds) Proceedings of the 1st All India Symposium in Acarology, Bangalore
Dharmaraj PS, Narayana YD, Kumar PL, Waliyar F, Jones AT (2004) Pigeonpea sterility mosaic disease: an emerging problem in northern Karnataka. Int. Chickpea Pigeonpea Newsl. 11:47–49
Di Bello PL, Ho T, Tzanetakis IE (2015) The evolution of emaraviruses is becoming more complex: seven segments identified in the causal agent of Rose rosette disease. Virus Res. 210:241–244
Di Bello PL, Laney AG, Druciarek T, Ho T, Gergerich RC, Keller KE, Martin RR, Tzanetakis IE (2016) A novel emaravirus is associated with redbud yellow ringspot disease. Virus Res. 222:41–47
Divya P, Kumar PL, Rangaswamy KT, Muniyappa V (2005) Detection of pigeonpea sterility mosaic virus in floral parts and seeds. Indian J. Virol. 16:36
Duijsings D, Kormelink R, Goldbach R (2001) In vivo analysis of the TSWV cap-snatching mechanism: Single base complementarity and primer length requirements. EMBO J. 20:2535–2552
Elbeaino T, Whitfield A, Sharma M, Digiaro M (2013) Emaravirus-specific degenerate PCR primers allowed the identification of partial RNA-dependent RNA polymerase sequences of Maize red stripe virus and Pigeonpea sterility mosaic virus. J. Virol. Methods 188:37–40
Elbeaino T, Digiaro M, Uppala M, Sudini H (2014) Deep sequencing of pigeonpea sterility mosaic virus discloses five RNA segments related to emaraviruses. Virus Res. 188:27–31
Elbeaino T, Digiaro M, Uppala M, Sudini H (2015) Deep sequencing of dsRNAs recovered from mosaic-diseased pigeonpea reveals the presence of a novel emaravirus: pigeonpea sterility mosaic virus 2. Arch. Virol. 160:2019–2029
Elliott RM, Blakqori G (2011) Molecular biology of orthobunyaviruses. In: Plyusnin A, Elliott RM (eds) The Bunyaviridae: Molecular and Cellular Biology. Horizon Scientific Press, Norwich
Ganapathy KN, Byregowda M, Venkatesha SC, Rama Chandra R, Gnanesh BN, Girish G (2009) Identification of AFLP markers linked to sterility mosaic disease in pigeonpea Cajanus cajan (L.) Millsp. Int. J. Integr. Biol. 7:145–149
Ganapathy KN, Gowda BM, Ajay BC, Venkatesha SC, Gnanesh BN, Gomashe SS, Prasanth BG, Girish PS, Prasad GN, Veerakumar, Pati JV (2012) Inheritance studies of sterility mosaic disease (SMD) resistance in vegetable type pigeonpea (Cajanus cajan(L.) Millsp.) Aust J Crop Sci. 6:1154–1158
Ghanekar AM, Sheila VK, Beniwal SPS, Reddy MV, Nene YL (1992) Sterility mosaic of pigeonpea. In: Singh US, Mukhopadhyay AN, Kumar J, Chaube HS (eds) Plant Diseases of International Importance, Diseases of Cereals and Pulses, vol 1. Prentice Hall, New Jersey, pp 415–428
Gnanesh BN, Abhishek B, Sarma M, Wesley V, Byre Gowda M, Pande S, Saxena R, Saxena KB, Varshney RK (2011) Genetic mapping and quantitative trait locus analysis of resistance to sterility mosaic disease in pigeonpea [Cajanus cajan (L) Millsp.] Field Crop Res. 123:56–61
Hassan M, Di Bello PL, Keller KE, Martin RR, Sabanadzovic S, Tzanetakis IE (2017) A new, widespread emaravirus discovered in blackberry. Virus Res 235:1–5. doi:10.1016/j.virusres.2017.04.006
Ishikawa K, Miura C, Maejima K, Komatsu K, Hashimoto M, Tomomitsu T, Fukuoka M, Yusa A, Yamaji Y, Namba S (2015) Nucleocapsid protein from Fig mosaic virus forms cytoplasmic agglomerates that are hauled by endoplasmic reticulum streaming. J. Virol. 89(1):480–491
Jones AT, Kumar PL, Saxena KB, Kulkarni NK, Muniyappa V, Waliyar F (2004) Sterility mosaic disease-the “green plague” of pigeonpea: advances in understanding the etiology, transmission and control of a major virus disease. Plant Dis. 88:436–445
Kannaiyan J, Nene YL, Reddy MV, Ryan JG, Raju TN (1984) Prevalence of pigeonpea diseases and associated crop losses in Asia, Africa and the Americas. Trop Pest Manag 30:62–71
Kaushik D, Srivastava S, Nath BC, Chauhan VB, Singh R (2013) Correlation between mite population (Aceria cajani) and environmental factors causing sterility mosaic disease of Pigeonpea. Int. J. Life Sci. 1:228–232
Kikkert M, van Lent J, Storms M, Bodegom P, Kormelink R, Goldbach R (1999) Tomato spotted wilt virus particle morphogenesis in plant cells. J. Virol. 73:2288–2297
Kormelink R, Garcia ML, Goodin M, Sasaya T, Haenni AL (2011) Negative-strand RNA viruses: the plant-infecting counterparts. Virus Res. 162:184–202
Krishna G, Reddy PS, Ramteke PW, Bhattacharya PS (2010) Progress of tissue culture and genetic transformation research in pigeonpea [Cajanus cajan (L.) Millsp.] Plant Cell Rep. 29:1079–1095
Kulkarni NK, Kumar PL, Muniyappa V, Jones AT, Reddy DVR (2002) Transmission of Pigeonpea sterility mosaic virus by the eriophyid mite, Aceria cajani (Acari: Arthropoda). Plant Dis. 86:1297–1302
Kulkarni NK, Kumar PL, Muniyappa V, Jones AT, Reddy DVR (2003a) Broad-based resistance to pigeonpea sterility mosaic disease in the accessions of Cajanus scarabaeoides. Ind. J. Plant Protect. 31:6–11
Kulkarni NK, Kumar PL, Muniyappa V, Jones AT, Reddy DVR (2003b) Studies on host range of Pigeonpea sterility mosaic virus. J. Mycol. Plant Pathol. 33:141–145
Kumar PL, Jones AT, Sreenivasulu P, Reddy DVR (2000) Breakthrough in the identification of the causal agent of pigeonpea sterility mosaic disease. J Mycol Plant Pathol. 30:249
Kumar PL, Fenton B, Duncan G, Jones AT, Sreenivasulu P, Reddy DVR (2001) Assessment of variation in Aceria cajani (Acari: Eriophyidae) using analysis of nuclear rDNA ITS regions and scanning electron microscopy: Implications for the variability observed in host plant resistance to pigeonpea sterility mosaic disease. Ann. Appl. Biol. 139:61–73
Kumar PL, Jones AT, Reddy DVR (2002a) Mechanical transmission of Pigeonpea sterility mosaic virus. J. Mycol. Plant Pathol. 32:88–89
Kumar PL, Duncan GH, Roberts IM, Jones AT, Reddy DVR (2002b) Cytopathology of Pigeonpea sterility mosaic virus in pigeonpea and Nicotiana benthamiana: similarities with those of eriophyid mite-borne agents of undefined aetiology. Ann. Appl. Biol. 140:87–96
Kumar PL, Jones AT, Reddy DVR (2003) A novel mite-transmitted virus with a divided RNA genome closely associated with pigeonpea sterility mosaic disease. Phytopathology 93:71–81
Kumar PL, Latha TKS, Kulkarni NK, Raghavendra N, Saxena KB, Waliyar F, Rangaswamy KT, Muniyappa V, Doriswamy S, Jones AT (2005) Broad based resistance to pigeonpea sterility mosaic disease in wild relatives of pigeonpea (Cajanus: Phaseoleae). Ann. Appl. Biol. 146:371–379
Kumar PL, Jones AT, Waliyar F, Sreenivasulu P, Muniyappa V, Latha TKS, Saxena KB (2007) Pigeonpea sterility mosaic disease. In: Kumar PL, Waliyar F (eds) Diagnosis and detection of viruses infecting ICRISAT mandate crops. ICRISAT’s methods manual
Kumar PL, Jones AT, Waliyar F (2008) Virus diseases of pigeonpea. In: Rao GP, Kumar PL, Holguin-Pena RJ (eds) Characterization, Diagnosis and Management of Plant Viruses, Vegetable and Pulse Crops, vol 3. Studium Press, Texas
Laney AG, Keller KE, Martin RR, Tzanetakis IE (2011) A discovery 70 years in the making: characterization of the Rose rosette virus. J. Gen. Virol. 92:1727–1732
Latha TKS, Doraiswamy S (2008) Detection of pigeonpea sterility mosaic virus, the causal agent of sterility mosaic disease of pigeonpea in viruliferous mite vector by DAS-ELISA and DIBA. Arch. Phytopathol. Plant Protect. 41:537–541
McGavin WJ, Mitchell C, Cock PJ, Wright KM, MacFarlane SA (2012) Raspberry leaf blotch virus, a putative new member of the genus Emaravirus, encodes a novel genomic RNA. J. Gen. Virol. 93:430–437
Mielke N, Muehlbach HP (2007) A novel, multipartite, negative-strand RNA virus is associated with the ringspot disease of European mountain ash (Sorbus aucuparia L.) J. Gen. Virol. 88:1337–1346
Mielke-Ehret N, Muhlbach HP (2012) Emaravirus: a novel genus of multipartite, negative strand RNA plant viruses. Virus 4:1515–1536
Mitra M (1931) Report of the imperial mycologist. Sci. Rep. Agric. Res. Inst. Pusa 19:58–71
Mühlbach HP, Mielke-Ehret N (2011) Emaravirus. In: AMQ K, Adams MJ, Carstens EB, Lefkovitz EJ (eds) Virus Taxonomy. Classification and Nomenclature of Viruses, Ninth Report of the International Committee on Taxonomy of Viruses. Elsevier, Oxford
Narayana YD, Mahalinga DM, Jayalakshmi SK, Benagi VI (2000) Prevalence of SMD of pigeonpea in Northern Karnataka. Karnataka J. Agric. Sci. 13:470–472
Nene YL (1995) Sterility mosaic of pigeonpea: the challenge continues. Indian J. Mycol. Plant Pathol. 25:1–11
Nene YL, Reddy MV (1976a) Screening for resistance to sterility mosaic of pigeonpea. Plant Dis. Rep. 60:1034–1036
Nene YL, Reddy MV (1976b) A new technique to screen pigeonpea for resistance to sterility mosaic for pigeonpea. Trop. Grain Legume Bull. 5:23–24
Oldfield G, Proeseler G (1996) Eriophiod mites as vectors of plant pathogens. In: Lindquist EE, Sabelis MW, Bruin J (eds) Eriophyoid Mites-Their Biology, Natural Enemies and Control. Elsevier, Amsterdam
Patil BL, Lava Kumar P (2015) Pigeonpea sterility mosaic virus: a legume-infecting Emaravirus from South Asia. Mol. Plant Pathol 16:775–786
Patil BL, Dangwal M, Mishra R (2017) Variability of Emaravirus species associated with sterility mosaic disease of pigeonpea in India provides evidence of segment reassortment. Viruses 9(7):183
Patil BL, Ogwok E, Wagaba H, Mohammed IU, Yadav JS, Bagewadi B, Taylor NJ, Kreuze JF, Maruthi MN, Alicai T, Fauquet CM (2011) RNAi-mediated resistance to diverse isolates belonging to two virus species involved in Cassava brown streak disease. Mol. Plant Pathol. 12:31–41
Peng JC, Chen TC, Raja JA, Yang CF, Chien WC, Lin CH, Liu FL, Wu HW, Yeh SD (2014) Broad-spectrum transgenic resistance against distinct tospovirus species at the genus level. PLoS One 9:e96073
Reddy MV, Nene YL (1980) Influence of sterility mosaic resistant pigeonpeas on multiplication of the mite vector. Ind Phytopathol 33:61–63
Reddy MV, Raju TN, Nene YL, Ghanekar AM, Amin KS, Arjunan G, Astaputre JV, Sinha BK, Reddy SV, Gupta RP, Gangadharan K (1993) Variability in sterility mosaic pathogen in pigeonpea in India. Indian Phytopathol. 46:206–212
Reddy MV, Raju TN, Lenne JM (1998) Diseases of pigeonpea. In: Allen DJ, Lenne JM (eds) The Pathology of Food and Pasture legumes. CAB International, Wallingford, pp 517–558
Sharma M, Rathore A, Mangala UN, Ghosh R, Sharma S, Upadhyay H, Pande S (2012) New sources of resistance to Fusarium wilt and sterility mosaic disease in a mini-core collection of pigeonpea germplasm. Eur. J. Plant Pathol. 133:707–714
Singh J, Raghuraman M (2011) Emerging scenario of important mite pests in north India. Zoosymposia 6:170–179
Singh AK, Rathi YPS (1997) Epidemiology of vector of pigeonpea sterility mosaic virus. Ind. J. Virol. 13:143–145
Singh NK, Gupta DK, Jayaswal PK, Mahato AK, Dutta S, Singh S, Bhutani S, Dogra V, Sing BP, Kumawat G, Pal JK, Pandit A, Singh A, Rawal H, Kumar A, Rama Prashat G, Khare A, Yadav R, Raje RS, Singh MN, Datta S, Fakrudin B, Wanjari KB, Kansal R, Dash PK, Jain PK, Bhattacharya R, Gaikwad K, Mohapatra T, Srinivasan R, Sharma TR (2012) The first draft of the pigeonpea genome sequence. J. Plant Biochem. Biotechnol. 21:98–112
Srinivas T, Reddy MV, Jain KC, Reddy MSS (1997) Studies on inheritance of resistance and allelic relationships for strain 2 of Pigeonpea sterility mosaic pathogen. Ann. Appl. Biol. 130:105–110
Tatineni S, McMechan AJ, Wosula EN, Wegulo SN, Graybosch RA, French R, Hein GL (2014) An eriophyid mite-transmitted plant virus contains eight genomic RNA segments with unusual heterogeneity in the nucleocapsid protein. J. Virol. 88:11834–11845
Varshney RK, Chen W, Li Y, Bharti AK, Saxena RK, Schlueter JA, Donoghue MT, Azam S, Fan G, Whaley AM, Farmer AD, Sheridan J, Iwata A, Tuteja R, Penmetsa RV, Wu W, Upadhyaya HD, Yang SP, Shah T, Saxena KB, Michael T, McCombie WR, Yang B, Zhang G, Yang H, Wang J, Spillane C, Cook DR, May GD, Xu X, Jackson SA (2012) Draft genome sequence of pigeonpea (Cajanus cajan), an orphan legume crop of resource-poor farmers. Nat. Biotechnol. 30:83–89
Yu C, Karlin DG, Lu Y, Wright K, Chen J, MacFarlane S (2013) Experimental and bioinformatic evidence that Raspberry leaf blotch emaravirus p4 is a movement protein of the 30K superfamily. J. Gen. Virol. 94:2117–2128
Zheng Y, Navarro B, Wang G, Wang Y, Yang Z, Xu W, Zhu C, Wang L, Serio FD, Hong N (2017) Actinidia chlorotic ringspot-associated virus: a novel emaravirus infecting kiwifruit plants. Mol. Plant Pathol. 18(4):569–581
Zote KK, Mali VR, Mayee CD, Kulkarni SV, Mote TS (1991) Outbreak of sterility mosaic of pigeonpea in Marathwada region Maharashtra. India. Int. Pigeonpea Newsl. 14:19–21
Acknowledgement
BLP acknowledges the financial support from ICAR-NRCPB to work on PPSMVs. Authors gratefully acknowledge research contributions of all the students, research scholars and scientists and a number of philanthropic donors for financial assistance to research work on SMD spanning over 85 years in India.
Author information
Authors and Affiliations
Corresponding author
Editor information
Editors and Affiliations
Rights and permissions
Copyright information
© 2017 Springer Nature Singapore Pte Ltd.
About this chapter
Cite this chapter
Patil, B.L., Lava Kumar, P. (2017). Pigeonpea Sterility Mosaic Emaraviruses: A Journey from a Mysterious Virus to Classic Emaraviruses. In: Mandal, B., Rao, G., Baranwal, V., Jain, R. (eds) A Century of Plant Virology in India. Springer, Singapore. https://doi.org/10.1007/978-981-10-5672-7_10
Download citation
DOI: https://doi.org/10.1007/978-981-10-5672-7_10
Published:
Publisher Name: Springer, Singapore
Print ISBN: 978-981-10-5671-0
Online ISBN: 978-981-10-5672-7
eBook Packages: Biomedical and Life SciencesBiomedical and Life Sciences (R0)