Abstract
Silver nanoparticles have unique assets which lead in molecular diagnostics, therapeutics, and devices that are used in several medical procedures. The major procedures used for silver nanoparticle synthesis are the physical and chemical methods. The problems with the chemical and physical methods, the synthesis is expensive and can also have toxic materials absorbed onto them. To overwhelm this, the biological procedures provide a reasonable alternative. In the biological systems involved in the bacteria, actinomycetes, fungi, algae, virus, and plant extracts. Most applications of silver nanoparticles are in the therapeutics, like antimicrobial and anti-inflammatory properties. This chapter provides a wide-ranging understanding on the mechanism of action, production, and application in plant protection.
Access provided by CONRICYT-eBooks. Download chapter PDF
Similar content being viewed by others
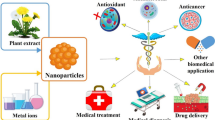
Keywords
- Silver nanoparticles
- Antimicrobial
- Phytopathogens
- Antibacterial mechanism
- Nano-antifungal
- Nano-antiviral
- Nanopesticides
- Nanofertilizers
9.1 Introduction
The word “nano” is the one billionth of a meter or 10−9. The term nanotechnology was coined by Professor Norio Taniguchi of Tokyo University of Science in 1974 who illustrated the precise manufacturing of materials at the nanoscale level (Taniguchi 1974). In green nanotechnology, microorganisms and plants are used for the synthesis of nanoparticles (NPs). It is well known that many microorganisms are capable of aggregating inorganic material within or outside the cell to form NPs. However, an enormous number of microbial species are capable of producing metal NPs, and the mechanism of NPs biosynthesis is very important. Microbial synthesis of NPs is an approach that interconnects nanotechnology and microbial biotechnology . Biosynthesis of many metals nanoparticles like gold, silver, gold-silver alloy, selenium, tellurium, platinum, palladium, silica, titania, zirconia, quantum dots, magnetite, and uraninite by bacteria, actinomycetes, fungi, yeasts, algae, and viruses has been reported (Narayanan and Sakthivel 2010; Prasad et al. 2016). Silver nanoparticles (AgNPs) have become one of the most commonly used nanomaterials in consumer products, and for several decades, silver (Ag+) has been studied as an antimicrobial agent against various harmful microorganisms (Prasad 2014).
Due to plant disease agricultural production is reduced worldwide every year; therefore, millions of moneys have been invested in efforts to control the plant diseases. Various natural and artificial methods of control for protection of plants from these diseases have been applied. Among methods for disease control, use of pesticides is the most prevalent. In recent years, environmental hazards caused by excessive use of pesticides have been widely discussed; therefore, researchers in the agricultural field are searching for alternative measures against pesticides. Nanotechnological applicability in crop disease protection offers a great promise in the management of insects and pathogens. AgNPs are very effective against phytopathogens with low toxicity and lead to broad range of applicability in pesticidal activity. It is efficiently used for site-targeted delivery of important agrochemical products and for diagnosis purpose tools in case of prior detection of plant diseases (Chowdappa and Shivakumar 2013). AgNPs are the most studied and utilized NPs in the field of agricultural research to improve the efficiency, yield, and sustainability of agricultural crops. It has long been known to have strong pesticidal, antifungal, antiviral, and bactericidal effects (Chen and Schluesener 2008). Due to its broad spectrum of antimicrobial activities, AgNPs have the prospect to increase food quality, global food production, plant protection, detection and regulation of plant diseases, monitoring of plant growth, and pest control for “sustainable agricultural development ” (Kim et al. 2012; Khan and Rizvi 2014; Prasad et al. 2017a, 2017b). AgNPs are highly stable and very well dispersive in aqueous solution. It is being used as foliar spray to inhibit the growth of fungi, molds, rot, and several other plant diseases (Singh et al. 2015). Moreover, AgNPs are also used as an excellent plant-growth stimulator . It provides novel tool for the management of diseases, rapid disease detection, and minimizing nutrient losses in fertilization through an optimized nutrient management (Pérez-de-Luque and Rubiales 2009). As an alternative to chemically manufactured pesticides, use of AgNPs as antimicrobial agents has become more common as technological advances make their production more economical. One of the important appliances of AgNPs is in the management of plant diseases. Silver displays multiple modes of inhibitory action against microorganisms; therefore, it may be used with relative safety for control of various plant pathogens, compared to synthetic fungicides (Aziz et al. 2016; Prasad et al. 2014, 2017a, 2017b).
Artificial chemical antimicrobials are widely used in modern agriculture to control plant diseases. Environmental hazards caused by excessive use of pesticides pose health problems as modern society is becoming more health-conscious. Therefore, agricultural scientists are searching for alternative eco-friendly and less capital-intensive approaches to control plant diseases. As an alternative to chemically manufactured pesticides, use of AgNPs as antimicrobial agents has become more common as technological advances make their production more economical. So, the focus of this chapter is to study the possibilities of using the synthesized AgNPs in plant protection (Fig. 9.1).
9.2 Chemical and Biological Silver Nanoparticles (AgNPs)
The physical and chemical methods are numerous in number for the synthesis of AgNPs, and many of these methods are expensive or use toxic substances which are major factors that make them “not so favored” methods of synthesis. Various types of physical and chemical methods that are employed in the production of nanoparticles are top-down method, bottom-up method, reduction of silver metal salt, electroreduction of AgNO3 in aqueous solution in the presence of polyethylene glycol, son decomposition, photoreduction in reverse micelle, and many more. The quest for such a method has led to the need for biomimetic production of silver nanoparticles whereby biological methods (using plants and microbes) are used to synthesize AgNPs. Biologically, various types of microbes and plants species are used for the biosynthesis of nanoparticles. In utmost cases, the chemical synthesis methods lead to some chemically toxic materials being absorbed on the surface and can hinder their practice in medical applications (Parashar et al. 2009; Swamy and Prasad 2012; Prasad and Swamy 2013; Prasad et al. 2016). Biological synthesis of AgNPs is a bottom-up method that typically involves reduction/oxidation reactions. The microbial enzymes or the plant phytochemicals with antioxidant or reducing properties act on the respective compounds and give the desired nanoparticles. The three major components involved in the biological synthesis of nanoparticles: solvent medium, the eco-friendly reducing agent, and a nontoxic stabilizing agent. The nanoparticles so produced have protein cap over it imparted by biological host. This capping helps in easy entry in pathogenic fungi. Presence of peak at 1654 in FTIR spectrum indicates the presence of amide bond in the sample indicating the presence of protein capping which is responsible for stabilizing the synthesized nanoparticles (Aziz et al. 2015).
9.3 Mechanism by Which Pathogens Cause Diseases
Pathogens cause infection via various mechanisms. Some common known mechanisms are:
-
1.
Cell wall degradation : some pathogens have enzymes which can degrade the cell walls of plant leading to the easy access to the host plant.
-
2.
Toxins : these chemicals are further categorized into host specific, which are specific for few plants, and non-host specific, which are active against all plants.
-
3.
Effector proteins : these proteins interfere with the chemical signaling pathways of the host plant which results in reduction of phytochemical production (Winbo 2011).
9.4 Mechanistic Approach of antimicrobial activity of Silver Nanoparticles and Controls of the Growth of Pathogens
There are numerous mechanisms by which AgNPs control the growth of pathogens . The exact mechanism of AgNPs by which it causes antimicrobial effect is not evidently known and is one of the debated topics. Numerous theories on which action of AgNPs based by which it cause antimicrobial effects. Silver nanoparticles possess the ability to anchor the bacterial cell wall and penetrate it, causing structural changes in the cell membrane like cell membrane permeability and cell death. There are formation of “pits” and accumulation of the nanoparticles on the cell surface. The formation of free radicals by the AgNPs may be considered as another mechanism by which the cells die. The electron spin resonance spectroscopy studies suggest that there is a formation of free radicals when AgNPs contact the bacteria and these free radicals make membrane porous which ultimately leads to cell death (Danilcauk et al. 2006; Kim et al. 2007). It has also been proposed that there may be a release of silver ions by the nanoparticles (Feng et al. 2008) and these ions have inbuilt property to interact with the thiol (-SH) groups of many crucial enzymes and inactivate them (Matsumura et al. 2003). The bacterial cells come in contact with silver ions, which inhibit many functions and damage the cells which result in the generation of reactive oxygen species (ROS) that may also be produced possibly through the inhibition of a respiratory enzyme by silver ions and attack the cell itself. The action of these nanoparticles on the cell can cause the reaction to take place and subsequently lead to cell death. Another important fact is that the DNA contains sulfur and phosphorus as their major components; the nanoparticles can act on these components and destroy the DNA which ultimately lead to cell death (Hatchett and Henry 1996). The interaction of the silver nanoparticles with the sulfur and phosphorus of the DNA can lead to problems in the DNA replication of the bacteria and thus terminates their growth. It has also been found that the AgNPs can modulate signal transduction in bacteria. It is a well-known fact that phosphorylation of protein substrates in bacteria persuade bacterial signal transduction. Dephosphorylation is only reported in the tyrosine residues of gram-negative bacteria. The phosphor tyrosine profile of bacterial peptides is altered by the nanoparticles. It was found that the nanoparticles dephosphorylate the peptide substrates on tyrosine residues , which leads to signal transduction inhibition and thus the stoppage of growth. It is however necessary to understand that further research is required on the topic to thoroughly establish the claims (Shrivastava et al. 2007) (Fig. 9.2).
Mechanistic approach of the antibacterial action indicating ROS generation induced by AgNPs. (Reprinted from Aziz et al. 2015)
9.5 Effect of Silver Nanoparticles on Phytopathogens
Phytopathogens , viz., bacteria, fungi, viruses and nematodes, are key limiting factors in the production of food material. Several methods are used to control pathogens but not a perfect method to control of the disease. Hence, a great prospect exists for the manipulation of nanotechnology for the management of plant pathogens. Silver is considered the most capable nanomaterials with fungicidal, bactericidal, and viricidal properties owing to its wide-ranging effectiveness, low toxicity, ease of use, charge capacity, high surface-to-volume ratios, crystallographic structure, and adaptability to several substrates (Nangmenyi and Economy 2009). AgNPs act as robust antimicrobial agent due to strong inhibitory effects against various microorganisms (Clement and Jarrett 1994). Nanosilver exhibits high level of toxicity to the microorganisms and lower toxicity to the mammalian cells. It was observed that the microbe-killing effects of AgNPs were size dependent (Raza et al. 2017). The AgNPs/PVP were tested for fungicidal activity against different yeasts and molds such as Candida albicans, C. krusei, C. tropicalis, C. glabrata, and Aspergillus brasiliensis. The hybrid materials showed strong antifungal effects against the tested microbes (Bryaskova et al. 2011). Traditional microbiological plating , scanning electron microscopy , and Raman spectroscopy were used to study antifungal activities of AgNPs and to characterize the changes in morphology and cellular compositions of fungal hyphae. Aziz et al. (2016) observed the effect of biogenic nanoparticles from AgNPs on pathogenic fungi, Candida albicans, Fusarium oxysporum, and Aspergillus flavus, and these antimicrobial attributes were comparable to those of established fungicides (amphotericin B, fluconazole, and ketoconazole). Importantly, these nanoparticles show significant synergistic characteristics when combined with the antibiotics and fungicides to offer substantially greater resistance to microbial growth. Ocsoy et al. (2013) developed nanocomposite DNA-directed AgNPs grown on graphene oxide (Ag@dsDNA@GO). These composites effectively decrease Xanthomonas perforans cell viability in culture and on plants. At the very low concentration (16 ppm), composites show excellent antibacterial ability with significant benefits in improved stability and higher antibacterial activity. Also, in most cases, inhibition increased as the concentration of AgNPs increased. This could be due to the high density at which the solution was able to saturate and cohere to fungal hyphae and to deactivate plant pathogenic fungi (Kim et al. 2012). Synthesis of nanoparticles chemically requires chemical substances that are toxic in nature. Even after purification, there is a chance of chemical contamination which leads to unsafe use of nanoparticles. Chemical and physical methods need expensive chemicals and instruments, which leads to hike in production cost. However, biologically synthesized nanoparticles don’t undergo any toxic and expensive procedure. Laboratory test reveals that biologically synthesized nanoparticles are safest to use and these nanoparticles possess protein caps which allow easy excess to the pathogen cell membrane.
Nano Silver is one of the known strong bacteriostats and possesses broad-spectrum antimicrobial activity. It has been reported that well-dispersed nanosilver colloid is more adhesive to bacteria and fungi leading to enhanced antimicrobial activity (Kim et al. 2008). In nature many agricultural crops and forestry are attacked by many microorganisms resulting in loss of agricultural product and death of tree species. AgNPs came up as a new hope and control disease mechanism by damaging fungal hyphae, interference with nutrient absorption, and enhanced inhibition of fungal growth and germination. The mechanism involved may be the influence of silver ions and nanoparticles on spore formation and disease progression in plant pathogenic fungi. Hence, AgNPs prove to have high potential to be used as nanopesticides for controlling phytopathogens (Alghuthaymi et al. 2015).
9.5.1 Nano-antibacterial
Silver nanoparticles in agricultural soil affect several bacterial communities which are beneficial/harmful for plant and environment (Panyala et al. 2008). AgNPs act as strong antimicrobial agent due to strong inhibitory effects against various bacterial species (Clement and Jarrett 1994; Joshi et al. 2018). Kamran et al. (2011) reported that the nanosilver and nano-TiO2 with a good potential may be used for removing the bacterial contaminants in the tobacco plant. AgNP exposure causes toxicity to bacteria, and treatment can prevent replication and protein synthesis (Chaloupka et al. 2010). Notably, the most common application problem involves the agglomeration and diffusion of these nanoparticles, which reduce antibacterial activity. These studies revealed that used various organic (Jo et al. 2009) and inorganic substances (Lamsal et al. 2011) as well as powerful carriers (Ouda 2014) to stabilize AgNPs . These substances can strongly influence the antibacterial activity and reduce the biological toxicity of nanoparticles. Also a synergistic antimicrobial effect is achieved when AgNPs are hybrid with other metal nanoparticles or oxides acting as a shell or a core to form bimetallic nanoparticles (Chou and Chen 2007). Chen et al. (2016) study revealed that bacteriostatic and bactericidal activity of the pure and surfactant-stabilized AgNPs (SDS-Tween 80- CTAB-PVP) capped silver nanoparticles. Ralstonia solanacearum (phytopathogenic fungi), which causes severe bacterial wilt in tobacco, is used to investigate the bacteriostatic and bactericidal activity of pure and surfactant-stabilized AgNPs . The surfactants affected the antibacterial activity of AgNPs toward R. solanacearum to different extents.
9.5.1.1 AgNP Antibacterial Mechanism
The accumulation of AgNPs in the cellular membrane led to an increase of its permeability and eventually to the death of bacterial cells. Also, they attempted to understand their mechanism of action. Presently, there are five main explanations that have been proposed to describe the antibacterial activity (Lemire et al. 2013):
-
1.
Release of toxic ions that bind to sulfur-containing proteins – this accumulation avoids the proper functioning of proteins in the membrane and interfere in cell permeability (Sondi and Salopek-Sondi 2004).
-
2.
They may be genotoxic – toxic ions can DNA destruction which leads to death of cell.
-
3.
Interruption of electron transport chain, protein oxidation system, and collapse of membrane potential.
-
4.
Generation of reactive oxygen species (ROS)-mediated cellular damage and different metal-catalyzed oxidation reactions might underlie specific types of DNA, protein, and membrane damage (Banerjee et al. 2010; Zeng et al. 2007; Aziz et al. 2015).
-
5.
Interruption with uptake of nutrients (Pal et al. 2007).
These mechanisms might not operate separately which suggests that more than one mechanism occur simultaneously. These multiple targets of action might access NPs to fight effectively against different plant pathogens.
9.5.2 Nano-antiviral
For AgNP role against plant viruses, there are few reports documented. For instance, the effective control of bean yellow mosaic virus (BYMV) , genus Potyvirus, family Potyviridae, would be of high interest for many African countries, which can suffer significant yield reductions in fava bean crops upon viral infection leading to considerable economic losses (Radwan et al. 2008). It has been suggested that AgNPs inhibit viral nucleic acid replication, while their antiviral activity depends on the particle size, as well as on the distribution of interacting ligand/receptor molecules (Lü et al. 2009; Papp et al. 2010). Elbeshehy et al. (2015) studied the effect of biosynthesized AgNPs on leaves of fava bean infected with BYMV which showed severe symptoms, including yellow mosaic, mottling, crinkling, size reduction, and deformation, symptoms that were absent from the non-infected leaves.
9.5.3 Nano-antifungal
The antifungal effect of AgNPs has established only insignificant attention and with very few publications (Roe et al. 2008; Kim et al. 2008). There are few studies available dealing precisely with their mechanism of action against clinical isolates and American type culture collection strains of Candida spp. and Trichophyton mentagrophytes (Li et al. 2012; Panáček et al. 2006; Min et al. 2009). The use of AgNPs as antimicrobial agents becomes more widespread as technological advances make their production more economical. Control of phytopathogens is one of the probable applications in which silver can be utilized in the management of plant diseases. Since silver displays a collection of modes of inhibitory action to plant pathogens (Park et al. 2006), it might be used as controlling agent for various plant pathogens in a moderately safer way as compared to synthetic fungicides. Ag-SiO NPs have a strong antifungal effect against Botrytis cinerea (Oh et al. 2006). The combined effect of fluconazole and AgNPs for their antifungal activity was evaluated by Gajbhiye et al. (2009) against Phoma glomerata, Phoma herbarum, F. semitectum, Trichoderma sp., and Candida albicans by disc diffusion technique. Ag2S nanocrystals on amorphous silica particles show antifungal activity against Aspergillus niger (Fateixa et al. 2009). The phytogenic AgNPs were tested against three different plant pathogenic fungi such as Rhizoctonia solani, Fusarium oxysporum, and Curvularia sp. and showed antifungal activity against R. solani followed by F. oxysporum and Curvularia sp. (Balashanmugam et al. 2016). Amphotericin B showed moderate antifungal activity against the three plants pathogenic fungi (Fig. 9.3).
Antifungal activity of phytosynthesized AgNPs against different plant pathogens on the third day (I) Rhizoctonia solani, (II) Fusarium oxysporum, (III) Curvularia sp. D-silver nitrate, S-phytosynthesized AgNPs, A-amphotericin-B, P-C. roxburghii aqueous leaf extract. (Reprinted from Balashanmugam et al. 2016)
9.6 Nano-insecticides
Advance investigation highlighted the extensive application of AgNPs for insecticidal application to kill the mosquitoes and fleas. AgNPs possessed excellent antilice and mosquito larvicidal activity, having dynamic application in community health improvement. There are tremendous researches investigating the efficacies of biogenic synthesized AgNPs as mosquito larvicidal agent against different species of mosquitoes, i.e., Culex quinquefasciatus, Heteroscodra maculata, Rhipicephalus microplus, and Anopheles subpictus, and suggesting that it can be used as an ideal eco-friendly approach for their control (Marimuthu et al. 2011; Suman et al. 2013; Mondal et al. 2014). Jayaseelan et al. (2011) documented on the pediculicidal and larvicidal activity of synthesized AgNPs (from leaf extract of Tinospora cordifolia) against the head louse Pediculus humanus and larvae of Anopheles subpictus and Culex quinquefasciatus and showed maximum mortality. Rouhani et al. (2012) evaluated the insecticidal activity of AgNPs against the Aphis nerii. Soni and Prakash (2015) have described the larvicidal and pupicidal properties of biologically produced AgNPs (from fungal strain of Aspergillus niger) against the mosquito larvae of Aedes aegypti, Culex quinquefasciatus, and Anopheles stephensi.
9.7 Controlled Released Nanofertilizers
Silver nanoparticles are very stable and biodegradable and it also displays slow release of agrochemicals. So, it can be used for formation of nanocapsules for slow and optimized delivery of agrochemicals, pesticides, and fertilizers in agricultural practices (Chowdappa and Shivakumar 2013). Nanoencapsulated agrochemicals are designed to possess the desired properties including effective optimum concentration, time-controlled release, enhanced activity on target site, and least toxic effects (Tsuji 2001). It helps in slow release of agrochemical in controlled way to the particular host through dissolution, biodegradation, diffusion, and osmotic pressure with specific pH. Nanotagged agrochemicals reduce the damage to nontarget plant tissues and reduce risk of nonspecific chemical contamination in the surrounding environment (González-Melendi et al. 2008; Rai and Ingle 2012). Combinations of inorganic fertilizer mainly supply three nutrients, nitrogen (N), potassium (K), and phosphorus (P), to various crops at different growing conditions. This brings out the idea of developing encapsulated fertilizers, in which NPK fertilizers are entrapped within nanosilver to boost nutrient management.
9.8 Positive Effect on Plant Growth
Some nanoparticles have a beneficial effect on some plant species manifested by enhancing seed germination, enhancing crop yield, or suppressing plant disease (Servin et al. 2015; Arruda et al. 2015). As we know that NPs have both positive and negative effects on plant growth and development. Recently, Krishnaraj et al. (2012) studied the effect of biogenic AgNPs on hydroponically grown Bacopa monnieri growth metabolism, showed a significant effect on seed germination, and induced the synthesis of protein and carbohydrate and decreased the total phenol contents and catalase and peroxidase activities. Also, Savithramma et al. (2012) revealed that biologically synthesized AgNPs enhanced seed germination and seedling growth of trees Boswellia ovalifoliolata. AgNPs increased plants morphological (shoot and root length, leaf area) and biochemical attributes (chlorophyll, carbohydrate and protein contents, antioxidant enzymes ) of Brassica juncea, common bean, and corn (Salama 2012; Sharma et al. 2012). However, Gruyer et al. (2013) reported AgNPs have both positive and negative effect on root elongation depending on the plant species. They reported that root length was increased in barley but was inhibited in lettuce. Also, Yin et al. (2012) studied the effects of AgNPs on germination of 11 wetland plants species (Lolium multiflorum, Panicum virgatum, Carex lurida, C. scoparia, C. vulpinoidea, C. crinita, Eupatorium fistulosum, Phytolacca americana, Scirpus cyperinus, Lobelia cardinalis, Juncus effusus) and found AgNPs enhanced the germination rate of 1 species (E. fistulosum). AgNPs induce root growth by blocking ethylene signaling in Crocus sativus (Rezvani et al. 2012).
Silver nanoparticles may have both a positive and a negative impact on plants, depending on size, concentration, chemical composition, zeta potential, stability, and the shape of nanoparticles (Mirzajani et al. 2013; Tripathi et al. 2015, 2017; Costa and Sharma 2016). Several studies have depicted a negative impact of nanoparticles on plants in the form of decrease in plant growth, productivity, and pigments (Tripathi et al. 2017). On the other hand, robust and smart engineered nanoparticles are also explored for the betterment of agricultural crop production, as growth stimulators, nanopesticides, nanofertilizers , soil-improving agents, or sensors for monitoring different agricultural parameters in the field (Fraceto et al. 2016; Prasad et al. 2016, 2017a). Due to the increased interest in the area, most of the research depicting the influence of industrial nanoparticles on plants has been performed in recent years. Recent studies have exposed that when AgNP was combined with different treatment/compounds, it may have a different impact on plants (Berahmand et al. 2012; Belava et al. 2017), due to the influence of other phenomena/compound on AgNPs. AgNP treatment in combination with magnetic field was observed to improve quantitative yields in Zea mays (Berahmand et al. 2012). In the wheat-pathogen phytosystem, an increase of lipid peroxidation was observed, when compared with NP or pathogen alone (Belava et al. 2017). The biosynthesized nanoparticles induced the protein and carbohydrate synthesis and decreased the total phenol contents, which can be considered as a positive effect; it may be due to the presence of altered size (2–50 nm) of nanoparticle or the different chemical property of biogenic NPs. The size of AgNPs of 200–800 nm was observed to enhance the plant growth (Jasim et al. 2016) whereas 35–40 nm observed to positively influence the root and shoot growth of different plant (Pallavi et al. 2016), which may be due to the inability of the penetration of large nanoparticles (Mirzajani et al. 2013). AgNPs (of comparatively small size, i.e., <30 nm) when applied in high concentration were observed to inhibit the root and shoot growth in different plants studied (Dimkpa et al. 2013; Vinković et al. 2017). Treatment of Lupinus termis seedlings with 100 ppm bio-AgNPs might improve the growth profile, while exposure of seedlings to high concentrations (300 and 500 ppm) resulted in a highly significant reduction in all growth parameters and growth indices (Al-Huqail et al. 2018) (Fig. 9.4 and Table 9.1).
(a) Effect of different concentrations of CSL-AgNPs (0–900 ppm) on Lupinus termis L. seed germination. (b) Effect of different concentrations of CSL-AgNPs (0, 100, 300 and 500 ppm) on growth parameters of Lupinus termis L. (Reprinted from Al-Huqail et al. 2018)
9.9 Pesticide Remediation
As an alternative, AgNPs can be applied for the degradation of pesticides to overcome these problems. In modern nanotechnological research, applicability of AgNPs in pesticide mineralization is well reported. Nair and Pradeep (2003) confirmed the halocarbon mineralization and catalytic destruction by means of silver and gold nanoparticles. Manimegalai et al. (2011) reported the applicability of AgNPs for the removal of pesticides “chlorpyrifos ” and “malathion ” from water. These nanoparticles have been shown to completely remove the pesticides as it actively anchored the pesticide to its inert surfaces.
9.10 Plant Pathogen Detection
It is worth mentioning here that newly developed smart nanomaterials with special nanoscale characteristics offer tremendous breakthrough in plant pathogen detection and diagnosis technology (Khiyami et al. 2014). Striping voltammetry as an electrochemical technique can be applied to detect the metal nanoparticles directly making the assay simple to perform. Gold and silver nanoparticles can be used in these methods including different inorganic nanocrystals (ZnS, PbS and CdS) for analytic detection (Upadhyayula 2012). Schwenkbier et al. (2015) developed a helicase-dependent isothermal amplification in combination with on-chip hybridization for the detection of Phytophthora species. This approach allows efficient amplification of the yeast GTP-binding protein (Ypt1) target gene region at one constant temperature in a miniaturized heating device. The assay’s specificity was determined by on-chip DNA hybridization and subsequent AgNP deposition. The silver deposits serve as stable endpoint signals that enable the visual as well as the electrical readout. These advancements point to the direction of a near future on-site application of the combined techniques for a reliable detection of several kinds of plant pathogens.
9.11 Conclusion
Silver has been constantly superb antimicrobial (antibacterial and antifungal) and has been used for the purpose for ages. The unique physicochemical properties of AgNPs only increase the efficacy of silver. Chemical and physical methods of AgNP synthesis were being followed by several periods, but they are expensive, and the use of several toxic chemicals for their synthesis makes the biological synthesis the more desired possibility. Though microbial and plant extract sources can be used for AgNP synthesis, the easy availability, the nontoxic nature, the various options available, and the advantage of quicker synthesis make plant extracts the best and an excellent choice for biogenic AgNP synthesis. The uses of AgNPs are varied and many, but the most exploited and desired aspect is their antimicrobial and anti-inflammatory activities. The disadvantage of AgNPs is that they can induce toxicity at various degrees. It is recommended that higher concentrations of AgNPs are toxic and can cause innumerable health problems. It also revealed that the nanoparticles of silver can induce various ecological problems and disturb the ecosystem if released into the environment. Hence, this chapter concludes the application of silver nanoparticles in plant disease management, nanofertilizers, nanopesticides, pesticide remediation, and plant pathogen detections; with that there would be mechanisms devised to nullify any toxicity caused by nanosilver to humans and the environment so that the unique properties of this substance can be put to great use for human betterment without any controversies.
References
Alghuthaymi MA, Almoammar H, Rai M, Said-Galiev E, Abd-Elsalam KA (2015) Myconanoparticles: synthesis and their role in phytopathogens management. Biotechnol Biotechnol Equip 29:221–236
Al-Huqail AA, Hatata MM, AL-Huqail AA, Ibrahim MM (2018) Preparation, characterization of silver phyto nanoparticles and their impact on growth potential of Lupinus termis L. seedlings. Saudi J Biol Sci 25:313–319
Arruda SCC, Silva ALD, Galazzi RM, Azevedo RA, Arruda MAZ (2015) Nanoparticles applied to plant science: a review. Talanta 131:693–705
Aziz N, Faraz M, Pandey R, Sakir M, Fatma T, Varma A, Barman I, Prasad R (2015) Facile algae-derived route to biogenic silver nanoparticles: synthesis, antibacterial and photocatalytic properties. Langmuir 31:11605–11612. https://doi.org/10.1021/acs.langmuir.5b03081
Aziz N, Pandey R, Barman I, Prasad R (2016) Leveraging the attributes of Mucor hiemalis-derived silver nanoparticles for a synergistic broad-spectrum antimicrobial platform. Front Microbiol 7:1984. https://doi.org/10.3389/fmicb.2016.01984
Balashanmugam P, Balakumaran MD, Murugan R, Dhanapal K, Kalaichelvan PT (2016) Phytogenic synthesis of silver nanoparticles, optimization and evaluation of in vitro antifungal activity against human and plant pathogens. Microbiol Res 192:52–64
Banerjee M, Mallick S, Paul A, Chattopadhyay A, Ghosh SS (2010) Heightened reactive oxygen species generation in the antimicrobial activity of a three component iodinated chitosan-silver nanoparticle composite. Langmuir 26:5901–5908
Belava VN, Panyuta OO, Yakovleva GM, Pysmenna YM, Volkogon MV (2017) The effect of silver and copper nanoparticles on the Wheat- Pseudocercosporella herpotrichoides pathosystem. Nanoscale Res Lett 12(250):250. https://doi.org/10.1186/s11671-017-2028-6
Berahmand AA, Ghafariyan-Panahi A, Sahabi H, Feizi H, Rezvani-Moghaddam P, Shahtahmassebi N et al (2012) Effects silver nanoparticles and magnetic field on growth of fodder maize (Zea mays L.). Biol Trace Elem Res 149:419–424
Bryaskova R, Pencheva D, Nikolov S, Kantardjiev T (2011) Synthesis and comparative study on the antimicrobial activity of hybrid materials based on silver nanoparticles (AgNps) stabilized by polyvinylpyrrolidone (PVP). J Chem Biol 4(4):185–191
Chaloupka K, Malam Y, Seifalian AM (2010) Nanosilver as a new generation of nanoproduct in biomedical applications. Trends Biotechnol 28:580–588
Chen J, Li S, Luo J, Wang R, Ding W (2016) Enhancement of the antibacterial activity of silver nanoparticles against phytopathogenic bacterium Ralstonia solanacearum by stabilization. J Nanomater 2016:7135852, 15 pages. https://doi.org/10.1155/2016/7135852
Chen X, Schluesener HJ (2008) Nanosilver: a nanoproduct in medical application. Toxicol Lett 176:1–12
Chou KS, Chen CC (2007) Fabrication and characterization of silver core and porous silica shell nanocomposite particles. Microporous Mater 98:208–213
Chowdappa P, Shivakumar G (2013) Nanotechnology in crop protection: status and cope. Pest Manag Hortic Ecosys 19:131–151
Clement JL, Jarrett PS (1994) Antibacterial silver. Met Based Drugs 1:467–482
Costa MVJD, Sharma PK (2016) Effect of copper oxide nanoparticles on growth, morphology, photosynthesis, and antioxidant response in Oryza sativa. Photosynthetica 54:110–119
Danilcauk M, Lund A, Saldo J, Yamada H, Michalik J (2006) Conduction electron spin resonance of small silver particles. Spectrochimaca Acta Part A 63:189–191
Dimkpa CO, McLean JE, Martineau N, Britt DW, Haverkamp R, Anderson AJ (2013) Silver nanoparticles disrupt wheat (Triticum aestivum L.) growth in a sand matrix. Environ Sci Technol 47:1082–1090
Elbeshehy EF, Elazzazy AM, Aggelis G (2015) Silver nanoparticles synthesis mediated by new isolates of Bacillus spp., nanoparticle characterization and their activity against Bean Yellow Mosaic Virus and human pathogens. Front Microbiol 6:453
Fateixa S, Neves MC, Almeida A, Oliveira J, Trindade T (2009) Anti-fungal activity of SiO2/Ag2 S nanocomposites against Aspergillus niger. Colloids Surfaces B 74:304–308
Feng QL, Wu J, Chen GQ, Cui FZ, Kim TN, Kim JO (2008) A mechanistic study of the antibacterial effect of silver ions on Escherichia coli and Staphylococcus aureus. J Biomed Mater Res 52:662–668
Fraceto LF, Grillo R, de Medeiros GA, Scognamiglio V, Rea G, Bartolucci C (2016) Nanotechnology in agriculture: which innovation potential does it have? Front Environ Sci 4(20). https://doi.org/10.3389/fenvs.2016.00020
Gajbhiye M, Kesharwani J, Ingle A, Gade A, Rai M (2009) Fungus mediated synthesis of silver nanoparticles and their activity against pathogenic fungi in combination with fluconazole. Nanomedicine 5:382–386
González-Melendi P, Fernández-Pacheco R, Coronado MJ, Corredor E, Testillano PS, Risueño MC, Marquina C, Ibarra MR, Rubiales D, Pérez-de-Luque A (2008) Nanoparticles as smart treatment-delivery systems in plants: assessment of different techniques of microscopy for their visualization in plant tissues. Ann Bot 101:187–195
Gruyer N, Dorais M, Bastien C, Dassylva N, Triffault-Bouchet G (2013) Interaction between sliver nanoparticles and plant growth. In: International symposium on new technologies for environment control, energy-saving and crop production in greenhouse and plant factory– greensys, Jeju, Korea, 6–11 Oct 2013
Hatchett DW, Henry S (1996) Electrochemistry of sulfur adlayers on the low-index faces of silver. J Phys Chem 100:9854–9859
Jasim B, Thomas R, Mathew J, Radhakrishnan EK (2016) Plant growth and diosgenin enhancement effect of silver nanoparticles in Fenugreek (Trigonella foenumgraecum L.). Saudi Pharm J 25:443–447
Jayaseelan C, Rahaman AA, Rajkumar G, Vishnu Kirthi A, Santhoshkumar T, Marimuthu S, Bagavan A, Kamaraj C, Zahir AA, Elango G (2011) Synthesis of pediculocidal and larvicidal silver nanoparticles by leaf extract from heart leaf moonseed plant, Tinospora cordifolia Miers. Parasitol Res 109:185–194
Jo YK, Kim BH, Jung G (2009) Antifungal activity of silver ions and nanoparticles on phytopathogenic fungi. Plant Dis 93(10):1037–1043
Joshi N, Jain N, Pathak A, Singh J, Prasad R, Upadhyaya CP (2018) Biosynthesis of silver nanoparticles using Carissa carandas berries and its potential antibacterial activities. J Sol-Gel Sci Techn https://doi.org/10.1007/s10971-018-4666-2
Kamran S, Forogh M, Mahtab E, Mohammad A (2011) In vitro antibacterial activity of nanomaterials for using in tobacco plants tissue culture. World Acade Sci Eng Technol 79:372–373
Khan MR, Rizvi TF (2014) Nanotechnology: scope and application in plant disease management. Plant Pathol J 13:214–231
Khiyami MA, Almoammar H, Awad YM, Alghuthaym MA et al (2014) Plant pathogen nanodiagnostic techniques: forthcoming changes? Biotechnol Biotechnol Equip 28(5):775–785
Kim H, Kang H, Chu G, Byun H (2008) Antifungal effectiveness of nanosilver colloid against rose powdery mildew in greenhouses. Solid State Phenomenon 135:15–18
Kim JS, Kuk E, Yu K, Kim JH, Park SJ, Lee HJ, Kim SH, Park YK, Park YH, Wang C-Y, Kim YK, Lee YS, Jeong DH, Cho MH (2007) Antimicrobial effects of silver nanoparticles. Nanomedicine 3:95–101
Kim SW, Jung JH, Lamsal K, Kim YS, Min JS, Lee YS (2012) Antifungal effects of silver nanoparticles (AgNPs) against various plant pathogenic fungi. Mycobiology 40(1):53–58
Krishnaraj C, Jagan EG, Ramachandran R, Abirami SM, Mohan N, Kalaichelvan PT (2012) Effect of biologically synthesized silver nanoparticles on Bacopa monnieri (Linn.) Wettst. Plant growth metabolism. Process Biochem 47(4):51–658
Lamsal K, Kim SW, Jung JH, Kim YS, Kim KS, Lee YS (2011) Inhibition effects of silver nanoparticles against powdery mildews on cucumber and pumpkin. Mycobiology 39(1):26–32
Lemire JA, Harrison JJ, Turner RJ (2013) Antimicrobial activity of metals: mechanisms, molecular targets and applications. Nat Rev Microbiol 11(6):371–384
Li G, He D, Qian Y, Guan B, Gao S, Cui Y, Yokoyama K, Wang L (2012) Fungus-mediated green synthesis of silver nanoparticles using Aspergillus terreus. Int J Mol Sci 3:466–476
Lü JM, Wang X, Marin-Muller C, Wang H, Lin PH, Yao Q, Chen C (2009) Current advances in research and clinical applications of PLGA based Nanotechnology. Expert Rev Mol Diagn 9:325–341
Manimegalai G, Kumar SS, Sharma C (2011) Pesticide mineralization in water using silver nanoparticles. Int J Chem Sci 9:1463–1471
Marimuthu S, Rahuman AA, Rajkumar G, Santhoshkumar T, Kirthi AV, Jayaseelan C, Bagavan A, Zahir AA, Elango G, Kamaraj C (2011) Evaluation of green synthesized silver nanoparticles against parasites. Parasitol Res 108:1541–1549
Matsumura Y, Yoshikata K, Kunisaki S, Tsuchido T (2003) Mode of bacterial action of silver zeolite and its comparison with that of silver nitrate. Appl Environ Microbiol 69:4278–4281
Min JS, Kim KS, Kim SW, Jung JH, Lamsal K, Kim SB, Jung M, Lee YS (2009) Effects of colloidal silver nanoparticles on sclerotium-forming phytopathogenic fungi. Plant Pathol J 25:376–380
Mirzajani F, Askari H, Hamzelou S, Farzaneh M, Ghassempour A (2013) Effect of silver nanoparticles on Oryza sativa L. and its rhizosphere bacteria. Ecotoxicol Environ Saf 88:48–54
Mondal NK, Chowdhury A, Dey U et al (2014) Green synthesis of silver nanoparticles and its application for mosquito control. Asian Pac J Trop Dis 4:S204–S210
Nair S, Pradeep T (2003) Halocarbon mineralization and catalytic destruction by metal nanoparticles. Curr Sci 84:12
Nangmenyi G, Economy J (2009) Nonmetallic particles for oligodynamic microbial disinfection. In: Street A, Sustich R, Duncan J, Savage N (eds) Nanotechnol application for clean water. William Andrew, Norwich, NY, pp 3–15
Narayanan KB, Sakthivel N (2010) Biological synthesis of metal nanoparticles by microbes. Adv Colloid Interf Sci 156:1–13
Ocsoy I, Paret ML, Ocsoy MA, Kunwar S, Chen T, You M, Tan W (2013) Nanotechnology in plant disease management: DNA-directed silver nanoparticles on graphene oxide as an antibacterial against Xanthomonas perforans. ACS Nano 7(10):8972–8980
Oh SD, Lee S, Choi SH, Lee IS, Lee YM, Chun JH, Park HJ (2006) Synthesis of Ag and Ag-SiO2abil nanoparticles by у-irradiation and their antibacterial and antifungal efficiency against Salmonellaenteric serovar Typhimurium and Botrytis cinerea. Colloids Surf A 275:228–233
Ouda SM (2014) Antifungal activity of silver and copper nanoparticles on two plant pathogens, Alternaria alternata and Botrytis cinerea. Res J Microbiol 9(1):34–42
Pal S, Tak UK, Song JM (2007) Does the antibacterial activity of silver nanoparticles depend on the shape of the nanoparticle? A study of the gram-negative bacterium Escherichia coli. App Environ Microbiol 73:1712–1720
Pallavi MCM, Srivastava R, Arora S, Sharma AK (2016) Impact assessment of silver nanoparticles on plant growth and soil bacterial diversity. Biotech 6(254):254. https://doi.org/10.1007/s13205-016-0567-7
Panáček A, Kolář M, Večeřová R, Prucek R, Soukupová J, Kryštof V, Park HJ, Kim SH, Kim HJ, Choi SH (2006) A new composition of nanosized silica-silver for control of various plant diseases. Plant Pathol J 22:295–302
Panyala NR, Pena Mendez EM, Havel J (2008) Silver or silver nanoparticle: a hazardous treat to the environment and human health? J Appl Med 6:117–129
Papp I, Sieben C, Ludwig K, Roskamp M, Böttcher C, Schlecht S et al (2010) Inhibition of influenza virus infection by multivalent sialic-acid-functionalized gold nanoparticles. Small 6:2900–2906
Parashar UK, Saxena SP, Srivastava A (2009) Bioinspired synthesis of silver nanoparticles. Dig J Nanomat Biostruct 4:159–166
Park H-J, Kim SH, Kim HJ, Choi S-H (2006) A new composition of nanosized silica-silver for control of various plant diseases. Plant Pathol J 22(3):295–302
Pérez-de-Luque A, Rubiales D (2009) Nanotechnology for parasitic plant control. Pest Manag Sci 65:540–545
Prasad R, Swamy VS (2013) Antibacterial activity of silver nanoparticles synthesized by bark extract of Syzygium cumini. J Nanopart 2013:1. https://doi.org/10.1155/2013/431218
Prasad R (2014) Synthesis of silver nanoparticles in photosynthetic plants. J Nanopart 963961., https://doi.org/10.1155/2014/963961:1
Prasad R, Bhattacharyya A, Nguyen QD (2017a) Nanotechnology in sustainable agriculture: recent developments, challenges and perspectives. Front Microbiol 8:1014. https://doi.org/10.3389/fmicb.2017.01014
Prasad R, Gupta N, Kumar M, Kumar V, Wang S, Abd-Elsalam KA (2017b) Nanomaterials act as plant defense mechanism. In: Prasad R, Kumar V, Kumar M (eds) Nanotechnology. Springer, Singapore, pp 253–269
Prasad R, Kumar V, Prasad KS (2014) Nanotechnology in sustainable agriculture: present concerns and future aspects. Afr J Biotechnol 13(6):705–713
Prasad R, Pandey R, Barman I (2016) Engineering tailored nanoparticles with microbes: quo vadis. WIREs Nanomed Nanobiotechnol 8:316–330. https://doi.org/10.1002/wnan.1363
Radwan DEM, Fayez KA, Mahmoud SY, Hamad A (2008) Protective action of salicylic acid against bean yellow mosaic virus infection in Vicia faba leaves. J Plant Physiol 165:845–857
Rai M, Ingle A (2012) Role of nanotechnology in agriculture with special reference to management of insect pests. Appl Microbiol Biotechnol 94:287–293
Raza MA, Kanwal Z, Rauf A, Sabri AN, Riaz S, Shahzad Naseem S (2017) Size- and shape-dependent antibacterial studies of silver nanoparticles synthesized by wet chemical routes. Nano 6(74):1–15
Rezvani N, Sorooshzadeh A, Farhadi N (2012) Effect of nano-silver on growth of saffron in flooding stress. World Acad Sci Eng Technol 1:517–522
Roe D, Karandikar B, Bonn-Savage N, Gibbins B, Roullet JB (2008) Antimicrobial surface functionalization of plastic catheters by silver nanoparticles. J Antimicrob Chemother 61:869–887
Rouhani M, Samih MA, Kalantri S (2012) Insecticidal effect of silica and silver nanoparticles on the cowpea seed beetle, Callosobruchus maculatus F (Col: Bruchidae). J Entomol Res 4:297–305
Salama HMH (2012) Effects of silver nanoparticles in some crop plants, common bean (Phaseolus vulgaris L.) and corn (Zea mays L.). Int Res J Biotech 3(10):190–197
Savithramma N, Ankanna S, Bhumi G (2012) Effect of nanoparticles on seed germination and seedling growth of Boswellia ovalifoliolata an endemic and endangered medicinal tree taxon. Nano Vision 2:61–68
Schwenkbier L, Pollok S, König S, Urban M, Werres S, Dana Cialla-May D, Karina Weber K, Popp J (2015) Towards on-site testing of Phytophthora species. Anal Methods 7:211–217
Servin A, Elmer W, Mukherjee A, De La Torre-Roche R, Hamdi H, White JC, Bindraban P, Dimkpa C (2015) A review of the use of engineered nanomaterials to suppress plant disease and enhance crop yield. J Nanopart Res 17:1–21
Sharma P, Bhatt D, Zaidi MG, Saradhi PP, Khanna PK, Arora S (2012) Silver nanoparticle mediated enhancement in growth and antioxidant status of Brassica juncea. Appl Biochem Biotechnol 167:2225–2233
Shrivastava S, Bera T, Roy A, Singh G, Ramachandrarao P, Dash D (2007) Characterization of enhanced antibacterial effects of novel silver nanoparticles. Nanotechnology 18:1–9
Singh S, Singh BK, Yadav SM, Gupta AK (2015) Applications of nanotechnology in agricultural and their role in disease management. Res J Nanosci Nanotechnol 5:1–5
Sondi I, Salopek-Sondi B (2004) Silver nanoparticles as antimicrobial agent: a case study on E. coli as a model for gram-negative bacteria. J Colloid Interface Sci 275:177–182
Soni N, Prakash S (2015) Antimicrobial and mosquitocidal activity of microbial synthesized silver nanoparticles. Parasitol Res 114:1023–1030
Swamy VS, Prasad R (2012) Green synthesis of silver nanoparticles from the leaf extract of Santalum album and its antimicrobial activity. J Optoelectron Biom Mater 4(3):53–59
Suman TY, Elumali D, Kaleena PK (2013) GCMS analysis of bioactive components and synthesis of silver nanoparticle using Ammannia baccifera aerial extract and its larvicidal activity against malaria and fiariasis vectors. Ind Crop Prod 47:239–245
Taniguchi N (1974) On the basic concept of ‘nano-technology’. Proceedings of the international conference on production engineering Tokyo, Part II; Tokyo: Japan Soc Precision Engineering. pp. 18–23
Tripathi DK, Singh S, Singh S, Srivastava PK, Singh VP, Singh S et al (2017) Nitric oxide alleviates silver nanoparticles (AgNps)-induced phytotoxicity in Pisum sativum seedlings. Plant Physiol Biochem 110:167–177
Tripathi DK, Singh VP, Prasad SM, Chauhan DK, Dubey NK (2015) Silicon nanoparticles (SiNp) alleviate chromium (VI) phytotoxicity in Pisum sativum (L.) seedlings. Plant Physiol Biochem 96:189–198
Tsuji K (2001) Microencapsulation of pesticides and their improved handling safety. J Microencapsul 18:137–147
Upadhyayula VKK (2012) Functionalized gold nanoparticle supported sensory mechanisms applied in detection of chemical and biological threat agents: a review. Anal Chim Acta 715:1–18
Vinković T, Novák O, Strnad M, Goessler W, Jurašin DD, Paradiković N, Vrček IV (2017) Cytokinin response in pepper plants (Capsicum annuum L.) exposed to silver nanoparticles. Environ Res 156:10–18
Winbo Ma (2011) How do plants fight disease? Breakthrough research by UC Riverside plant pathologist offers a clue. http://newsroom.ucr.edu/2587
Yin L, Colman BP, McGill BM, Wright JP, Bernhardt ES (2012) Effects of silver nanoparticle exposure on germination and early growth of eleven wetland plants. PLoS One 7:e47674. https://doi.org/10.1371/journal.pone.0047674
Zeng F, Hou C, Wu SZ, Liu XX, Tong Z, Yu SN (2007) Silver nanoparticles directly formed on natural macroporous matrix and their anti-microbial activities. Nanotechnology 18:1–8
Author information
Authors and Affiliations
Editor information
Editors and Affiliations
Rights and permissions
Copyright information
© 2018 Springer International Publishing AG, part of Springer Nature
About this chapter
Cite this chapter
Gupta, N., Upadhyaya, C.P., Singh, A., Abd-Elsalam, K.A., Prasad, R. (2018). Applications of Silver Nanoparticles in Plant Protection. In: Abd-Elsalam, K., Prasad, R. (eds) Nanobiotechnology Applications in Plant Protection. Nanotechnology in the Life Sciences. Springer, Cham. https://doi.org/10.1007/978-3-319-91161-8_9
Download citation
DOI: https://doi.org/10.1007/978-3-319-91161-8_9
Published:
Publisher Name: Springer, Cham
Print ISBN: 978-3-319-91160-1
Online ISBN: 978-3-319-91161-8
eBook Packages: Biomedical and Life SciencesBiomedical and Life Sciences (R0)