Abstract
The use of stent-based endovascular electrode arrays is a recent approach to brain–computer interfaces (BCIs). These arrays can record neuronal activity from within the large blood vessels of the brain following placement via catheterization. Accessing the brain without the need for open craniotomy is appealing and may inspire future generations of endovascular BCI devices. Here we review some of the bioethical considerations arising from the adoption of endovascular electrode arrays for BCIs. We consider the safety and efficacy of endovascular arrays in comparison to the risks/benefits of other approaches to BCI. We explore the bioethical implications of their permanency as these implants are designed to remain in place indefinitely following endothelialization. Additionally, we consider how endovascular approaches to BCI might affect the informed consent process and discuss how the publicity surrounding BCIs may influence the expectations of a new generation of endovascular BCIs.
Access provided by Autonomous University of Puebla. Download chapter PDF
Similar content being viewed by others
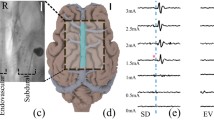
Keywords
1 Introduction
A recent early feasibility trial by Oxley and colleagues [1] demonstrated effective control of a personal computer using a brain–computer interface (BCI) in two individuals with paralysis due to amyotrophic lateral sclerosis. The trial was noteworthy as it provided the first demonstration of a working BCI using brain signals recorded endovascularly in humans. This approach involved placement of a stent into the superior sagittal sinus adjacent to the motor cortices via a catheter accessing the jugular vein. The stent housed sixteen recording electrodes, thus providing access to electrocorticographic recordings from within the brain without the need for craniotomy. This endovascular BCI arrangement is illustrated in Fig. 1.
Panel a illustrates a stent-based endovascular electrode array deployed within a cortical vein. Once placed, the stent may be incorporated into the wall of the blood vessel via endothelialization. Panel b depicts a patient using an endovascular BCI system. Such systems may be fully implanted with recorded signals transmitted wirelessly out of the body to control various devices such as a personal computer
While the use of endovascular electrode arrays within BCI systems is a recent development, the first demonstration of endovascular electrophysiological recordings took place almost four decades ago [2]. Development of endovascular recording technologies has since progressed from wire electrodes to nanowire and catheter electrodes and finally stent-based electrode arrays [3]. Coupled with an unmet clinical need, these technological advances have attracted significant commercial investment, putting fully implantable BCIs such as the Stentrode™ system used by Oxley et al. [1] on the path to market entry and clinical adoption [4].
In this chapter, we explore some of the bioethical considerations specific to the use of endovascular electrode arrays for BCI. We first examine the safety of endovascular electrode arrays including how they compare to previous approaches to BCI and what unique risks they might pose. As risks should be weighed against the potential for benefit, we also present the efficacy of endovascular recording devices. A unique consideration of endovascular electrode arrays among approaches to BCI is that implantation may be permanent. We therefore explore how this might affect bioethical considerations of BCIs that rely upon this method of recording from the brain. The consequences of endovascular approaches to BCI on the informed consent process are briefly considered. Finally, we also reflect on the impact the recent publicity surrounding BCIs may have on the immediate expectations of newly developed endovascular BCIs and how commercial ventures in this technology may differ from academic pursuits. Our discussion focuses on the bioethical impact of using endovascular recording electrodes within BCIs from a hardware perspective, but does not offer extensive consideration of the applications of endovascular BCI as the initial applications may be similar to BCIs using other sensor modalities.
2 Safety of Endovascular BCIs
Endovascular BCIs are novel among BCIs as they are implanted via a minimally invasive neurointerventional procedure and might, therefore, pose a substantially different risk profile to existing BCI procedural approaches. Rather than requiring burr hole craniotomies for implantation, endovascular BCIs require that a small incision be made to gain entry into a blood vessel, such as the jugular vein. A guide catheter is then advanced to the implant location where the stent is deployed (Fig. 1a, and the trailing lead is tunneled and connected to an internal telemetry unit (ITU) within a subcutaneous pocket in the chest [1] (Fig. 1b)). This approach to BCI placement involves unique safety challenges, and the potential for benefits compared to other BCI approaches should be assessed under a bioethical lens.
All invasive BCIs carry risks for infection, hemorrhage, and other hardware related complications. Although there is limited literature discussing the safety profile of endovascular BCIs [5], endovascular stenting is considered standard of care for idiopathic intracranial hypertension (IIH), thrombectomy, vascular malformation, and aneurysms [6, 7]. As such, we can make inferences regarding the potential for adverse events associated with stent based endovascular BCI implantation and compare them to that of other invasive systems.
Infection can be a serious complication following BCI implantation, as it can result in meningitis, brain abscesses, septic emboli, or other life-threatening consequences. The infection risk for subdural electrode arrays has been reported to be 2.3% [8]. Similarly, infections have been found in 1–5% of patients after implantation of depth electrodes [9]. In a systematic review including 174 deep brain stimulation (DBS) articles that reported data on infection, the incidence rate of infection was identified as 3.79%. Of the 104 papers that included the location of the infection, 44.2% occurred at the site of the internal pulse generator, 17.8% occurred at the scalp or burr hole, 13.6% occurred at the connector and extension cable, and 11.1% occurred in the brain along the electrode lead [10]. The procedural-related risk of infection for the Neuropace RNS system, a BCI with both ECoG and depth electrode components, has been reported to be 4.1%, with an overall incidence rate of infection of 12.1% over the cumulative 1895 patient-implantation years [11]. Alternatively, infections associated with venous sinus stenting for IIH are rare, with only one case of infection identified in several systematic reviews [12,13,14], which was a urinary tract infection [15]. However, the only existing report of a stent based BCI technology used in humans [1] included a hardware piece, an ITU, that is comparable to that of the internal pulse generator in DBS systems and may carry a similar infection rate when placed in the chest. It is difficult to make a direct comparison to the infection rate of Utah arrays due to limited literature on complications and adverse events in chronically implanted Utah arrays [10].
Hemorrhaging after a BCI implant procedure can also result in serious life-threatening complications including neurological damage. Non-seizure-related hemorrhage was reported in 2.7% of patients implanted with the Neuropace RNS system [11]. Bullard et al. [10] reported a similar incidence rate of 2.9% for DBS systems with 86.9% being intracerebral hemorrhages. A meta-analysis and systematic review reporting on 21 studies and 2542 patients with subdural electrode arrays noted a slightly higher incidence rate of 4.0% [8]. Rates of hemorrhages may be slightly lower for endovascular stenting procedures in clinical settings. For the endovascular treatment of venous sinus stenosis in IIH, a systematic review comprising of 17 studies with a total of 185 patients reported hemorrhages in 3 patients (1.6%) [14]. It is worth noting that humans implanted with the Stentrode™ endovascular BCI underwent dual antiplatelet therapy [1] which might increase the risk of systemic hemorrhage for those not already taking this medication [16, 17].
Other hardware related complications have been reported including device migration. In the endovascular treatment of intracranial aneurysms, migration of a coil or stent occurs in 2–6% of cases [18, 19]. For IIH, Teleb et al. [20] reported a single intraprocedural event of stent migration out of 25 procedures. These rates are comparable to that of DBS hardware complications; Bullard et al. [10] reported that lead migration occurred 3.49% of the time, followed by lead fractures (2.53%), internal pulse generator malfunctions (2.33%), and extension cable malfunctions (1.95%). Events such as these may require additional surgical procedures or removal which may pose additional surgical risks [21].
Endovascular BCIs may also carry additional risks including perforation or occlusion of the vein/artery, stenosis, and catheter related complications. For instance, intraprocedural vessel perforation during stent retriever thrombectomy occurred in 16 of 1599 cases [22]. Occlusion or perforation of the vessel can lead to hemorrhage or stroke [23]. At the time of writing this, endovascular BCI technologies have used venous implantation routes, and it is important to consider the risk profile of venous versus arterial implantation routes to understand why this is the case. Alawieh et al. [24] noted the advantage of the venous route by highlighting its lack of smooth muscle layers and lower likelihood of provoking vasospasm. As recording technology changes over time, there may be a functional advantage of using an arterial route if it allows an endovascular recording technology to achieve a location that is closer to the target brain region than the venous route. Should this occur, a separate risk-benefit analysis will need to be conducted. Also, the additional procedural risks associated with stenosis must be considered, as in IIH. Starke et al. [14] reported in-stent stenosis in 6 patients (3.4%) and stent-adjacent stenosis in 19 patients (11.4%), with 10 patients requiring restenting. Finally, the diameter of the catheter and vessels accessed may also influence the rates of adverse events. For example, venous dissection, subdural hemorrhage, and acute thrombus formation occurred when using a 5-Fr or 6-Fr catheter in animal testing, whereas no complications occurred when using a 4-Fr or 2-Fr catheter. In some cases, these complications led to non-recovery after anesthesia [25].
The first in-human study of the Stentrode™ found no instances of infection, device migration, stenosis, or device related adverse events. They did however find a post-procedural-related adverse event of syncope associated with two sinus pauses which was attributed to post-procedural vagal tone and required no intervention. It is important to note that there were only two participants involved in this study [1]. A clinical trial with a larger sample size needs to be performed before the safety profile of this stent based endovascular BCI can be established.
Endovascular stent electrodes may also have several advantages. For example, they circumvent the need for craniotomy which can open additional avenues of care for individuals with stroke or traumatic brain injury [24]. Also, considering the relatively smaller scale and shorter duration of surgery, implantation of endovascular BCIs would require smaller total doses of anesthesia. This would likely limit surgical risk for individuals with respiratory compromise. Additionally, the implantation of other invasive BCI systems is still a niche skill, whereas the advent of thrombectomy in stroke has made stent placement a ubiquitous skillset for interventional neurosurgeons [26,27,28]. As such, the surgical risk should be adjusted accordingly.
Finally, non-invasive BCI systems including scalp electroencephalography, magnetoencephalography, and functional near-infrared spectroscopy-based devices pose lower procedural risks than invasive BCIs as they do not penetrate the skin [23]. However, non-procedural risks should also be considered. Unlike endovascular BCIs, some users have found headsets used for non-invasive BCI to be uncomfortable [29, 30]. Application of these systems also requires assistance which can be burdensome and may increase feelings of dependency [31]. Their visible machinery and medical connotation may also contribute to stigma [32], thereby affecting patients’ self-consciousness, willingness to socialize, and social status. Conversely, endovascular BCIs may be fully implantable and may add less to stigma, burden, and feelings of frustration.
A summary of our safety considerations is provided in Table 1. While the short- and long-term safety profile of endovascular BCIs in humans has yet to be established, endovascular stenting for a range of indications has shown slightly lower rates of infection and hemorrhage compared to that of other invasive hardware. Circumventing the need for craniotomy during implantation of an endovascular BCI may also play a role in lowering the rate of these complications and avoiding other procedural risks. Larger human clinical trials investigating the rate of new endovascular BCI-specific safety concerns and advantages are required to develop pre- and post-implant mitigation procedures and enable comprehensive risk-benefit analyses.
3 Efficacy of Endovascular BCIs
The overall effectiveness and benefit derived from a BCI may depend on several factors including the signal decoding algorithms, device software, system usability, ergonomics, and the design/engineering of any effector. Additionally, the type of sensor used to record the brain signal may also impact BCI effectiveness. The reliability, the number of outputs, and customizability of a BCI may all be affected by inadequate recording quality. Therefore, the efficacy of endovascular electrodes for recording neural signals is worth considering within our bioethics discussion as it will impact the risk-benefit profile of any BCI that uses this technology.
3.1 Comparisons with Other Sensor Types
Comparisons between endovascular and scalp EEG were included in the earliest demonstrations of endovascular recordings. Penn and colleagues [2] demonstrated that endovascular EEG recordings were able to detect localized activity in intracerebral structures that were not accessible to routine scalp EEG. Their report concluded that the endovascular technique offered advantages over non-invasive scalp EEG recordings for the detection of clinically relevant, paroxysmal EEG activity. Comparing endovascular and scalp EEG signal quality, Nakase et al. [33] reported a 2–5 times stronger EEG voltage potential in endovascular EEG compared to scalp EEG. Similarly, Stoeter et al. [34] found higher peak-to-peak amplitudes of both somatosensory and auditory evoked potentials when comparing concurrent endovascular and scalp EEG recordings in patients undergoing interventional angiographies. These results indicated that endovascular EEG signals were subject to less attenuation than extracranial recordings. More recently, He et al. [35] compared visual and auditory evoked potentials recorded using both endovascular and scalp EEG in rabbits. Their results confirmed that higher amplitudes of evoked responses can be observed in endovascular recordings and indicated that their endovascular recordings were of a higher quality than their scalp recordings with up to 100 times better signal-to-noise ratio. These results were shown to be unrelated to non-neural biological signals or differences in electrode materials.
Endovascular EEG recordings have also been compared to surface ECoG. Nakase and colleagues [33] found that interictal spike discharges were simultaneously visible in concurrent endovascular and subdural strip electrode recordings from the medial temporal lobe in three patients with epilepsy. Likewise, Bower et al. [36] found that epileptiform spikes recorded by endovascular and subdural electrodes were similar in both amplitude and waveshape. Here, recordings were collected from pigs using endovascular electrodes within the superior sagittal sinus and subdural electrode arrays placed in parallel, and epileptic activity was induced via direct cortical injection of penicillin. Using low-amplitude sinusoidal currents passed between opposing corners of the subdural grid, these authors reported that the frequency response of the intravascular recordings were reduced by 11.0% and 24.2% at 30 Hz and 100 Hz, respectively, relative to the recording electrodes of the subdural array [36]. Nevertheless, the endovascular microelectrodes were able to record the low-amplitude, high-frequency signals arising from spatially localized seizure activity that were only observed by the microelectrodes within the subdural grid and missed by the standard clinical macroelectrodes of the subdural array.
Oxley et al. [37] compared the spectral content of recordings from their stent-based endovascular electrode array (Stentrode™) with contemporaneous recordings collected using commercially available subdural and epidural arrays in sheep. No difference in absolute power was found in mu, beta or low gamma bands between the three array types, whereas higher power was seen in the higher gamma bands in the subdural recordings compared to both the endovascular and epidural recordings, which were similar. Maximum bandwidth was also highest in the subdural array and similar between the endovascular and epidural devices.
John et al. [38] also compared the signal quality of the Stentrode™ array with both subdural and epidural ECoG interfaces. Again, sheep were used, and measurements were recorded 3–4 weeks after electrode placement to allow for stabilization of signal quality following endothelialization of the stent-based electrode array. The authors found no significant effect of recording location or electrode sizes on the bandwidth of the recorded signal, indicating the quantity of information that can be obtained from endovascular, subdural, and epidural electrode arrays is similar. There was also no significant effect of electrode location or size on the signal-to-noise ratio indicating similar signal quality. However, the variability between subdural electrodes was higher, meaning select subdural electrodes achieved a higher signal-to-noise ratio than the endovascular electrodes. Finally, the difference in spatial resolution between the arrays was frequency dependent between 8 and 180 Hz, differing only in the lowest frequency band investigated (8–24 Hz), where subdural electrode arrays produced the best spatial resolution.
In the same study, John et al. [38] demonstrated that the similar signal qualities between the epidural, subdural, and endovascular arrays meant that there was no appreciable difference in single-trial decoding performance (classification of the presence or absence of an evoked potential). In addition, Forsyth et al. [39] compared decoding performance between Stentrode™, subdural, and epidural arrays implanted in a sheep trained to perform left and right head movements in response to an external stimulus. Classification of these movements was computed offline. Using 50% of the trials for training data and 50% for test data, results demonstrated that the epidural array had a slightly lower classification accuracy (80%) than the endovascular and subdural arrays (both 85%) when comparing movement vs. rest. All were higher than the minimum accuracy required to exceed chance, which was dependent on the number of trials used to test the classification. When comparing left vs. right movements, classification accuracies from the endovascular (58%), subdural (55%), and epidural (51%) arrays were again similar, but only the endovascular array distinguished between left and right movements at a higher rate than chance.
To the authors knowledge, no direct comparisons in signal quality or classification performance between endovascular and penetrative electrode arrays have been made. This may be more complicated as these sensor types might record different neural signals for classification. For example, upon implantation, penetrative microelectrode arrays offer spike recordings from individual neurons. This resolution of neural activity is not available to endovascular electrode arrays, which record synchronized post-synaptic potentials from within the wall of the blood vessel. However, over time, encapsulation of microelectrodes may occur in response to the damage caused to the surrounding tissues during implantation. Thereafter, microelectrode arrays may be limited to recording local field potentials [40] making comparisons of endovascular and microelectrode arrays more valid.
3.2 Longitudinal Assessments
Oxley et al. [37] performed a series of experiments over a 28-day period following implantation of their Stentrode™ into a superficial cortical vein overlying the motor cortex in sheep. This time window allowed for examination of changes in recording signal quality as the stent was incorporated into the blood vessel, its permanent recording location, via endothelialization. Direct median nerve stimulation was used to elicit somatosensory evoked potentials, which were detected in 98% of all functional channels. This increased from approximately 50% on day 1 post-implant to 92% by day 4. The peak-to-peak amplitudes were unchanged over the 28-day study period indicating stability of the recorded signals over this time. Anesthesia-induced theta burst suppression (see [41]) was used to examine changes in recording sensitivity. Higher burst-suppression ratios at 1 month versus baseline indicated an increase in sensitivity over this time. In 10 sheep, neural recordings were continued for up to 190 days. Maximum bandwidth was recorded and found to be stable throughout, indicating long-term viability of the Stentrode™. Maximum bandwidth was lower than contemporaneous subdural ECoG recordings but similar to epidural recordings.
In another study, Opie et al. [42] examined the electrical signals recorded by the same Stentrode™ device following implantation within the superior sagittal sinus of 15 sheep. Using electrochemical impedance spectroscopy, this study found that 1 kHz impedances were consistent across the 91-day examination period, and capacitance stabilized after approximately 8 days after initial increases accompanying the early stages of endothelialization. Opie and colleagues [43] also reported that the bandwidth of the recordings (~193 Hz) was consistent both between sheep and over time across their 6-month study period.
More recently, a study with human subjects using the Stentrode™ for BCI demonstrated effective control of a computer up to 238 days following implantation [1]. Signal decoder settings were fixed as early as the third training session (53 days post-implantation) and, thereafter, required only calibration of the feature normalization constants, which took 30 seconds to complete.
These results demonstrate the potential for chronically stable endovascular recordings from within the superior sagittal sinus, adjacent to the motor cortices. Signal quality may even improve with biological activity at the electrode–tissue interface as the stent carrying the electrodes is integrated into the wall of the blood vessel via endothelialization. This contrasts with other invasive electrode arrays, where numerous biological reactions to the implanted device may occur often resulting in a decrease in signal quality [44]. These reactions are commonly cited as a limitation to the use of these BCIs. However, it should be noted that the length of study of recent endovascular array based BCIs does not yet exceed previous investigations that have demonstrated high utility of both surface ECoG and microelectrode based BCIs after 3–5 years despite degradation of the underlying signals [45, 46].
3.3 Endovascular Neurostimulation
The potential to use endovascular electrodes for brain stimulation has also been explored. Gerboni et al. [47] demonstrated cortical activation in response to monopolar, endovascular electrical stimulation in sheep. Clear evoked potentials were demonstrated that were graded in relation to the current applied. No observable responses were recorded after culling the animal, verifying the neural origin of the responses. In another proof-of-concept study, Opie et al. [43] elicited motor responses in the lip, face, jaw, neck, and limbs of 25 sheep using cortical stimulation delivered via endovascular electrodes. The observed responses were similar to those elicited using invasive penetrating and subdural arrays. These results demonstrated the ability of endovascular electrodes to deliver focal stimulation to neural tissue without open brain surgery.
Endovascular electrode arrays offer superior signal quality compared to non-invasive EEG and comparable recordings to existing subdural ECoG arrays. Early evidence indicates that these high-quality signals may be stable over time when using stent-based endovascular arrays that are incorporated into the wall of the blood vessel. Thus, with some potential safety advantages compared to other invasive approaches that require craniotomy, endovascular electrodes may offer an advantageous risk-benefit profile for certain uses. Moreover, in the future, access to smaller blood vessels may enable recordings from regions of the brain not readily accessible using surface ECoG or microelectrode arrays, and without the neural damage caused by depth electrodes. The potential for neurostimulation in these brain regions may also increase the utility of endovascular electrode arrays.
4 Endovascular BCI Permanency
The implantation of endovascular BCIs is intended to be permanent. Stents carrying the electrode arrays are incorporated into the wall of the blood vessel via endothelialization. Partial endothelialization may be observed within days and may be complete within 4 weeks of placement [37, 48]. Thereafter, removal from the brain would require major vascular surgery. The permanency of endovascular BCIs raises important bioethical considerations for their development and use.
First-in-human trials of endovascular BCIs have begun [1]. This research required participants’ consent to the permanent implantation of the electrode array in their brains. This is a departure from previous invasive BCI research that has mostly relied on the temporary implantation of electrode arrays for clinical purposes as a window of opportunity. For example, the use of ECoG arrays in perioperative observation for epilepsy surgery has enabled much of the opportunity for invasive BCI research [49,50,51]. Whereas, to the authors’ knowledge, only one case of a chronically implanted ECoG-based BCI has been reported in the literature [45, 52]. Similarly, most microelectrode array implants have also been temporarily implanted to study epilepsy, anesthesia, cognition, memory, or language [10]. Relatively few have been chronically implanted. In their review, Bullard and colleagues reported that only 18 of 48 people implanted with the Utah array were implanted for longer than 30 days [10]. Accordingly, bioethical considerations regarding the permanent implantation of investigational BCI devices has received a paucity of attention in the literature. Here we consider the ethical impact of BCI permanency using the four principles of bioethics as a framework: non-maleficence, beneficence, autonomy, and justice.
4.1 Nonmaleficence
At the early feasibility phase of research, the risks of any investigational BCI are uncertain. Various safety and biocompatibility data need first be collected in bench top and animal testing. This process will be mostly unchanged by the permanency of a BCI, although longer durations of monitoring during animal testing may be warranted prior to human studies. The risk of a malfunction or toxicity may increase with the duration of implantation, which would become the lifetime of the patient when using endovascular BCIs. Moreover, the consequences of adverse reactions to an endovascular BCI may be greater if they necessitate a major neurovascular surgery to remove the device. However, the biggest difference in the risk of harm between permanent and temporary implants may be the potential for indirect harm to permanently implanted patients. For example, if the endovascular BCI is not MRI compatible, then implantation would preclude the patient from undergoing an MRI scan for any future medical need.
In addition to the risks being uncertain, the efficacy of investigational BCIs for individuals with different neurological deficits will be unknown. Research subjects who fail to learn how to operate their BCI as intended (e.g., control a computer cursor or prosthetic arm) may suffer psychological harm due to their failure to meet perceived expectations or achieve an expected therapeutic benefit [53]. This psychological harm may be amplified or prolonged if the user is unable to have the ineffective device removed from their person. Thus, carefully considered selection criteria may become even more essential when admitting study subjects to a trial of an investigational endovascular BCI.
Permanent implantation of a BCI could also lead to reductions in harm. Invasive BCIs intended to be temporary would necessitate a second surgery for explantation. This would have associated risks that might exceed the risks of not removing the device. This exposure to potential harm may be unwarranted if the patient does not wish the device to be removed and there is low anticipated risk from the device remaining in place.
Finally, the permanency of endovascular BCIs may further blur the murky distinction between BCI research and treatment [54]. Research has a finite timeframe. Outcome measures must be collected at preset times following implantation. However, permanently implanted BCIs will remain in place beyond any scheduled data collection. Researchers may need to incorporate a plan for continued care beyond the collection of primary outcome measures.
4.2 Beneficence
BCIs hold the potential for myriad benefits for individuals with a wide variety of neurological conditions. For example, BCIs may bestow a sense of agency, increase the opportunity for social participation, and have a positive effect on a user’s self-image [55]. Temporary implantation of investigational BCIs may offer benefits to the patient, only for these to be taken away when the device is explanted. This practice could arguably be seen as unethical. It may at least be unattractive to potential study participants who may not want to end their participation [55]. Although research with an indefinite timeline raises concerns of exploitation, in exploratory studies where the goal of the research has not yet been fully realized, the subject does not wish to end their participation, and the research continues to progress, it may be appropriate to continue participation in the research until these criteria are met [56]. Similarly, restricting potential research subjects to temporary use of a BCI may be a barrier to participation in BCI research. This may obstruct the potential for benefit to the individual and hinder the progress of BCI development toward a clinically available and beneficial product for the wider patient population.
Permanent implantation of an endovascular BCI may offer benefits to the user that extend beyond the time span of a research study. This possibility requires considerations regarding the need for continued technical and medical support [57]. Commitment to life-long follow-up should be necessitated by funders of endovascular BCI research. However, guarantees made by entrepreneurial health technology companies could be unreliable given the high business risks involved in entering this emerging market. If the manufacturer of a BCI goes out of business and the device is no longer available, then any benefit the user received could discontinue. Replacing or maintaining components of the device may not be possible, which could transform a beneficial situation into a potentially harmful one [53].
The permanent implantation of endovascular BCIs could also make recipients ineligible for future opportunities including subsequent generations of devices or different upgrades that may offer greater benefit. This should be made clear within the informed consent process. As much as possible, manufacturers should consider the compatibility of first-generation endovascular BCIs with anticipated future device generations to ensure the maximization of benefit from the implantation of investigational endovascular BCIs.
From a research and development perspective, the long-term safety outcomes of a BCI are not clear from transient implantation of devices. Permanent implantation during typically low n feasibility studies early in the development process would provide the most information on safety prior to larger pivotal trials and post-market surveillance involving larger cohorts. Similarly, some EEG features are more robust over time (“permanent”) than others [58]. Therefore, the efficacy of BCI systems over extended periods may remain unknown without longitudinal monitoring across the lifespan of a device, even if biological interactions with the implanted materials can be demonstrated as stable or mitigated. These benefits must be weighed against the risk of harm to the first recipients of permanent devices.
4.3 Justice
The permanency of endovascular BCIs engenders deliberation on which patient populations might be appropriate for feasibility studies of investigational devices. Some exclusions should certainly be applied. Pediatric populations with still developing vascular anatomy would be inappropriate, at least for the current generation of devices. Other decisions may be less clear. For example, individuals with life-limiting disorders such as ALS may be less impacted by the inability to receive future upgrades (see Beneficence). As such, researchers might seek to target these patient populations. However, access to these opportunities should not be restricted from eligible individuals with other neurological conditions, providing they are fully informed prior to giving their consent. Equally, those with limited expected life spans may be viewed as being more willing to take on higher levels of risk or less impacted by severe adverse events; however, the potential for harm should not be concentrated in this population.
4.4 Autonomy
Individuals with severe communication difficulties are a target population of some BCIs. For these individuals, providing informed consent may be difficult or even impossible, especially if simple yes/no responses are considered insufficient and amounting only to assent at best [59]. In these cases, legally authorized representatives may issue consent on the patient’s behalf [57]. However, the BCI may restore the capacity for higher levels of communication and subsequently the ability to exercise greater autonomy [60] including the ability to consent for themselves. Should the recipient not wish to continue the use of their BCI they may be unable to have the device removed. This would leave only the potential for harm from the unused implanted device. Therefore, the use of endovascular BCIs might be precluded for individuals unable to provide autonomous informed consent. Alternatively, the use of non-invasive BCIs might enable the ability to communicate informed consent for more invasive BCIs including endovascular devices.
While it is not beyond the realms of possibility that temporary endovascular BCIs will be developed, the endothelialization of the materials in the current generation of devices dictates that, for the near future at least, their placement within the brains of humans will be permanent. This will necessitate an adjustment to existing bioethical considerations surrounding the development and use of invasive BCIs. This chapter section outlined some initial considerations; however, with early feasibility testing of investigational endovascular BCIs underway, further attention is urgently required.
5 Informed Consent for Endovascular BCIs
The informed consent process serves to provide the information pertinent to participation in a research trial, as well as comprehension of that information, to enable an informed, voluntary decision on participation to be made by the potential subject or their legally authorized representative. The information provided on the informed consent form, along with the opportunity to ask questions about the research trial, helps subjects to weigh the unique risks and benefits for them as individuals. The uncertain risk profile and permanency of endovascular BCIs creates additional challenges for researchers and participants to convey and comprehend any potential risks and benefits. The goal of this chapter section is to highlight some of these challenges.
Endovascular BCI is still an investigational treatment, and its therapeutic viability has not been established [1]. Caution should be applied when participants are determining the clinical benefit from clinical trials [61, 62]. Unrealistically high expectations pose a risk of psychological harm to subjects [63] and should be screened for before and during the informed consent process. Comprehension of the information provided during consent should help to provide a realistic level of expectation of benefit. For example, researchers consenting for feasibility trials should emphasize that the primary goal of the study is to investigate safety outcomes and that no expectation of benefit can be assured. Researchers should also be careful not to over-compensate for a lack of supporting evidence for BCIs by over-stressing the technological limitations [64, 65]. As mentioned previously, the permanency of endovascular BCIs introduces potential risks including MRI incompatibility, ineligibility for future devices or upgrades, and the possibility for tech support to become unavailable. These risks should also be covered during informed consent to guide realistic expectations of the potential for harm.
Prior literature has identified some of the psychosocial challenges of high importance to BCI users and several target populations. Users regard the ability to feel like the author of one‘s actions have a social life, self-esteem, freedom, and empowerment as critical aspects in the use of BCI [66]. Additionally, the aesthetics and medical connotations of BCI systems have been considered a significant barrier to adoption by many users [32, 67,68,69]. In the context of endovascular BCI, the electrodes of the device might be hidden, but wires or devices connected to the patient still pose a risk for users to feel stigma. These and other considerations reported as important by BCI user groups should be addressed during the informed consent process to adequately convey the potential for both risks and benefits. However, simply disclosing psychosocial risks to potential participants may not be sufficient for an informed decision to be made. Risks such as challenges to personal identity, loss of agency, and stigma may require a deeper exploration [63]. Moreover, the meaning of a shift in identity, loss of agency, or the experience of stigma can only be determined on an individual basis [65, 70]. These experiences related to BCI have been evaluated over the course of an intervention, but their exploration during the informed consent process is rarely reported. Moreover, assessing these risks requires high self-awareness, and many subjects may not be accustomed to health-related self-reflection [71]. Newly disabled patients may not possess this self-awareness due to insufficient experience with their condition [72]. The process of self-reflection prior to consenting to participation in an endovascular BCI trial may be additionally complicated due to both the novelty and permanency of this technology. For procedures as significant as the implantation of a permanent endovascular BCI, the study team might require a trained psychologist to explore a potential subject’s suitability beyond meeting a set of rigid inclusion and exclusion criteria.
In outlining their frameworks for informed consent best practices for implantable BCIs, Klein and Ojemann [72] emphasized the importance of self-reflection and exploration of participant’s preferences and values. They expressed that the subjects are required to understand their values, preferences, and goals to project how their life will change in the years following implantation of a BCI. Qualitative information on current and potential BCI end-users are available and could be used to create a method or tool(s) to help participants explore their needs, values, and goals with respect to BCI [55, 68, 73, 74]. However, much of the current BCI research has not incorporated these existing insights or suggestions [66]. Researchers and clinicians may also need to enhance their understanding of common BCI user perspectives to develop effective informed consent processes [75].
Frameworks for guiding value-based decision-making in healthcare may be applicable to informed consent for undergoing clinical procedures within a BCI research trial. For example, the Patient Priorities Care prototype is a clinically feasible approach to identify and link what matters most to a patient to the health care they are willing and able to participate in [76, 77]. This framework was developed for patients with multiple chronic conditions, which may have similar values to many BCI candidates with chronic conditions [55, 78]. Clinicians on the research team can be trained in identifying patient priorities [79]. Although this training might require additional time and resources, failure to accommodate for a subject’s values ahead of and during the informed consent process could lead to rejection of a potentially efficacious BCI technology.
Due to the permanency of endovascular BCI, greater attention to the needs and values of the potential subjects is warranted during the consent process. If researchers do not attend to end users’ perspectives during the informed consent process, informed consent might be provided for the implantation of a permanent device that may not align with their needs, goals, and values. Effectively evaluating patient suitability on these criteria may require additional resources and expertise, which should be considered during the design stage of research trials and encouraged by funding agencies.
6 Ethical Considerations of Industry Funded Endovascular BCI Research
Traditionally, access to invasive BCI technologies by people living with severe paralysis has occurred under the auspices of government-funded research initiatives, with a primary focus on scientific discovery. However, the field of invasive BCI technology is currently at crossroads with the advent of multiple private and for-profit corporations that have the goal of developing consumer oriented BCI products. This produces a variety of novel and unprecedented ethical challenges that have not been previously navigated in the field of BCI technology. Some of these ethical issues may be common to all invasive BCI technologies, but at the time of writing, there is a particular relevance to endovascular BCIs as the first and, to date, only published human trial of an endovascular BCI [1] investigated the safety and efficacy of the Stentrode™, an endovascular BCI developed by a for-profit corporation: Synchron Inc. (Brooklyn, New York). This section of this chapter will largely focus on how industry funded, invasive BCI research influences both the pace and the publicity of the research and the ethical implications of both.
Industry-sponsored BCI development differs starkly from government sponsored initiatives when it comes to the pace of the research that is to be performed. The field of health technology has frequently cited the concept of the “valley of death,” which is the idea that novel health technologies take, on average, almost two decades to successfully traverse the path from “bench to bedside” [80]. Traditionally, technology developed by industry moves faster than technology that is developed by federal research funding simply due to the differences in timelines [81]. This is due to the disparity in risk tolerance in the two funding sources. By its nature, government-sponsored research has an apparent responsibility to the public to be low-risk and incremental, meaning that the intuitive and innovative leaps forward necessary to keep a health technology startup alive cannot be easily supported within the natural lifespan of a startup company [82]. Thus, industry-sponsored research is required to move at a much faster pace, with a higher risk of failure. However, while the pace of both government- and industry-sponsored research must be tempered by ethics and the well-being of research participants, lowering the overall risk of the implantation procedure for the BCI technology will allow research to move forward at a faster pace. If research involving endovascular BCI technologies do, in fact, pose a lower risk to research participants, they may have greater potential for successful rapid development when compared with intracranial approaches that require penetration of the skull. This difference in research pace, driven by ethics, may be dramatic enough to influence the number of invasive BCI companies that choose an endovascular route in the future. This is a powerful example of how the ethics of BCI technology can influence the focus of research and technological development in the field.
In addition to an accelerated research pace, industry-sponsored research is often much more public-facing, with marketing and media relations professionals who are dedicated to sharing product progress and presenting research findings in the best possible light. Across the field of BCI research, this creates ethical tension, because it can lead to people with disabilities having unrealistic expectations of a BCI product or being led toward a scenario that creates false hope in that product [83]. Notably, at the time of writing this chapter, Elon Musk’s BCI company Neuralink is frequently making headlines in mainstream media about seemingly audacious goals for their novel, implantable BCI device [84]. To be clear, these are not claims that are related to an existing product (because such claims would be subject to the scrutiny of regulatory agencies), these are hopeful statements about the long-term vision of the company. Traditionally trained scientists tend to avoid statements of this style, because their scientific reputation relies upon restricting their public statements to what can be empirically proven by their science. Technology startup CEOs tend to enjoy more latitude when it comes to making such claims, and, as the technology startup ecosystem is increasingly entering the healthcare sector, ethical concerns are certain to emerge. Although it may be tempting to dismiss these concerns as a standard occurrence in consumer product marketing, the ethical implications of these actions could be far-reaching. For instance, a large virtual footprint paired with attractive virtual advertising will significantly increase the number of individuals who express interest in ongoing clinical trials [85, 86]. This strategy is known to be particularly effective for identifying research participants who have rare conditions and may be marginalized or difficult to otherwise reach, which is highly relevant to those with a severe disability [87, 88]. However, research participants who are recruited via social media typically display lower rates of retention than those recruited through more conventional means, especially when participation in clinical trial activities require more than a single interaction with researchers [89,90,91]. This concern is particularly relevant to endovascular BCIs due to the permanency of the technology and the need for long-term follow-up in participants who engage with such a novel, implanted technology. For a technology in its early stages of development with few active users due to regulatory restrictions, the potential loss of data from a premature dropout could be catastrophic to the development of that technology. As such, researchers investigating endovascular technologies must engage in a detailed study recruitment process and mitigation strategies in order to avoid poor retention that can occur as a result of social media recruitment [91]. The disparities that exist between the real-world efficacy and the research needs of endovascular BCI technology and the way that industry sponsored BCI technologies may be advertised must be considered carefully to ensure that false hope in potential research participants does not affect the quality of the research that is conducted.
A final ethical consideration, given the novelty of endovascular BCI technology, relates to the protection of privacy of research participants. Highly publicized research (especially as it pertains to industry-led publicity) that involves very few participants, or participants that are a part of small communities, can run the risk of identifying research participants who may not wish to disclose their involvement in a clinical trial. In addition, researchers who are leading clinical trials involving public–private partnerships, such as government-sponsored research of a privately owned technology, must be particularly vigilant to ensure that recruitment of research participants is not predicated upon the participant’s willingness to be involved in publicity activities, only the research itself.
7 Conclusion
The advent of endovascular electrode arrays in BCI has brought new bioethical considerations and challenges that should be considered as the use of this technology progresses. In particular, the permanency of stent-based electrode arrays has wide-ranging bioethical implications. Endovascular electrode arrays can be placed using minimally invasive surgical procedures, which might attenuate some of the safety concerns in comparison to other types of invasive BCI that require craniotomy. This might contribute to an improved risk-benefit ratio. However, substantially more investigation is required before either the full risks or benefits of endovascular BCIs will become apparent. This considerable uncertainty poses challenges to the informed consent process for clinical trials of BCI technologies. Additionally, subjects entering the consent process may have unrealistic expectations of novel BCIs due to the publicity surrounding some for-profit BCI companies. Overall, this chapter outlined some initial considerations of the bioethics of endovascular approaches to BCI, with further discussion needed.
References
Oxley TJ, Yoo PE, Rind GS, et al. Motor neuroprosthesis implanted with neurointerventional surgery improves capacity for activities of daily living tasks in severe paralysis: first in-human experience. J NeuroInterventional Surg. 2021;13:102–8.
Penn R. Intravascular intracranial EEG recording Technical note. J Neurosurg. 1973;38:239–43.
Sefcik RK, Opie NL, John SE, Kellner CP, Mocco J, Oxley TJ. The evolution of endovascular electroencephalography: historical perspective and future applications. Neurosurg Focus. 2016;40:E7.
Rapeaux AB, Constandinou TG. Implantable brain machine interfaces: first-in-human studies, technology challenges and trends. Curr Opin Biotechnol. 2021;72:102–11.
Raza SA, Opie NL, Morokoff A, Sharma RP, Mitchell PJ, Oxley TJ. Endovascular neuromodulation: safety profile and future directions. Front Neurol. 2020;11:351.
John SE, Grayden DB, Yanagisawa T. The future potential of the Stentrode. Expert Rev Med Devices. 2019;16:841–3.
Rajah G, Saber H, Singh R, Rangel-Castilla L. Endovascular delivery of leads and Stentrodes and their applications to deep brain stimulation and neuromodulation: a review. Neurosurg Focus. 2018;45:E19.
Arya R, Mangano FT, Horn PS, Holland KD, Rose DF, Glauser TA. Adverse events related to extraoperative invasive EEG monitoring with subdural grid electrodes: a systematic review and meta-analysis. Epilepsia. 2013;54:828–39.
Zentner J. Complications. In: Surgical treatment of Epilepsies. Cham: Springer; 2020. p. 331–70.
Bullard AJ, Hutchison BC, Lee J, Chestek CA, Patil PG. Estimating risk for future intracranial, fully implanted, modular neuroprosthetic systems: a systematic review of hardware complications in clinical deep brain stimulation and experimental human intracortical arrays. Neuromodulation. 2020;23:411–26.
Nair DR, Laxer KD, Weber PB, et al. Nine-year prospective efficacy and safety of brain-responsive neurostimulation for focal epilepsy. Neurology. 2020;95:e1244–56.
Nicholson P, Brinjikji W, Radovanovic I, Hilditch CA, Tsang ACO, Krings T, Mendes Pereira V, Lenck S. Venous sinus stenting for idiopathic intracranial hypertension: a systematic review and meta-analysis. J NeuroInterventional Surg. 2019;11:380–5.
Puffer RC, Mustafa W, Lanzino G. Venous sinus stenting for idiopathic intracranial hypertension: a review of the literature: table 1. J NeuroInterventional Surg. 2013;5:483–6.
Starke RM, Wang T, Ding D, Durst CR, Crowley RW, Chalouhi N, Hasan DM, Dumont AS, Jabbour P, Liu KC. Endovascular treatment of venous sinus stenosis in idiopathic intracranial hypertension: complications, neurological outcomes, and radiographic results. Sci World J. 2015;2015:1–8.
Kumpe DA, Bennett JL, Seinfeld J, Pelak VS, Chawla A, Tierney M. Dural sinus stent placement for idiopathic intracranial hypertension: clinical article. J Neurosurg. 2012;116:538–48.
Fan JZ, Lopez-Rivera V, Sheth SA. Over the horizon: the present and future of endovascular neural recording and stimulation. Front Neurosci. 2020;14:432.
Saber H, Lewis W, Sadeghi M, Rajah G, Narayanan S. Stent survival and stent-adjacent stenosis rates following venous sinus stenting for idiopathic intracranial hypertension: a systematic review and meta-analysis. Interv Neurol. 2018;7:490–500.
Ding D, Liu KC. Management strategies for intraprocedural coil migration during endovascular treatment of intracranial aneurysms: table 1. J NeuroInterventional Surg. 2014;6:428–31.
Turek G, Kochanowicz J, Lewszuk A, Lyson T, Zielinska-Turek J, Chwiesko J, Mariak Z. Early surgical removal of migrated coil/stent after failed embolization of intracranial aneurysm. J Neurosurg. 2015;123:841–7.
Teleb MS, Cziep ME, Issa M, Lazzaro M, Asif K, Hun Hong S, Lynch JR, Fitzsimmons B-FM, Remler BF, Zaidat OO. Stenting and angioplasty for idiopathic intracranial hypertension: a case series with clinical, angiographic, ophthalmological, complication, and pressure reporting: an IIH case series with clinical, angiographic, and pressure reporting. J Neuroimaging. 2015;25:72–80.
Boviatsis EJ, Stavrinou LC, Themistocleous M, Kouyialis AT, Sakas DE. Surgical and hardware complications of deep brain stimulation. A seven-year experience and review of the literature. Acta Neurochir. 2010;152:2053–62.
Mokin M, Fargen KM, Primiani CT, et al. Vessel perforation during stent retriever thrombectomy for acute ischemic stroke: technical details and clinical outcomes. J NeuroInterventional Surg. 2017;9:922–8.
Leuthardt EC, Moran DW, Mullen TR. Defining surgical terminology and risk for brain computer Interface technologies. Front Neurosci. 2021;15:599549.
Alawieh A, Fernando Gonzalez L, Feng W. Barriers and opportunities of cortical stimulation via cerebral venous approach. Brain Stimulat. 2020;13:401–2.
Oxley TJ, Opie NL, Rind GS, et al. An ovine model of cerebral catheter venography for implantation of an endovascular neural interface. J Neurosurg. 2018;128:1020–7.
Campbell BCV, Mitchell PJ, Kleinig TJ, et al. Endovascular therapy for ischemic stroke with perfusion-imaging selection. N Engl J Med. 2015;372:1009–18.
Jovin TG, Chamorro A, Cobo E, et al. Thrombectomy within 8 hours after symptom onset in ischemic stroke. N Engl J Med. 2015;372:2296–306.
Saver JL, Goyal M, Bonafe A, et al. Stent-retriever thrombectomy after intravenous t-PA vs. t-PA alone in stroke. N Engl J Med. 2015;372:2285–95.
Peters B, Bieker G, Heckman SM, Huggins JE, Wolf C, Zeitlin D, Fried-Oken M. Brain-computer Interface users speak up: the virtual users’ forum at the 2013 international brain-computer Interface meeting. Arch Phys Med Rehabil. 2015;96:S33–7.
Rashid M, Sulaiman N, Majeed APPA, Musa RM, Ab. Nasir AF, Bari BS, Khatun S. Current status, challenges, and possible solutions of EEG-based brain-computer Interface: a comprehensive review. Front Neurorobot. 2020;14:25.
Holz EM, Botrel L, Kaufmann T, Kübler A. Long-term independent brain-computer Interface home use improves quality of life of a patient in the locked-in state: a case study. Arch Phys Med Rehabil. 2015;96:S16–26.
Carmichael C, Carmichael P. BNCI systems as a potential assistive technology: ethical issues and participatory research in the BrainAble project. Disabil Rehabil Assist Technol. 2014;9:41–7.
Nakase H, Ohnishi H, Touho H, Karasawa J, Yamamoto S, Shimizu K. An intra-arterial electrode for intracranial electro-encephalogram recordings. Acta Neurochir. 1995;136:103–5.
Stoeter P, Dieterle L, Meyer A, Prey N. Intracranial electroencephalographic and evoked-potential recording from intravascular guide wires. AJNR Am J Neuroradiol. 1995;16(6):1214–7.
He BD, Ebrahimi M, Palafox L, Srinivasan L. Signal quality of endovascular electroencephalography. J Neural Eng. 2016;13:016016.
Bower MR, Stead M, Van Gompel JJ, Bower RS, Sulc V, Asirvatham SJ, Worrell GA. Intravenous recording of intracranial, broadband EEG. J Neurosci Methods. 2013;214:21–6.
Oxley TJ, Opie NL, John SE, et al. Minimally invasive endovascular stent-electrode array for high-fidelity, chronic recordings of cortical neural activity. Nat Biotechnol. 2016;34:320–7.
John SE, Opie NL, Wong YT, et al. Signal quality of simultaneously recorded endovascular, subdural and epidural signals are comparable. Sci Rep. 2018;8:8427.
Forsyth IA, Dunston M, Lombardi G, et al. Evaluation of a minimally invasive endovascular neural interface for decoding motor activity. In: 2019 9th International IEEE/EMBS Conference on Neural Engineering (NER). IEEE, San Francisco, CA, pp. 2019. 750–753.
Moran D. Evolution of brain–computer interface: action potentials, local field potentials and electrocorticograms. Curr Opin Neurobiol. 2010;20:741–5.
Lukatch HS, Kiddoo CE, MacIver MB. Anesthetic-induced burst suppression EEG activity requires glutamate-mediated excitatory synaptic transmission. Cereb Cortex. 2005;15:1322–31.
Opie NL, John SE, Rind GS, Ronayne SM, Grayden DB, Burkitt AN, May CN, O’Brien TJ, Oxley TJ. Chronic impedance spectroscopy of an endovascular stent-electrode array. J Neural Eng. 2016;13:046020.
Opie NL, John SE, Rind GS, et al. Focal stimulation of the sheep motor cortex with a chronically implanted minimally invasive electrode array mounted on an endovascular stent. Nat Biomed Eng. 2018;2:907–14.
Polikov VS, Tresco PA, Reichert WM. Response of brain tissue to chronically implanted neural electrodes. J Neurosci Methods. 2005;148:1–18.
Pels EGM, Aarnoutse EJ, Leinders S, Freudenburg ZV, Branco MP, van der Vijgh BH, Snijders TJ, Denison T, Vansteensel MJ, Ramsey NF. Stability of a chronic implanted brain-computer interface in late-stage amyotrophic lateral sclerosis. Clin Neurophysiol. 2019;130:1798–803.
Colachis SC, Dunlap CF, Annetta NV, Tamrakar SM, Bockbrader MA, Friedenberg DA. Long-term intracortical microelectrode array performance in a human: a 5 year retrospective analysis. J Neural Eng. 2021;18:0460d7.
Gerboni G, John SE, Ronayne SM, Rind GS, May CN, Oxley TJ, Grayden DB, Opie NL, Wong YT. Cortical brain stimulation with endovascular electrodes. In: 2018 40th Annual International Conference of the IEEE Engineering in Medicine and Biology Society (EMBC). IEEE, Honolulu, HI, 2018. pp. 3088–3091.
Opie NL, van der Nagel NR, John SE, Vessey K, Rind GS, Ronayne SM, Fletcher EL, May CN, O’Brien TJ, Oxley TJ. Micro-CT and histological evaluation of an neural Interface implanted within a blood vessel. IEEE Trans Biomed Eng. 2017;64:928–34.
Felton EA, Wilson JA, Williams JC, Garell PC. Electrocorticographically controlled brain–computer interfaces using motor and sensory imagery in patients with temporary subdural electrode implants: report of four cases. J Neurosurg. 2007;106:495–500.
Vansteensel MJ, Hermes D, Aarnoutse EJ, Bleichner MG, Schalk G, van Rijen PC, Leijten FSS, Ramsey NF. Brain-computer interfacing based on cognitive control. Ann Neurol. 2010;67:809–16.
Levine SP, Huggins JE, BeMent SL, Kushwaha RK, Schuh LA, Passaro EA, Rohde MM, Ross DA. Identification of electrocorticogram patterns as the basis for a direct brain Interface. J Clin Neurophysiol. 1999;16:439.
Vansteensel MJ, Pels EGM, Bleichner MG, et al. Fully implanted brain–computer interface in a locked-in patient with ALS. N Engl J Med. 2016;375:2060–6.
Glannon W. Ethical issues in neuroprosthetics. J Neural Eng. 2016;13:021002.
Desmoulin-Canselier S. Patient’s lived experience with DBS between medical research and care: some legal implications. Med Health Care Philos. 2019;22:375–86.
Kögel J, Jox RJ, Friedrich O. What is it like to use a BCI?—Insights from an interview study with brain-computer interface users. BMC Med Ethics. 2020;21:2.
Klein E, Peters B, Higger M. Ethical considerations in ending exploratory brain–computer interface research studies in locked-in syndrome. Camb Q Healthc Ethics. 2018;27:660–74.
Hendriks S, Grady C, Ramos KM, et al. Ethical challenges of risk, informed consent, and posttrial responsibilities in human research with neural devices: a review. JAMA Neurol. 2019;76:1506.
Sadeghi K, Lee J, Banerjee A, Sohankar J, Gupta SKS. Permanency analysis on human electroencephalogram signals for pervasive brain-computer Interface systems. In: 39th Annual International Conference of the IEEE Engineering in Medicine and Biology Society (EMBC). IEEE, Seogwipo. 2017. pp. 767–770.
Fins JJ, Schiff ND. In the blink of the mind’s eye. Hast Cent Rep. 2010;40:21–3.
Friedrich O, Racine E, Steinert S, Pömsl J, Jox RJ. An analysis of the impact of brain-computer interfaces on autonomy. Neuroethics. 2021;14:17–29.
Sim J. Distinctive aspects of consent in pilot and feasibility studies. J Eval Clin Pract. 2021;27:657–64.
Fisher CE, Dunn LB, Christopher PP, Holtzheimer PE, Leykin Y, Mayberg HS, Lisanby SH, Appelbaum PS. The ethics of research on deep brain stimulation for depression: decisional capacity and therapeutic misconception: ethics of DBS for depression research. Ann N Y Acad Sci. 2012;1265:69–79.
Klein E. Informed consent in implantable BCI research: identifying risks and exploring meaning. Sci Eng Ethics. 2016;22:1299–317.
Grübler G, Al-Khodairy A, Leeb R, Pisotta I, Riccio A, Rohm M, Hildt E. Psychosocial and ethical aspects in non-invasive EEG-based BCI research—a survey among BCI users and BCI professionals. Neuroethics. 2014;7:29–41.
Specker Sullivan L, Illes J. Ethics in published brain–computer interface research. J Neural Eng. 2018;15:013001.
Kögel J, Schmid JR, Jox RJ, Friedrich O. Using brain-computer interfaces: a scoping review of studies employing social research methods. BMC Med Ethics. 2019;20:18.
Holz EM, Höhne J, Staiger-Sälzer P, Tangermann M, Kübler A. Brain–computer interface controlled gaming: evaluation of usability by severely motor restricted end-users. Artif Intell Med. 2013;59:111–20.
Blain-Moraes S, Schaff R, Gruis KL, Huggins JE, Wren PA. Barriers to and mediators of brain–computer interface user acceptance: focus group findings. Ergonomics. 2012;55:516–25.
Zickler C, Halder S, Kleih SC, Herbert C, Kübler A. Brain painting: usability testing according to the user-centered design in end users with severe motor paralysis. Artif Intell Med. 2013;59:99–110.
Richmond F, Loeb G. Dissemination: getting BCIs to the people who need them. In: Wolpaw JR, editor. Brain–computer interfaces: principles and practice; 2012.
Lim CY, Berry ABL, Hartzler AL, Hirsch T, Carrell DS, Bermet ZA, Ralston JD. Facilitating self-reflection about values and self-care among individuals with chronic conditions. In: Proceedings of the 2019 CHI Conference on Human Factors in Computing Systems. ACM, Glasgow Scotland UK, 2019. pp. 1–12.
Klein E, Ojemann J. Informed consent in implantable BCI research: identification of research risks and recommendations for development of best practices. J Neural Eng. 2016;13:043001.
Huggins JE, Wren PA, Gruis KL. What would brain-computer interface users want? Opinions and priorities of potential users with amyotrophic lateral sclerosis. Amyotroph Lateral Scler. 2011;12:318–24.
Liberati G, Pizzimenti A, Simione L, Riccio A, Schettini F, Inghilleri M, Mattia D, Cincotti F. Developing brain-computer interfaces from a user-centered perspective: assessing the needs of persons with amyotrophic lateral sclerosis, caregivers, and professionals. Appl Ergon. 2015;50:139–46.
Gilbert F, Cook M, O’Brien T, Illes J. Embodiment and estrangement: results from a first-in-human “intelligent BCI” trial. Sci Eng Ethics. 2019;25:83–96.
Naik AD, Dindo LN, Liew JR, et al. Development of a clinically feasible process for identifying individual health priorities. J Am Geriatr Soc. 2018;66:1872–9.
Tinetti ME, Esterson J, Ferris R, Posner P, Blaum CS. Patient priority–directed decision making and care for older adults with multiple chronic conditions. Clin Geriatr Med. 2016;32:261–75.
Uhlig K, Leff B, Kent D, et al. A framework for crafting clinical practice guidelines that are relevant to the care and Management of People with multimorbidity. J Gen Intern Med. 2014;29:670–9.
Freytag J, Street RL, Barnes DE, Shi Y, Volow AM, Shim JK, Alexander SC, Sudore RL. Empowering older adults to discuss advance care planning during clinical visits: the PREPARE randomized trial. J Am Geriatr Soc. 2020;68:1210–7.
Putrino D. Introduction—why me? In: Putrino D, editor. Hacking health make money save lives heal. World. Cham: Springer; 2018. p. 5–11.
Simon GE, Richesson RL, Hernandez AF. Disseminating trial results: we can have both faster and better. Healthcare. 2020;8:100474.
Putrino D. Identifying opportunities to innovate and create your niche. In: Evidence-based leadership, innovation, and entrepreneurship in nursing and healthcare: a practical guide for success. 1st ed. New York: Springer; 2019. p. 297–312.
Nijboer F, Clausen J, Allison BZ, Haselager P. The Asilomar survey: stakeholders’ opinions on ethical issues related to brain-computer interfacing. Neuroethics. 2013;6:541–78.
Rogers A. Neuralink is impressive tech, Wrapped in Musk Hype. Wired.
Bisset CN, Carter B, Law J, et al. The influence of social media on recruitment to surgical trials. BMC Med Res Methodol. 2020;20:201.
Christensen T, Riis AH, Hatch EE, Wise LA, Nielsen MG, Rothman KJ, Sørensen HT, Mikkelsen EM. Costs and efficiency of online and offline recruitment methods: a web-based cohort study. J Med Internet Res. 2017;19:e6716.
Russomanno J, Patterson JG, Tree JMJ. Social media recruitment of marginalized, hard-to-reach populations: development of recruitment and monitoring guidelines. JMIR Public Health Surveill. 2019;5:e14886.
Topolovec-Vranic J, Natarajan K. The use of social Media in Recruitment for medical research studies: a scoping review. J Med Internet Res. 2016;18:e5698.
Leonard A, Hutchesson M, Patterson A, Chalmers K, Collins C. Recruitment and retention of young women into nutrition research studies: practical considerations. Trials. 2014;15:23.
Murray E, Khadjesari Z, White I, Kalaitzaki E, Godfrey C, McCambridge J, Thompson S, Wallace P. Methodological challenges in online trials. J Med Internet Res. 2009;11:e1052.
Pedersen ER, Kurz J. Using Facebook for health-related research study recruitment and program delivery. Curr Opin Psychol. 2016;9:38–43.
Author information
Authors and Affiliations
Corresponding author
Editor information
Editors and Affiliations
Rights and permissions
Copyright information
© 2023 The Author(s), under exclusive license to Springer Nature Switzerland AG
About this chapter
Cite this chapter
Fry, A., Breyman, E., LaGrassa, E., Oxley, T., Putrino, D. (2023). Ethical Considerations of Endovascular Brain–Computer Interfaces. In: Dubljević, V., Coin, A. (eds) Policy, Identity, and Neurotechnology. Advances in Neuroethics. Springer, Cham. https://doi.org/10.1007/978-3-031-26801-4_4
Download citation
DOI: https://doi.org/10.1007/978-3-031-26801-4_4
Published:
Publisher Name: Springer, Cham
Print ISBN: 978-3-031-26800-7
Online ISBN: 978-3-031-26801-4
eBook Packages: MedicineMedicine (R0)