Abstract
This study investigated the effect of using waste glass powder (GP) as partial replacement of fly ash on the strength and microstructure development of geopolmer binders. GP was used at different rates varying from 1 to 5% mass of fly ash and 8 molar NaOH solution was used as the activator. Geopolymer paste specimens of 50 mm cube were heat cured at 80 °C for the first 24 h after casting and then left in ambient conditions. The 28-day compressive strength of the fly ash geopolymer without GP was 23 MPa. The inclusion of 2% GP improved the 28-day compressive strength of heat cured fly ash geopolymer by 18%. This is attributed to the increment of soluble silica from the GP. However, compressive strength declined with further increase of GP content beyond 2%. The decline of strength is attributed to the presence of excess unreacted GP particles. X-ray diffraction (XRD) analysis indicated that the presence of higher amount of crystalline Na-type chabazite and zeolite phases for using 2% GP. Scanning electron microscopy (SEM) images showed denser microstructure of geopolymers containing 1–2% GP. The elemental composition of gel formed in these binders consisted of higher percentages of Si, Al and Na, which indicates the formation of higher amount of sodium aluminosilicate gel (N–A–S–H).
Access provided by Autonomous University of Puebla. Download conference paper PDF
Similar content being viewed by others
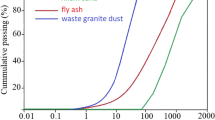
Keywords
1 Introduction
Research on alkali activated binders or geopolymer binders is increasing in the recent time since it has a huge potential to reduce the carbon footprint of construction sector by replacing Portland cement. Alkali activated binders or geopolymer binders are produced by the chemical reaction between aluminosilicate materials and an alkali activator. In this binder system, two most common industrial by-products such as fly ash and ground granulated blast furnace slag (GGBFS) can be used as the main aluminosilicate sources. Besides, sodium hydroxide, sodium carbonate and sodium silicate solutions are the most effective and common alkaline activators used for activation of the solid aluminosilicate source materials [1, 2]. Previous studies showed that the amount of available reactive silica in the source materials has a great impact on the geopolymerization process [3, 4]. Therefore, some studies added a certain amount of silica rich materials such as nanosilica and silica fume to improve the geopolymerization process [5, 6]. For instance, Deb et al. [5] found that the use of 2% nanosilica significantly improved the workability, compressive strength, sorptivity and acid resistance of fly ash based geopolymer mortars. Similarly, Ramezanianpour and Moeini [6] noticed that the inclusion of 5% silica fume enhanced the mechanical and durability properties of alkali activated slag mortars. Besides, some researchers studied the effects of rice husk ask, sugar cane bagasse ash and palm oil fuel ash in geopolymers due to the availability of reactive silica in these materials [7,8,9].
The current research trend indicates that waste glass powder (GP) has a potential to be used as a supplementary binder material in cementitious systems due to the abundance of reactive silica [10, 11]. Usually, GP is produced by crushing the waste glasses. However, studies related to the use of GP as a source of reactive silica in geopolymers is very limited. Only a few studies were conducted to determine the feasibility of GP in geopolymers as a source of reactive silica [12,13,14]. Therefore, the primary objective of this study is to determine the effect of glass powder on the strength and microstructural development of fly ash geopolymer binders. In this regard, compressive strength and microstructural development of fly ash geopolymer paste samples with 0, 1, 2, 3, 4 and 5% GP were studied.
2 Materials and Methods
2.1 Materials
In this study, fly ash was used as the main aluminosilicate source and waste glass powder (GP) was used as a partial replacement of fly ash. Fly ash was collected from the Gladstone power station in Queensland, Australia and glass powder was produced by crushing waste glass cullet using a laboratory ball mill. As seen in Table 1, the fly ash used in this study is classified as class F according to ASTM C618 [15] since the total of SiO2, Al2O3 and Fe2O3 contents exceeded 70% and the amount of SO3 was only 0.25%. GP was mainly consisted of 70.61% SiO2, 10.90% CaO and 12.8% Na2O. The particle diameters of fly ash and GP are presented in Table 2. It can be seen that the average particle sizes (d50) of fly ash and GP were 13.62 µm and 10.74 µm, respectively which indicates that waste glass powder used in this study was finer than the fly ash. The photographs of fly ash and GP are shown in Fig. 1.
2.2 Preparation of the Testing Samples
The binder compositions of the geopolymer paste mixes are provided in Table 3. The mix proportions were selected based on the previous work reported by Deb et al. [5]. Fly ash was used as the main binder with 0–5% GP as fly ash replacement and 8 M NaOH solution was used as the alkaline solution. The number at the end of a mix ID in Table 3 represents the mass percentage of GP in the solid binder. The amount of alkaline solution was kept constant at 40% of the total binder. First, all the dry ingredients were placed in the Hobart mixer and then 8 M NaOH solution was added as the mixing continued to prepare the geopolymer paste mixture. Later, this freshly prepared geopolymer paste were poured into the 50 mm acrylic plastic cube moulds. The moulds were placed in an oven after casting and cured it for the next 24 h at 80 °C. After the desired heat curing period, the specimens were demolded and stored in room temperature of 20 ± 2 °C with a relative humidity of 70 ± 10% until testing age.
The compressive strength of paste specimens was determined at 28 days using a 300 kN Shimadzu machine with a constant loading rate of 0.9 kN/s as recommended in ASTM C109 [16]. Scanning electron micrographs (SEM) and energy dispersive X-ray (EDX) spectroscopy were conducted using a NEON 40EsB (ZEISS) microscopy instrument on small cut paste sample to examine the morphology and microstructure of the paste specimens. The samples were coated with carbon before SEM and EDX analysis. X-ray diffraction (XRD) was performed on powdered paste samples at 28 days using a D8 advance (Bruker AXS) instrument to investigate the reaction products of the paste samples.
3 Results and Discussion
3.1 Compressive Strength
The effect of GP content on the compressive strength of fly ash geopolymers is shown in Fig. 2. It has been noticed that compressive strength increased for 1–2% GP in fly ash geopolymers and the maximum compressive strength was achieved for 2% GP. For instance, FGP1, FGP2 and FGP3 geopolymer paste samples showed about 13, 18 and 5% higher compressive strength as compared to the FGP0 geopolymer paste sample. Besides, it is noted that compressive strength declined when the amount of GP content exceeded to 2%. This can be attributed to the decrease of Si/Na ratio and increase of Si/Al ratio by the inclusion of GP, as shown in Table 2. It can be seen that the amounts of SiO2, and Na2O were higher in GP than in fly ash, whereas the amount of Al2O3 was significantly higher in fly ash than in GP. As a result, when a small percentages of GP (about 1–3%) was used to replace fly ash, it improved the geopolymerization process by adding additional soluble Si, Al and Na. However, the use of GP beyond 3% could not produce additional geopolymeric product. This is attributed to the reduced amount of reactive Al. Therefore, compressive strength declined with the increase of GP content above 2%. These results show good agreement with the results reported by Torres-Carrasco and Puertas [12] and Deb et al. [5].
3.2 Microstructural Investigation
3.2.1 XRD Analysis
The XRD results of fly ash geopolymers containing 0, 2 and 5% glass powder are shown in Fig. 3. In all cases, quartz, mullite, chabazite and zeolite are the main detected crystalline phases. The peaks of crystalline quartz and mullite phases are considered to be due to the presence of unreacted fly ash particles in these mixes, whereas crystalline chabazite and zeolite phases represent the formation of sodium aluminosilicate hydrate (N–A–S–H) gel. It is seen that the presence of chabazite and zeolite phases in FGP2 paste are more prominent than the FGP0 and FGP5 paste samples which indicate the formation of a greater amount of N–A–S–H gel in the FGP2 paste mix. The formation of this greater amount of N–A–S–H gel in FGP2 paste mix is mainly attributed to the dissolution of additional Si and Na that was present in GP. Therefore, FGP2 showed the maximum compressive strength. Besides, the obtained peaks for quartz and mullite in FGP5 paste is higher than the FGP0 paste which indicates the presence of more unreacted particles in FGP5 paste. As a result, FGP5 showed lower compressive strength as compared to FGP0 paste specimen.
3.2.2 SEM and EDX
The SEM and EDX of FGP0, FGP2 and FGP5 paste specimens are presented in Figs. 4, 5 and 6, respectively. By comparing Figs. 4 and 5, it is seen that the elemental compositions of the reaction product observed in FGP0 and FGP2 pastes are quite similar. This reaction product is mainly sodium aluminosilicate gel which is consisted of primarily Si, Al and Na as confirmed by EDX. However, the presence of unreacted fly ash particles and voids are noticeably higher in FGP0 specimen than the FGP2 specimen. In addition, it is also noticed that the unreacted fly ash particles in FGP2 paste are well connected with the reaction products, whereas a loose connection is observed between the unreacted fly ash particles and reaction product in FGP0 paste. Furthermore, it is noticed that the microstructure of FGP5 contained greater amount of unreacted fly ash particles with visible cracks and internal voids. Besides, the peaks for Si, Al and Na in the reaction products are comparatively lower in FGP5 paste than in FGP0 and FGP2 pastes. This shows a good agreement with the XRD results reported in the earlier section as well as with some previous studies [5, 12].
4 Conclusions
Strength and microstructure development of fly ash geopolymers with 0–5% GP were studied. The following conclusions can be drawn based on the results of the experimental study:
-
1.
Compressive strengths of the fly ash geopolymers increased with the use of GP up to 2% level and strength declined with the further increase of GP. The geopolymer paste containing 2% GP showed about 18% higher compressive strength than the geopolymer paste without GP.
-
2.
XRD analysis showed that fly ash geopolymer with 2% GP had higher peaks for crystalline chabazite and zeolite phases, which indicates the formation of higher amount of sodium aluminosilicate hydrate (N–A–S–H) gel. This is attributed to the dissolution of additional Si and Na from 2% GP.
-
3.
The SEM images and associated EDX analysis indicated that the microstructure of fly ash geopolymer containing 2% GP had fewer unreacted fly ash particles and less voids as compared to the microstructure of fly ash geopolymer without GP.
-
4.
Therefore, it is concluded that the use of 2% GP in fly ash geopolymers improved compressive strength and microstructure development due to the improvement of reaction kinetics by providing additional soluble Si and Na.
References
Provis, J.L.: Geopolymers and other alkali activated materials: why, how, and what? Mater. Struct. 47(1–2), 11–25 (2014)
Karim, M.R., Hossain, M.M., Khan, M.N.N., Zain, M.F.M., Jamil, M., Lai, F.C.: On the utilization of pozzolanic wastes as an alternative resource of cement. Materials 7(12), 7809–7827 (2014)
Chen-Tan, N.C., Riessen, Ly, C.V., Southam, D.C.: Determining the reactivity of a fly ash for production of geopolymer. J. Am. Ceramic Soc. 92(4), 881–887 (2009)
Criado, M., Fernandez, J.A., Torre, A.G., Aranda, M.A.G., Palomo, A.: An XRD study of the effect of the SiO2/Na2O ratio on the alkali activation of fly ash. Cem. Concr. Res. 37(5), 671–679 (2007)
Deb, P.S., Sarker, P.K., Barbhuiya, S.: Effects of nano-silica on the strength development of geopolymer cured at room temperature. Constr. Build. Mater. 101, 675–683 (2015)
Ramezanianpour, A.A., Moeini, M.A.: Mechanical and durability properties of alkali act vated slag coating mortars containing nanosilica and silica fume. Constr. Build. Mater. 163, 611–621 (2018)
Meija, J.M., Mejia de Gutierrez, R., Puertas, F.: Rice husk ash as a source of silica in alkali-activated fly ash and granulated blast furnace slag systems. Mater. de Construccion 63(311), 361–375 (2013)
Moraes, J.C.B., Tashima, M.M., Akasaki, J.L., Melges, J.L.P., Monzo, J., Borrachero, M.V., Soriano, L., Paya, J.: Effect of sugar cane straw ash (SCSA) as solid precursor and the alkaline activator composition on alkali-activated binders based on blast furnace slag (BFS). Constr. Build. Mater. 144, 214–224 (2017)
Toniolo, N., Boccaccini, A.R.: Fly ash-based geopolymers containing added silicate waste. A review. Ceramics Int. 43(17), 14545–14551 (2017)
Khan, M.N.N., Saha, A.K., Sarker, P.K.: Reuse of waste glass as a supplementary binder and aggregate for sustainable cement-based construction materials: a review. J. Build. Eng. 28, 101052 (2020)
Khan, M.N.N., Sarker, P.K.: Alkali silica reaction of waste glass aggregate in alkali activated fly ash and GGBFS mortars. Mater. Struct. 52(93), 1–17 (2019)
Torres-Carrasco, M., Puertas, F.: Waste glass in the geopolymer preparation. Mechanical and microstructural characterization. J. Clean. Prod. 90, 397–408 (2015)
El-Naggar, M.R., El-Dessouky, M.I.: Re-use of waste glass in improving properties of metakaolin-based geopolymers: mechanical and microstructure examinations. Constr. Build. Mater. 132, 543–555 (2017)
Liu, Y., Shi, C., Zhang, Z., Li, N.: An overview on the reuse of waste glasses in alkali activated materials. Resour. Conserv. Recycl. 144, 297–309 (2019)
ASTM C618: Standard Specification for Coal Fly Ash and Raw or Calcined Natural Pozzolan for Use in Concrete. ASTM International, West Conshohocken, PA (2012)
ASTM C109/C109M: Standard Test Method for Compressive Strength of Hydraulic Cement Mortars (Using 2-in. or [50-mm] Cube Specimens), ASTM International, West Conshohocken, PA (2013)
Acknowledgements
The first author received Curtin International Postgraduate Research Scholarship (CIPRS) and Curtin Strategic International Research Scholarship (CSIRS) from Curtin University, Australia. The authors would like to thank SKM Recycling, Victoria, Australia for providing the waste glass cullet for this study. Besides, the assistance of John de Laeter Centre at Curtin University to the microstructural analysis is gratefully acknowledged.
Author information
Authors and Affiliations
Corresponding author
Editor information
Editors and Affiliations
Rights and permissions
Copyright information
© 2021 The Author(s), under exclusive license to Springer Nature Switzerland AG
About this paper
Cite this paper
Khan, M.N.N., Kuri, J.C., Sarker, P.K. (2021). Strength and Microstructure Development of Fly Ash Geopolymer Binders Using Waste Glass Powder. In: M.C.F. Cunha, V., Rezazadeh, M., Gowda, C. (eds) Proceedings of the 3rd RILEM Spring Convention and Conference (RSCC 2020). RSCC 2020. RILEM Bookseries, vol 35. Springer, Cham. https://doi.org/10.1007/978-3-030-76543-9_5
Download citation
DOI: https://doi.org/10.1007/978-3-030-76543-9_5
Published:
Publisher Name: Springer, Cham
Print ISBN: 978-3-030-76542-2
Online ISBN: 978-3-030-76543-9
eBook Packages: EngineeringEngineering (R0)