Abstract
This chapter addresses the dilemma facing the clinician when serum thyroglobulin (Tg) is clearly measurable post-thyroidectomy and radioiodine ablation, thereby indicating evidence of residual thyroid cancer, but traditional isotopic scans with iodine-123 or iodine-131 are negative and hence the source of the thyroglobulin cannot be localizable. Causes for a Tg-positive/scan-negative situation are described as is the approach to management of such patients by methods of alternative imaging modalities or by empiric radioiodine treatment. The opposite clinical picture of the patient with an undetectable serum Tg but a positive isotope scan is also discussed briefly.
Access provided by Autonomous University of Puebla. Download chapter PDF
Similar content being viewed by others
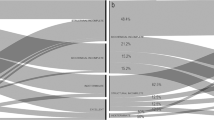
Keywords
- Thyroglobulin
- Anti-thyroglobulin antibodies
- Radioisotope scan
- Empiric radioiodine treatment
- FDG-PET scan
In the past two decades, significant improvements in the assays for serum thyroglobulin (Tg) have revolutionized the standard follow-up and surveillance for recurrence in patients with thyroid carcinoma [1–6]. Not infrequently, we are faced with the management dilemma presented by patients with differentiated thyroid cancer (DTC) in whom measurable or high serum Tg levels suggest residual or metastatic disease, but their radioiodine diagnostic survey scans are negative [7].
Some workers have advocated empiric high-dose radioiodine therapy in these patients, based on the Tg levels indicating disease, even when the negative scan suggests there will be little to no uptake. However, this approach has been somewhat controversial [8–14]. The National Comprehensive Cancer Network guidelines [15] are far from definitive on this issue but point out that no studies have yet demonstrated significantly reduced morbidity and mortality from radioiodine therapy given strictly for elevated serum Tg levels. The British Thyroid Association guidelines [16] are completely silent on the dilemma. Pacini and Schlumberger [17] were the first investigators to advocate this empiric high-dose 131I therapy for patients who are “scan-negative, Tg-positive,” whereas Sherman and Gopal [18] advised caution in the absence of data confirming efficacy and an acceptable risk/benefit ratio. It is not likely that Pacini and Schlumberger would have the same opinion today, as members of the American Thyroid Association Cancer Guidelines Committee that recommends that radioiodine not be given for radioiodine refractory tumors, i.e., in the absence of radioiodine uptake [19].
When initially faced with this perplexing pairing of diagnostic data—positive Tg and negative scan—it is essential to first attempt to uncover a cause for a possibly false-negative scan or a false-positive elevation of serum Tg before even considering empiric radioiodine therapy. For example, a falsely positive serum Tg level could occur because of interfering anti-Tg antibodies [20–23]. Explanations for a false-negative radioiodine scan include inadequate thyrotropin (TSH) elevation, stable iodine contamination (e.g., history of recent iodine contrast radiography), dispersed microscopic metastases too small to visualize, or dedifferentiation of the tumor such that it can still produce Tg but has lost its iodide-trapping ability. It has even been speculated that there could be radioiodine-resistant remnants of normal thyroid tissue or Tg secretion from thymus gland that might account for the measurable Tg [24]. On the other hand, the failure to visualize foci of uptake on a radionuclide scan could be due to iodine excess. To rule out iodine contamination, serum or urinary iodide can be measured, and if found to be moderately elevated, a repeat total-body scan (TBS) 4–6 weeks after an iodide depletion regimen can be considered [25]. It is also possible, as has been seen in some series of patients, that patients with residual tumor may have both undetectable serum Tg and a negative scan, presumably representing dedifferentiation of these tumors [26].
In managing the patient who presents with a negative scan and measurable Tg, patient-specific clinical aspects should be taken into account before definitive action is taken to prescribe empiric radioiodine therapy. Important matters to consider include risk factors, evidence of prior metastatic or aggressive disease, and the options for employing other imaging tools, such as magnetic resonance imaging (MRI) or ultrasound to visualize potential occult disease. How the clinical context can alter the approach is illustrated by two hypothetical cases. First, in a 60-year-old man with a history of a stage III 4-cm papillary or a 2-cm invasive follicular carcinoma, the author would consider application of the earlier Pacini-Schlumberger approach of empiric treatment with high-dose radioiodine. Alternatively, in a 25-year-old woman with a history of a 2-cm stage I papillary cancer with negative nodes and only marginally measurable or slightly elevated Tg (e.g., <4 ng/mL), the author might favor a more conservative approach, at least for a period of time with continued monitoring of the patient. One argument for simply continuing to monitor is that serum Tg levels are known to fall spontaneously with time over the course of 1–5 years post-ablation [27, 28, 28a].
Whether serum Tg levels are stable or rising is very useful in the decision-making process regarding radioiodine therapy. Of course, the patient must be brought into the decision-making process and informed fully of the extent of collective knowledge, experience, and biases pertaining to that patient’s specific situation. We would like to avoid treatment with aggressive high-dose radioiodine for uncertain indications and treatment that might result in harmful sequelae, such as neutropenia, xerostomia, and/or azoospermia.
As pointed out by Sherman and Gopal [18], the risks of aggressive radioiodine therapy may not be warranted given the ill-defined goals, unless there is evidence of progressive disease. Pacini et al. [17] and Schlumberger et al. [29] are not the only investigators to describe a salutary result from empiric treatment of the Tg-positive/scan-negative patient. Pineda et al. [30] reported their results in 17 Tg-positive/scan-negative patients who all had prior total thyroidectomy and radioiodine ablation. After empiric treatment with 150–300 mCi of 131I, 16 of 17 had visualization of metastases on their posttreatment scan. Tg levels decreased in 81 % of patients after their first treatment dose and decreased in 90 % and 100 % of those who received second and third doses, respectively. Although these results sound impressive [30, 31], examination of the individual patient’s Tg level response is less so. Mean Tg decreased from 74–62 to 32 over 1–2 years of follow-up, and only 6 of 29 positive scans became negative.
A definite tilt toward empiric radioiodine therapy in 16 scan-negative/Tg-positive patients was evident in the report by de Keizer et al. [32] who described a decreased Tg level in 88 % of patients. The period of follow-up was too short to indicate any improvement in survival or disease-free interval; however, the authors proposed treatment of such patients with at least one dose when scans were negative. As with the Pineda and Robbins studies [30, 31], serum Tg did decline in the majority of patients but not dramatically so.
The cogent issues raised by Sherman and Gopal, and previously by McDougall [33] and Mazzaferri [34], reflect the fact that many patients treated by Schlumberger et al. and Pineda and Robbins had minimal, if any, disease that would affect their life expectancy. The empiric therapy would expose them to unwarranted doses of radiation exposure, unwarranted at least until sufficient data is obtained from well-controlled studies that confirm efficacy of therapy. Based on their own experience in 24 patients [35] and their analysis of the literature [36], Fatourechi and Hay suggested two general patient categories. The first group has a higher risk of demonstrating uptake on the scan after high-dose therapy, representing younger patients with diffuse micrometastases and negative whole-body scan and conventional imaging but moderately elevated Tg levels. Patients in the second group are likely to be older, higher risk patients with known metastases that release Tg and do not take up radioiodine but are identified by other imaging. In their experience, this latter group will not demonstrate uptake on a posttreatment scan and therefore does not warrant therapy.
Arguably, the most useful experience reported to date is that of Pacini et al. [28] who compared the outcomes in 42 scan-negative/Tg-positive patients (group 1) treated with radioiodine to 28 patients (group 2) followed without treatment, where the average follow-up was 6.7 and 11.9 years, respectively. The first posttherapy scan was positive in 30 of 42 treated patients, negative in 12, and only the patients with positive scans were given additional 131I therapy. Complete remission was seen in 10 of 30 (normalized Tg levels), partial remission (still detectable Tg) in 9 of 30, and evidence of persistent disease in 11 of 30 (measurable Tg and scans became positive). Among these 30 patients with positive posttherapy scans, when radioiodine uptake was seen in the lungs (metastatic disease) on the posttherapy scan, it resolved in 8 of 9 (89 %) cases but in only 11 of 18 cases with cervical lymph node involvement. In the remaining 12 of the 42 group 1 patients who did not have positive scans after treatment doses, 2 entered remission, 7 had persistent Tg elevations, 2 had mediastinal lymph node involvement, and only 1 died of disease during the follow-up period. The changes in Tg were not directly compared to the changes in the no-treatment group. Significantly, of these 28 patients (group 2) who were followed with no treatment, 19 of 28 (68 %) became Tg-negative, another 6 of 28 (21.4 %) were unchanged, and only 3 (11 %) had an increase in Tg levels. Pacini et al. concluded that there may be a role for empiric 131I therapy in patients with pulmonary metastases but that their data supporting empiric therapy in patients with cervical lymph nodes was far from compelling. In view of the remarkable stability in those who were not treated, they did not advocate further radioiodine therapy in those patients whose first posttherapy scan remains negative—an approach that seems to be quite balanced.
Serum Tg levels drawn soon after ablation have prognostic value in regard to future recurrence [37]. It was possible to compare the change in Tg levels in 28 treated vs 32 nonradioiodine-treated patients in the study by Koh et al. [38]. Significantly greater reductions in Tg were seen with 131-I therapy, with four patients actually becoming undetectable. Posttherapy scans became positive in 12 of 28 (43 %) of the scan-negative/Tg-positive patients. Like deKeizer et al. [32], the authors encouraged consideration of empiric radioiodine therapy, both for palliation and potential localization of lesions on the posttreatment scan.
In their review of the literature, Ma et al. [39] concluded that the decision to treat should be individualized on the basis of the extent of disease as indicated by serum Tg levels. Thus, individuals with Tg levels >10 ng/mL or patients stratified at high risk for recurrence should be treated with radioiodine with the expectation that approximately two thirds will show both a fall in Tg and a positive posttreatment scan.
It may seem easy to recommend empiric therapy based on these data indicating declines in serum Tg after treatment, but it should be noted that improvement in survival is yet to be shown. In addition to the potential untoward complications of high-dose radioiodine therapy mentioned above (and enumerated in Chap. 50), another important aspect of empiric therapy is the cost to the patient regarding either the morbidity of hypothyroidism or the dollar cost of rhTSH and the negative impact on productivity when hypothyroid, as well as the cost in health care related to hospitalization and the associated expensive technological procedures.
In the author’s approach to the scan-negative/Tg-positive patient, it is important to turn at an early stage in evaluation to alternative imaging procedures. Although radioiodine therapy may not be feasible because of the lack of visible uptake on diagnostic scanning, alternative therapeutic approaches to metastatic deposits of thyroid cancer may be available. These might include surgical excision, localized ablation by ethanol instillation or radiofrequency ablation (see Chap. 54), or localized external-radiation therapy ([40, 41]; see Chap. 51), but the location of the metastases should first be identified and assessed before one of these approaches is taken. Imaging with computed tomography (CT), MRI, and ultrasound have been employed for this purpose, and alternative scanning agents may have a role in identifying lesions that are not visualized with traditional 131I whole-body scan (see Chap. 35).
Alternative Scanning Agents
Two early scanning agents used as alternatives to radioiodine were 201Tl [42] and 99mTc sestamibi [43]. In one study of patients with bone metastases documented with positive 131I scans, 201Tl was compared to the bone agent, 99mTc hydroxymethylene diphosphonate (99mTc-HMDP; [42]). The two agents had a combined sensitivity of 93.5 %. In a group of 14 patients with negative 131I scans and other evidence of thyroid malignancy, 201Tl was positive in 10 of 14, and 99mTcHMDP was positive in all 14. Carril et al. [44] found that 201Tl showed a sensitivity and specificity higher than that associated with 131I for recurrent or persistent disease. Lesions were detected in 31 of 116 patients by 201Tl but not by 131I TBS. In patients who have been ablated and show no further 131I uptake, the authors proposed continuing management with no additional 131I scans. Because 201Tl scanning does not require levothyroxine withdrawal, follow-up would be guided only by 201Tl scanning and monitoring serum Tg. Dadparvar et al. [45] compared 201Tl and scanning with 99mTc-methoxyisobutyl isonitrile (99mTc-MIBI) . They found that a 131I TBS alone was satisfactory as a preablation diagnostic study, but the addition of either alternative agent increased the diagnostic yield postablation, particularly when the 131I TBS was negative. However, these results have not been universal because Lorberboym et al. [46] found 131I TBS to be more sensitive and specific than 201Tl, with the latter giving several false-positive scans. Ugur et al. [47] noted a 70 % overall concordance between 201Tl, 99mTcMIBI, and 131I TBS but observed false-negatives with both alternative agents, concluding that they should not be used in lieu of 131I TBS. In one reported case, 201Tl scanning was positive and useful in a patient with metastatic disease with both negative 131I TBS and negative serum Tg levels [48].
Employing 99m-Tc-depreotide , Rodriques et al. [49] were able to visualize lesions in 9/10 radioiodine scan-negative patients with suspected residual disease. In studies with the agent, 99mTc sestamibi, Elser et al. [50] noted a 94 % sensitivity for the detection of positive lymph nodes and local recurrence; they detected 32 of 40 metastases with sestamibi vs only 18 of 40 with an 131I TBS. Another cationic scanning agent, 99mTc tetrafosmin, which has been used previously for myocardial perfusion imaging, was assessed for the detection of thyroid cancer [51–53]. For 12 patients with elevated serum Tg (4 with negative 131I TBS), tetrafosmin was slightly superior to 201Tl and 99mTc-MIBI. This same group of workers [53] reported that tetrafosmin successfully identified all of 21 lesions that were positive by 131I TBS, as well as an additional 17 of 23 lesions that were negative by 131I TBS. The agent had 86 % sensitivity for distant metastases and was positive in four patients with 131I-negative proven pulmonary metastases. The findings correlated with other imaging modalities for tumor identification, e.g., CT or ultrasound.
It is also significant that these alternative agents are logistically both more convenient and expedient than scanning with 131I. Along with the ability to scan patients while euthyroid and still on TSH-suppressive levothyroxine therapy, the time required for evaluation is much reduced. Instead of scanning 48–72 h after a dose of 131I, the 99mTc tetrafosmin planar scan is performed 20 min after injection of the isotope, with additional images taken by single-photon emission computed tomography of any suspicious lesions. 99mTc tetrafosmin scans were negative in all 68 patients studied by Lind et al. [53], who were free of disease on the basis of 131I TBS and serum Tg.
Fluorodeoxyglucose-Positron Emission Tomography (Chaps. 34 and 61)
Some insight into the minimal value of the above-described imaging agents is apparent from the fact that they have not been widely adopted for this group of patients over the past several years. Rather, another agent, 18-fluorine fluorodeoxyglucose (FDG), has shown the most promise and has been employed with positron emission tomography (PET) for detection of thyroid cancer with uptake of the agent related to glucose utilization by tumor tissue [54–57]. Indeed, FDG-PET scanning is rapidly becoming a part of the routine armamentarium of diagnostic imaging procedures for thyroid cancer, especially metastatic disease [58, 59], and may be the optimal imaging modality for medullary thyroid cancer [60]. Notwithstanding that several workers have recommended its routine use [61], its more widespread adoption has been limited by its relatively high cost and the reluctance of insurers to provide coverage for all but selected patient populations. One of the populations in which PET scanning has been found useful is the scan-negative/Tg-positive patients. Numerous publications testify to its utility in both individual patient case reports and small patient series [62–68].
The greatest uptake sensitivity for FDG-PET scanning has been noted with the fastest growing undifferentiated tumors. Grunwald et al. [69] compared FDG-PET to 99mTc sestamibi and 131I TBS. Of 29 studies, 11 of 29 had disease detected only with FDG-PET, 8 of 29 were detected only with 131I TBS, and 10 of 29 were detected by both. Five sites were found by FDG-PET and not by 99mTc sestamibi. FDG-PET may be useful in patients in whom 131I TBS is not feasible owing to a history of iodine exposure; similarly, its use would not preclude CT scanning (with contrast if desired) as an additional means to image tumors.
A larger study by Schluter et al. [70] described 118 PET scans in 64 scan-negative/Tg-positive patients. Of 64 patients, 44 had positive PET studies, 34 of whom were proven to be true positives, leading to an altered therapeutic approach in 19 of 34 (surgery and/or external irradiation), whereas 20 patients had negative scans. Their results indicated a positive predictive value (PPV) for PET of 83 % but a negative predictive value (NPV) of only 25 %. In seven patients, there was so much metastatic disease identified that a palliative, rather than curative, approach was taken. Yet, for the most part, they found PET to be a valuable adjunct to identify patients who could benefit from further therapy. Wang and coworkers [71] reported good results with PET scanning in 37 patients with negative 131I scans. PET identified occult lesions in 71 %, with a 92 % PPV in patients with high serum Tg and a 93 % NPV in patients with lower Tg levels. Chung et al. [72] reported excellent utility of FDG-PET scanning in 54 patients with negative 131I scans after thyroxine withdrawal, and they demonstrated a 94 % PPV and a 93 % NPV. FDG-PET may be particularly useful when both the iodine scan and serum Tg are false-negatives, and this often applies when the low-serum Tg is a result of interfering antithyroglobulin antibodies. Chung et al. noted that this often implied regional lymph node involvement. Although Wang et al. [71] found that FDG-PET was not that useful in detecting small degrees of residual papillary tumor in the neck, Chung et al. showed PET to be positive more often with neck disease and conventional scanning than in detecting pulmonary metastases, and Ozkan et al. found that the best success with PET imaging occurred in the patients with the highest Tg antibody levels [73]. Comparably encouraging results have been reported by Helal et al. [74] in a series of 37 scan-negative/Tg-positive patients with DTC. In a group of ten patients with known metastases via conventional imaging, PET confirmed tumor at 17 of 18 sites and identified tumors at 11 additional sites. PET was positive in 19 of 27 patients in a second group with negative imaging by other methods. These findings led to a change in treatment management in 29 of 37 patients, with 23 receiving further surgery and 14 of 23 achieving disease-free status. These authors proposed PET as the “first-line investigation” in scan-negative/Tg-positive patients. A more pessimistic view was expounded by van Tol and colleagues in a letter to the editor [75] in which they described an extraordinary high rate of false-positives (64 %) with PET and that the findings led to a significant change in management in only 1 of 11 (9 %) patients. A drawback is the lack of widespread availability of PET scanners because of their high cost, and, most importantly, these scans are currently not reimbursed by most insurers in the United States except for specific indications. (This situation has been improving steadily over the past few years.)
Fridrich et al. [76] compared FDG-PET to 99mTc-MIBI and 131I TBS and found both to be more sensitive than 131I TBS, with a slight edge in favor of 99mTc-MIBI. In addition to the benefit of good uptake, independent of the patients’ serum TSH level, FDG-PET or MIBI did not have the propensity for high background in the neck, mediastinum, and chest as with 131I and could be used more effectively to detect small metastases in these areas. In contrast, the liver and brain demonstrate high FDG uptake, and the ability to detect metastases in these areas is limited with this agent. Indeed, Feine et al. [77] were able to localize and identify positive neck metastases with FDG-PET in six patients with elevated serum Tg levels. Dietlein et al. proposed a more conservative perspective regarding the utility of FDG-PET scanning [78]. They observed positive FDG-PET images in 7 of 21 patients with positive lymph node metastases but negative 131I TBS; sensitivity was 82 % in patients with high serum Tg but negative TBS. They concluded that FDG-PET should not be used in lieu of 131I-TBS but would serve as a useful adjunct or complement to evaluation, particularly when the 131I TBS was negative in conflict with a rising or elevated level of serum Tg. Altenvoerde et al. [79] performed PET studies in 12 of 32 patients with scan-negative/Tg-positive findings, and the PET scans were positive in 6 of 12. Interestingly, the mean Tg level in the six positive patients was 147 ± 90, whereas the PET-negative patients had a mean Tg of only 9 ± 7.6, suggesting that PET is most useful in those patients with more aggressive and/or larger metastases. Similar conclusions were reached by Grunwald et al. [80] and Wang et al. [81]. In this regard, PET-positive patients with larger volume disease have a worse prognosis [71, 82]. The association of PET positivity with dedifferentiation was commented upon by Caobelli et al. in a communication [83] that discussed a report by Vural et al. [84] linking prognosis to PET findings. The latter workers described their experience with 105 scan-negative/Tg-positive patients and noted that the best prognosis was associated with Tg levels that were suppressible by levothyroxine in PET-negative patients whereas PET positivity correlated with recurrence and extrathyroidal spread. A correlation of better prognosis with negative FDG-PET was also noted by Pachon-Garrudo et al. [85].
Clearly, PET scanning may miss some types of metastases. In the report of Hung et al. [86], 20 scan-negative/Tg-positive patients underwent FDG-PET scanning with lesions detected in 17 of 20, but PET scans were negative in 2 patients proven to have miliary distribution of pulmonary micrometastases. The authors suggest imaging with chest CT scans in such cases. Most valuable has been the newest technology that combines PET scanning with CT or MRI by either fusion software or preferably within one dedicated PET/CT scanner [58, 59]. An overlay of high SUV on a CT or MR image provides much greater specificity that the imaged finding is cancer. As with many diagnostic modalities, negative findings would not preclude disease, but positive findings on PET/CT would dictate CT-guided or ultrasound-guided FNA cytology and then surgery if tumor is confirmed. As mentioned, the utility of FDG-PET scanning in this group of patients tends to correlate with the magnitude of the serum Tg level. For example, Na et al. [64] noted a sensitivity of PET of 28.6 % with Tg levels between 2 and 5, 57.1 % with levels between 5 and 10 and 86 % when Tg was equal to or greater than 20 ng/mL.
TSH Stimulation of FDG-PET
Whether FDG uptake and imaging might be enhanced by either endogenous TSH after levothyroxine withdrawal or by recombinant human TSH (rhTSH) remains somewhat controversial. It appears that the effect of TSH stimulation was best seen in patients with the highest levels of Tg suggesting greater tumor mass, and it has been proposed that FDG-PET may be most useful above a threshold Tg level of 10 ng/mL [87]. Some workers relate the basis for TSH stimulation to in vitro thyroid cell culture studies in which TSH will increase uptake of both FDG [88] and 201Tl [89]; however, that does not prove that thyroid cancer cells will respond in the same way in vivo. TSH could probably improve imaging because TSH stimulates glucose transport into the cells and cellular metabolism.
The best proof of this concept is derived from several clinical studies. For example, Chin et al. [90] evaluated seven patients who were scan-negative/Tg-positive and compared PET scans of those patients on thyroxine suppression to PET scans after rhTSH. The scans after rhTSH disclosed more lesions, and the average tumor-to-background (T:B) ratio values were higher. Similar results were seen by Petrich et al. [91] in 30 patients with largely negative radioiodine scans. rhTSH stimulation provided higher T:B ratios and uncovered more lesions in more patients. Comparing PET scans after thyroxine withdrawal (not rhTSH) to PET scans of patients on suppressive therapy, both Moog et al. [92] and van Tol et al. [93] concluded that TSH stimulation detected more lesions. Rational patient selection is needed, and clinicians and payors have to determine whether the additional cost burden of rhTSH to an already expensive PET scan will constitute a favorable cost–benefit ratio. After an analysis of seven prospective controlled clinical trials that included a total of 168 patients, Ma et al. [94] concluded that TSH stimulation was beneficial in demonstrating more PET-positive lesions but that the findings were associated with altered clinical management in less than 10 % of the patients. Notwithstanding the results of these studies indicating improved imaging with TSH stimulation, Bertagna et al. [66] found no difference in the utility of FDG-PET in patients on levothyroxine vs those with an elevated TSH level. Unrelated to PET scanning but relevant to rhTSH, there is a case report of a scan-negative/Tg-positive patient in whom radioiodine trapping was restored after a period of levothyroxine withdrawal and rhTSH stimulation [95]. Occasionally, radioiodine trapping may also be restored after chemotherapy [96], and the matter of redifferentiation is discussed in Chap. 86.
Somatostatin Imaging
Because some thyroid cancers—especially medullary thyroid cancer [97]—contain somatostatin receptors, somatostatin receptor scintigraphy (SRS) with octreotide or octreotide derivatives has been studied and reported [98, 99]. These agents have also been employed for therapy [100, 101]. Of 25 patients with DTC and elevated serum Tg levels studied by Baudin [98], 16 of 25 had negative 131I TBS, and SRS was positive in 12 of these patients as well as in 8 of 9 patients with positive 131I TBS. Stokkel et al. [102] studied ten Tg-positive/131I scan-negative patients with octreotide scanning and described multiple metastases in nine of ten. Based on octreotide uptake, the authors speculate that 111-In-labeled octreotide or its analogues might be useful for therapy of such patients as it has been shown to be for medullary thyroid carcinoma [97]. Sarlis et al. [103] compared octreotide scanning to PET and conventional imaging in 21 patients with aggressive disease, finding that octreotide had only moderate sensitivity yet detected disease in 5 patients who were negative by PET and other imaging. In preliminary studies, Sager et al. [104] examined a group of radioiodine scan-negative patients employing technetium-labeled octreotide analogues 99mTc-HYNIC-TOC and HYNIC-TATE and proposed that these agents could be used routinely in patients who are somatostatin receptor positive. In a comparison to FDG-PET scanning, Middendorp et al. [105] employed [(68)Ga]DOTATOC and found comparable diagnostic utility although FDG-PET did exhibit a slightly higher rate of lesion detection. Confirmatory studies are required, but SRS with labeled octreotide or one of these newer derivatives may represent another useful alternative to 131-I TBS or FDG-PET, with the advantage of lacking the need to withdraw TSH-suppressive levothyroxine therapy.
Surgery
As mentioned above, ultrasonography of the neck is extremely useful to identify occult metastases of papillary thyroid cancer [106, 107] and deserves to be an almost routine imaging tool for this purpose (see Chaps. 21 and 37), particularly if used in conjunction with rhTSH-stimulated thyroglobulin levels [108]. Another approach has been to proceed with cervical exploration and node dissection in the case of papillary carcinoma, even when all additional imaging studies are unrevealing. In one such series of 21 patients, Alzahrani et al. [109] performed neck dissections after confirming the presence of tumor by ultrasound-guided fine-needle aspiration cytology. Postoperatively, serum Tg fell from a mean of 185 ± 79 to 127 ± 59, with 4 patients who achieved remission, 13 who had persistent disease, and 4 who showed progression. They concluded that the additional surgery offered benefit in a minority of patients and that most remained stable during follow-up. In addition, the intraoperative use of ultrasound can readily improve the detection of residual or recurrent thyroid cancer [110], and there has been greater success with positive node dissections since its use by surgeons at our institution. In some hands the combined use of (18)F-FDG PET/CT imaging and gamma probe radio-guided surgery has proved successful for the localization and confirmation of metastatic lesions in the radioiodine scan-negative patient [111].
The opposite Scenario: Undetectable TG and Positive 131-I Scan
In recent years, the opposite even more puzzling scenario has been noted, that of finding patients with positive uptake seen on follow-up radionuclide scans indicating the likelihood of residual disease but having undetectable levels of serum Tg [112–114]. While this situation is relatively infrequent in our experience, it has been reported more frequently by others, with undetectable Tg levels observed in 20 % of patients who were thought to have residual benign thyroid remnants and in 8 % who were identified as having residual thyroid carcinoma [112]. Significant metastatic disease has been found in some of these patients, typically to regional lymph nodes, leading some investigators to advocate for more routine performance of survey radioiodine scans [114], perhaps as part of periodic rhTSH stimulation testing with both Tg levels and scan post-stimulation [112]. On the other hand, in a retrospective review of 389 patients of whom 44 (11.3 %) had positive scans and undetectable Tg levels, Lim et al. [115] found that long-term clinical outcomes did not differ in these patients and suggested that repetitive radioiodine scans were not necessary and that conservative monitoring would suffice for long-term follow-up.
We believe that the explanation for this clinical situation in most cases is related to false-negative results for serum Tg. In recent years, we have begun to understand better the reasons for differences in results between various ICMA or RIA methodologies for both serum Tg and anti-Tg antibodies, on the basis of differences in assay reagents or assay “hook effects,” and the presence of different idiotypic antithyroglobulin antibodies in the patient’s serum [116]. Both interfering anti-Tg antibodies and heterophile antibodies have been incriminated [113, 117]. Our suggestion to clinicians facing this dilemma is to obtain Tg and Tg antibody measurements in several different laboratories, by both ICMA and RIA methodology. Doing so is likely to discover the presence of antibodies detected in one system that were not detected in the original assay, thereby accounting for the discordant results.
Conclusions
In conclusion, how should the scan-negative/Tg-positive patient be managed with no underlying reason to suspect either false-negative scan or false-positive serum Tg level? Schlumberger [118, 119] has advocated empiric administration of 100 mCi 131I to any patient with a Tg level more than 10 ng/mL while off levothyroxine and would only repeat the 131I whole-body scan every 2–5 years when the Tg level is in the range of 1–10 ng/mL. As mentioned above, no studies show improved survival with this approach. On the contrary, the follow-up study of van Tol et al. [120] indicates little support for empiric therapy based on an average follow-up period of 4.2 years. Of 56 patients given a “blind” dose of 150 mCi of 131I, uptake was revealed on the posttreatment scan in 28 of 56 (50 %) of the patients with no difference in serum Tg levels. They concluded that therapy had no salutary effect on survival or reduction of tumor burden. There may be many factors that could differentiate those patients who seem to respond to empiric therapy from those who do not. One factor could be the size of the lesions as micrometastases may be more readily ablated than macrometastases [121]. At this point in our knowledge, I am attracted to the concept of individualization of empiric therapy as proposed by Ma et al. [39] based upon the height of the Tg levels and whether or not they are seen to be increasing during follow-up. Indeed, although controversial as discussed above and elsewhere [14], empiric radioiodine therapy can be attempted at least one more time in selected patients with Tg levels >10 ng/mL or rising. Posttherapy Tg levels and the posttherapy scan should be examined to assess potential benefit. In an attempt to maximize the efficacy of the radioiodine, I employ a low-iodine diet for 3 weeks prior to therapy supplemented by low-dose diuretic (20 mg/day furoseamide) and confirm the patient’s adherence to the diet by measurement of urinary iodine 1 week prior to the planned therapy. We proceed with therapy if the urinary iodide is less than 50 μg/L, and most patients can achieve levels of less than 20 μg/L. In addition, radioiodine retention and presumed therapeutic benefit can be augmented by lithium carbonate therapy as discussed in Chap. 49. The growing number of reports [32, 37–39, 121, 122] indicating benefit of empiric therapy appears to justify this approach.
If radioiodine therapy is not to be given in the face of clearly measurable Tg levels, alternative imaging procedures should be encouraged. For papillary thyroid carcinoma with a propensity to regional recurrence, that could include ultrasound, CT, MRI, 99mTc-MIBI, or FDG-PET, with fine-needle aspiration cytology when feasible to confirm the diagnosis. For follicular thyroid cancer with its propensity for distant metastases (especially to bone and lung), imaging with PET/CT, 99mTc tetrafosmin, 99mTc-HMDP, or 201Tl could be attempted. Identification of isolated distant lesions by these methods would allow earlier intervention by surgical excision, a local ablative technique, or external radiotherapy instead of delaying further treatment until a subsequent 131-I TBS might become positive or serum Tg levels might increase further because of further tumor growth. In patients with higher risk disease following early total thyroidectomy and high-dose radioiodine ablation, this approach should permit effective management until more target-specific tumoricidal therapies become available.
References
Spencer CA. New insights for using serum thyroglobulin (Tg) measurement for managing patients with differentiated thyroid carcinomas. Thyroid Int. 2003;4:1–14.
Spencer CA, Fatemi S, Singer P, Nicoloff J, LoPresti J. Serum basal thyroglobulin measured by a second-generation assay correlates with the recombinant human thyrotropin-stimulated thyroglobulin response in patients treated for differentiated thyroid cancer. Thyroid. 2010;20:587–95.
Schlumberger M, Hitzel A, Toubert ME, et al. Comparison of seven serum thyroglobulin assays in the follow-up of papillary and follicular thyroid cancer patients. J Clin Endocrinol Metab. 2007;92:2487–95.
Nascimento C, Borget I, Troalen F, Al Ghuzlan A, Deandreis D, Hartl D, et al. Ultrasensitive serum thyroglobulin measurement is useful for the follow-up of patients treated with total thyroidectomy without radioactive iodine ablation. Eur J Endocrinol. 2013;169(5):689–93. doi:10.1530/EJE-13-0386.
Giovanella L, Teglia G, Sadeghi R, Trimboli P, Ceriani L, Verburg FA. Unstimulated high-sensitive thyroglobulin in follow-up of differentiated thyroid cancer patients: a meta-analysis. J Clin Endocrinol Metab. 2014;99:440–7.
Lee JI, Chung YJ, Cho BY, Chong S, Seok JW, Park SJ. Postoperative-stimulated serum thyroglobulin measured at the time of 131I ablation is useful for the prediction of disease status in patients with differentiated thyroid carcinoma. Surgery. 2013;153(6):828–35.
Wartofsky L. Management of scan negative thyroglobulin positive differentiated thyroid carcinoma. J Clin Endocrinol Metab. 1998;83:4195–9.
Giovanella L, Treglia G, Ceriani L, Verburg F. Detectable thyroglobulin with negative imaging in differentiated thyroid cancer patients: what to do with negative anatomical imaging and radioiodine scan? Nucl Med. 2014;53:1–10.
Clark OH, Hoelting T. Management of patients with differentiated thyroid cancer who have positive serum thyroglobulin levels and negative radioiodine scans. Thyroid. 1994;4:501–5.
McDougall IR. Management of thyroglobulin positive/whole-body scan negative: is 131-I therapy useful? J Endocrinol Invest. 2001;24:194–8.
Levy EG, Fatourechi V, Robbins R, Ringel MD. Thyroglobulin-positive, radioiodine-negative thyroid cancer. Thyroid. 2001;11:599–602.
Gemsenjager E. Thyroglobulin-positive, radioiodine-negative thyroid cancer (letter to editor). Thyroid. 2003;13:833–4.
Hurley JR. Management of thyroid cancer: radioiodine ablation, “stunning”, and treatment of thyroglobulin-positive, (131)I scan-negative patients. Endocr Pract. 2000;6:401–6.
Mazzaferri EL. Empirically treating high serum thyroglobulin levels. J Nucl Med. 2005;46:1079–88.
Tuttle RM, Ball DW, Byrd D, Dilawari RA, Doherty GM, Duh QY, et al. Thyroid carcinoma. National Comprehensive Cancer Network. 2012. Version 3.2012. Available from: http://www.nccn.org/professionals/physician_gls/pdf/thyroid.pdf. Accessed 24 Aug 2012.
British Thyroid Association, Royal College of Physicians. Guidelines for the management of thyroid cancer. In: Perros P, editor. Report of the thyroid cancer guidelines update group. 2nd ed. London: Royal College of Physicians; 2007.
Pacini F, Lippi F, Formica N, et al. Therapeutic doses of iodine-131 reveal undiagnosed metastases in thyroid cancer patients with detectable serum thyroglobulin levels. J Nucl Med. 1987;28:1888–91.
Wartofsky L, Sherman SI, Gopal J, et al. The use of radioactive iodine in patients with papillary and follicular thyroid cancer. J Clin Endocrinol Metab. 1998;83:4195–203.
Haugen BR, Alexander EK, Bible KC, Doherty GM, Mandel SJ, Nikiforov Y, et al. 2015 American Thyroid Association Guidelines for patients with thyroid nodules and differentiated thyroid cancer. Available online at Thyroid 25: DOI:10.1089/thy.2015.0020; print version in Thyroid 26:1–133, 2016.
Spencer CA. Clinical utility of thyroglobulin antibody (TgAb) measurements for patients with differentiated thyroid cancers (DTC). J Clin Endocrinol Metab. 2011;96:3615–27.
Spencer CA, Petrovic I, Fatemi S. Current thyroglobulin autoantibody (TgAb) assays often fail to detect interfering TgAb that ca result in the reporting of falsely low/undetectable serum Tg IMA values for patients with differentiated thyroid cancer. J Clin Endocrinol Metab. 2011;96:1283–91.
Verburg FA, Luster M, Cupini C, et al. Implications of thyroglobulin antibody positivity in patients with differentiated thyroid cancer: a clinical position paper. Thyroid. 2013;23:1211–25.
Spencer CA. Commentary on: implications of thyroglobulin antibody positivity in patients with differentiated thyroid cancer: a clinical position paper. Thyroid. 2013;23:1190–2.
Zanotti-Fregonara P, Keller I, Calzada-Nocaudie M, Al-Nahhas A, Devaux JY, Grassetto G, et al. Increased serum thyroglobulin levels and negative imaging in thyroid cancer patients: are there sources of benign secretion? A speculative short review. Nucl Med Commun. 2010;31(12):1054–8.
Maxon HR, Thomas SR, Boehringer A, et al. Low iodine diet in I-131 ablation of thyroid remnants. Clin Nucl Med. 1983;8:123–6.
Klutmann S, Jenicke L, Geiss-Tonshoff M, et al. Prevalence of iodine-and thyroglobulin-negative findings in differentiated thyroid cancer. A retrospective analysis of patients treated from 1951 to 1998 in university hospital. Nuklearmedizin. 2001;40:143–7.
Padovani RP, Robenshtok E, Brokhin M, Tuttle RM. Even without additional therapy, serum thyroglobulin concentrations often decline for years after total thyroidectomy and radioactive remnant ablation in patients with differentiated thyroid cancer. Thyroid. 2012;22:778–83.
Pacini F, Agate L, Elisei R, et al. Outcome of differentiated thyroid cancer with detectable serum Tg and negative diagnostic (131)I whole body scan: comparison of patients treated with high (131)I activities versus untreated patients. J Clin Endocrinol Metab. 2001;86:4092–7.
Frank RW, Middleton L, Stack Jr BC, Spencer HJ, Riggs AT, Bodenner DL. Conservative management of thyroglobulin positive, nonlocalizable thyroid carcinoma. Head Neck. 2014;36:155–7.
Schlumberger M, Mancusi F, Baudin E, Pacini F. 131-I therapy for elevated thyroglobulin levels. Thyroid. 1997;7:273–6.
Pineda JD, Lee T, Ain K, et al. Iodine-131 therapy for thyroid cancer patients with elevated thyroglobulin and negative diagnostic scan. J Clin Endocrinol Metab. 1995;80:1488–92.
Robbins J. Management of thyroglobulin-positive, body scan-negative thyroid cancer patients: evidence for the utility of I-131 therapy. J Endocrinol Investig. 1999;22:808–10.
De Keizer B, Koppeschaar HP, Zelissen PM, et al. Efficacy of high therapeutic doses of iodine-131 in patients with differentiated thyroid cancer and detectable serum thyroglobulin. Eur J Nucl Med. 2001;28:198–202.
McDougall IR. 131-I treatment of 131-I negative whole body scan, and positive thyroglobulin in differentiated thyroid carcinoma: what is being treated? Thyroid. 1997;7:669–72.
Mazzaferri EL. Editorial: treating high thyroglobulin with radioiodine: a magic bullet or a shot in the dark? J Clin Endocrinol Metab. 1995;80:1485–7.
Fatourechi V, Hay ID, Javedan H, et al. Lack of impact of radioiodine therapy in Tg-positive, diagnostic whole-body scan-negative patients with follicular cell-derived thyroid cancer. J Clin Endocrinol Metab. 2002;87:1521–6.
Fatourechi V, Hay ID. Treating the patient with differentiated thyroid cancer with thyroglobulin-positive iodine-131 diagnostic scan-negative metastases: including comments on the role of serum thyroglobulin monitoring in tumor surveillance. Semin Nucl Med. 2000;30:107–14.
Webb RC, Howard RS, Stojadinovic A, Gaitonde DY, Wallace MK, Ahmed J, Burch HB. The utility of serum thyroglobulin measurement at the time of remnant ablation for predicting disease-free status in patients with differentiated thyroid cancer: a meta-analysis involving 3947 patients. J Clin Endocrinol Metab. 2012;97:2754–63.
Koh JM, Kim ES, Ryu JS, et al. Effects of therapeutic doses of 131-I in thyroid papillary carcinoma patients with elevated thyroglobulin level and negative 131-I whole-body scan: comparative study. Clin Endocrinol. 2003;58:421–7.
Ma C, Xie J, Kuang A. Is empiric 131-I therapy justified for patients with positive thyroglobulin and negative 131-K whole-body scanning results? J Nucl Med. 2005;46:1164–70.
Ford D, Giridharan S, McConkey C, et al. External beam radiotherapy in the management of differentiated thyroid cancer. Clin Oncol. 2003;15:337–41.
Brierley JD, Tsang RW. External-beam radiation therapy in the treatment of differentiated thyroid cancer. Semin Surg Oncol. 1999;16:42–9.
Alam MS, Takeuchi R, Kasagi K, et al. Value of combined technetium-99m hydroxy methylene diphosphonate and Thallium-201 imaging in detecting bone metastases from thyroid carcinoma. Thyroid. 1997;7:705–12.
Almeida-Filho P, Ravizzini GC, Almeida C, et al. Whole-body Tc-99m sestamibi scintigraphy in the follow-up of differentiated thyroid carcinoma. Clin Nucl Med. 2000;25:443–6.
Carril JM, Quirce R, Serrano J, et al. Total body scintigraphy with thallium-201 and iodine-131 in the follow-up of differentiated thyroid cancer. J Nucl Med. 1997;38:686–92.
Dadparvar S, Chevres A, Tulchinsky M, et al. Clinical utility of technetium-99m methoxisobutylisonitrile imaging in differentiated thyroid carcinoma: comparison with thallium-201 and iodine-131 scintigraphy and serum thyroglobulin quantitation. Eur J Nucl Med. 1995;22:1330–8.
Lorberboym M, Murthy S, Mechanick JI, et al. Thallium-201 and iodine-131 scintigraphy in differentiated thyroid carcinoma. J Nucl Med. 1996;37:1487–91.
Ugur O, Kostakoglu L, Caner B, et al. Comparison of 201-Tl, 99mTcMIBI and 131-I imaging in the follow-up of patients with well differentiated thyroid carcinoma. Nucl Med Commun. 1996;17:373–7.
Harder W, Lind P, Molnar M, et al. Thallium-201 uptake with negative iodine-131 scintigraphy and serum thyroglobulin in metastatic oxyphilic papillary thyroid carcinoma. J Nucl Med. 1998;39:236–8.
Rodrigues M, Li S, Gabriel M, Heute D, Greifendeder M, Virgolini I. 99m-Tc-depreotide scintigraphy versus 18F-FDG-PET in the diagnosis of radioiodine-negative thyroid cancer. J Clin Endocrinol Metab. 2006;91:3997–4000.
Elser H, Henze M, Hermann C, et al. 99m-Tc-MIBI for recurrent and metastatic differentiated thyroid carcinoma. Nuklearmedizin. 1997;36:7–12.
Lind P, Gallowitsch HJ. The use of non-specific tracers in the follow up of differentiated thyroid cancer: results with Tc-99m tetrofosmin whole body scintigraphy. Acta Med Aust. 1996;23:69–75.
Gallowitsch HJ, Kresnik E, Mikosch P, et al. Tc-99m-tetrafosmin scintigraphy: an alternative scintigraphic method for following up differentiated thyroid carcinoma—preliminary results. Nuklearmedizin. 1996;35:230–5.
Lind P, Gallowitsch HJ, Langsteger W, et al. Technetium-99m-tetrafosmin whole-body scintigraphy in the follow-up of differentiated thyroid carcinoma. J Nucl Med. 1997;38:348–52.
van Dijk D, Plukker JTM, Phan HTT, et al. 18-fluorodeoxyglucose positron emission tomography in the early diagnostic workup of differentiated thyroid cancer patients with a negative post-therapeutic iodine scan and detectable thyroglobulin. Thyroid. 2013;23(8):1003–9.
Iagaru A, Kalinyak JE, McDougall IR. F-18 FDG PET/CT in the management of thyroid cancer. Clin Nucl Med. 2007;32(9):690–5.
Choi WH, Chung YA, Han EJ, Sohn HS, Lee SH. Clinical value of integrated [18F]fluoro-2-deoxy-D-glucose positron emission tomography/computed tomography in the preoperative assessment of papillary thyroid carcinoma: comparison with sonography. J Ultrasound Med. 2011;30(9):1267–73.
Schoder H, Yeung HWD. Positron emission imaging of head and neck cancer, including thyroid carcinoma. Semin Nucl Med. 2004;34:180–97.
Ota N, Kato K, Iwano S, et al. Comparison of 18F-fluoride PET/CT, 18F-FDG PET/CT, and bone scintigraphy (planar and SPECT) in detection of bone metastases of differentiated thyroid cancer: a pilot study. Brit J Radiol. 2014;87(1034):20130444.
Nakajo M, Jinguji M, Tani A, et al. Diagnosis of metastases from postoperative differentiated thyroid cancer: comparison between FDG and FLT PET/CT studies. Radiology. 2013;267(3):891–901.
deGroot JW, Links TP, Jager PL, et al. Impact of 18F-fluoro-2-D-glucose positron emission tomograph (FDG-PET) in patients with biochemical evidence of recurrent or residual medullary thyroid cancer. Ann Surg Oncol. 2004;11:786–94.
Bannas P, Derlin T, Groth M, Apostolova I, Adam G, Mester J, Klutmann S. Can (18)F-FDG-PET/CT be generally recommended in patients with differentiated thyroid carcinoma and elevated thyroglobulin levels but negative I-131 whole body scan? Ann Nucl Med. 2012;26(1):77–85.
Bertagna F, Biasiotto G, Orlando E, Bosio G, Giubbini R. Role of 18F-fluorodeoxyglucose positron emission tomography/computed tomography in patients affected by differentiated thyroid carcinoma, high thyroglobulin level, and negative 131I scan: review of the literature. Jpn J Radiol. 2010;28(9):629–36.
Muros MA, Llamas-Elvira JM, Ramirez-Navarro A, et al. Utility of fluorine-18-fluorodeoxyglucose positron emission tomography in differentiated thyroid carcinoma with negative radioiodine scans and elevated serum thyroglobulin levels. Am J Surg. 2000;179:457–61.
Na SJ, Yoo IR, O JH, Lin C, Lin Q, Kim SH, Chung SK. Diagnostic accuracy of (18)F-fluorodeoxyglucose positron emission tomography/computed tomography in differentiated thyroid cancer patients with elevated thyroglobulin and negative (131)I whole body scan: evaluation by thyroglobulin level. Ann Nucl Med. 2012;26(1):26–34.
Creach KM, Nussenbaum B, Siegel BA, Grigsby PW. Thyroid carcinoma uptake of 18F-fluorodeoxyglucose in patients with elevated serum thyroglobulin and negative 131I scintigraphy. Am J Otolaryngol. 2013;34(1):51–6.
Bertagna F, Bosio G, Biasiotto G, Rodella C, Puta E, Gabanelli S, et al. F-18 FDG-PET/CT evaluation of patients with differentiated thyroid cancer with negative I-131 total body scan and high thyroglobulin level. Clin Nucl Med. 2009;34(11):756–61.
Dong MJ, Liu ZF, Zhao K, Ruan LX, Wang GL, Yang SY, Sun F, Luo XG. Value of 18F-FDG-PET/PET-CT in differentiated thyroid carcinoma with radioiodine-negative whole-body scan: a meta-analysis. Nucl Med Commun. 2009;30(8):639–50.
Kim SJ, Lee TH, Kim IJ, Kim YK. Clinical implication of F-18 FDG PET/CT for differentiated thyroid cancer in patients with negative diagnostic iodine-123 scan and elevated thyroglobulin. Eur J Radiol. 2009;70(1):17–24.
Grunwald F, Menzel C, Bender H, et al. Comparison of 18FDG-PET with 131-Iodine and 99m-Tc-sestamibi scintigraphy in differentiated thyroid cancer. Thyroid. 1997;7:327–35.
Schluter B, Bohuslavizki KH, Beyer W, et al. Impact of FDG PET on patients with differentiated thyroid cancer who present with elevated thyroglobulin and negative 131I scan. J Nucl Med. 2001;42:71–6.
Wang W, Macapinlac H, Larson SM, et al. [18F]-2-fluoro-2-deoxy-Dglucose positron emission tomography localizes residual thyroid cancer in patients with negative diagnostic (131)I whole body scans and elevated serum thyroglobulin levels. J Clin Endocrinol Metab. 1999;84:2291–302.
Chung JK, So Y, Lee JS, et al. Value of FDG-PET in papillary thyroid carcinoma with negative 131-I whole body scan. J Nucl Med. 1999;40:986–92.
Ozkan E, Aras G, Kucuk NO. Correlation of 18F-FDG PET/CT findings with histopathological results in differentiated thyroid cancer patients who have increased thyroglobulin or antithyroglobulin antibody levels and negative 131I whole-body scan results. Clin Nucl Med. 2013;38(5):326–31.
Helal BO, Merlet P, Toubert ME, et al. Clinical impact of (18)F-FDG PET in thyroid carcinoma patients with elevated thyroglobulin levels and negative (131)I scanning results after therapy. J Nucl Med. 2001;42:1464–9.
van Tol KM, Jager PL, Dullaart RP, Links TP. Follow-up in patients with differentiated thyroid carcinoma with positive 18F-fluoro-2-deoxy-Dglucose-positron emission tomography results, elevated thyroglobulin levels, and negative high-dose 131I posttreatment whole body scans. J Clin Endocrinol Metab. 2000;85:2082–3.
Fridrich L, Messa C, Landoni C, et al. Whole-body scintigraphy with 99m-TC-MIBI, 18F-FDG and 131-I in patients with metastatic thyroid carcinoma. Nucl Med Commun. 1997;18:3–9.
Feine U, Lietzenmayer R, Hanke JP, et al. Fluorine-18-FDG and iodine-131 uptake in thyroid cancer. J Nucl Med. 1996;37:1468–72.
Dietlein M, Scheidhauer K, Voth E, et al. Fluorine-18 fluorodeoxyglucose positron emission tomography and iodine-131 whole-body scintigraphy in the follow-up of differentiated thyroid cancer. Eur J Nucl Med. 1997;24:1342–8.
Altenvoerde G, Lerch H, Kuwert T, et al. Positron emission tomography with F-18-deoxyglucose in patients with differentiated thyroid carcinoma, elevated thyroglobulin levels, and negative iodine scans. Langenbeck's Arch Surg. 1998;383:160–3.
Grunwald F, Kalicke T, Feine U, et al. (18)F-FDG PET scanning in patients with thyroid cancer: results of a multicentre study. Eur J Nucl Med. 1999;26:1547–52.
Wang W, Larson SM, Fazzari M, et al. Prognostic value of (18)F-FDG PET scanning in patients with thyroid cancer. J Clin Endocrinol Metab. 2000;85:1107–13.
Marcus CS. Prognostic significance of FDG PET/CT on the follow-up of patients of differentiated thyroid carcinoma with negative I131 whole- body scan and elevated thyroglobulin levels. Clin Nucl Med. 2013;38(3):195.
Caobelli F, Pizzocaro C, Guerra UP. Prognostic significance of FDG PET/CT on the follow-up of patients of differentiated thyroid carcinoma with negative I131 whole-body scan and elevated thyroglobulin levels. Clin Nucl Med. 2013;38(3):196.
Vural GU, Akkas BE, Ercakmak N, Basu S, Alavi A. Prognostic significance of FDG PET/CT on the follow-up of patients of differentiated thyroid carcinoma with negative 131I whole-body scan and elevated thyroglobulin levels: correlation with clinical and histopathologic characteristics and long-term follow-up data. Clin Nucl Med. 2012;37(10):953–9.
Pachon-Garrudo VM, Cuenca-Cuenca JI, Ruiz-Franco-Baux J, Borrego-Dorado I, Tirado-Hospital JL, Navarro-Gonzalez E, Vazquez-Albertino R. Value of the negative PET-FDG in the middle term follow-up of differentiated thyroid cancer in patients with negative 131 I-Na scan and elevated thyroglobulin serum levels. Rev Esp Med Nucl Imag Mol. 2012;31(6):315–21.
Hung MC, Wu HS, Kao C, et al. F18-fluorodeoxyglucose positron emission tomography in detecting metastatic papillary thyroid carcinoma with elevated human serum thyroglobulin levels but negative I-131 whole body scan. Endocr Res. 2003;29:169–75.
Vera P, Kuhn-Lansoy C, Sanson AE, Hapdey S, Modzelewski R, Hitzel A, et al. Does recombinant human thyrotropin-stimulated positron emission tomography with [18F]fluoro-2-deoxy-D-glucose improve detection of recurrence of well-differentiated thyroid carcino a in patients with low serum thyroglobulin? Thyroid. 2010;20:15–23.
Deichen JT, Schmidt C, Prante O, et al. Influence of TSH on uptake of [18F]fluorodeoxyglucose in human thyroid cells in vitro. Eur J Nucl Med Mol Imaging. 2004;31:507–12.
Mruck S, Pfahlberg A, Papadopoulos T, et al. Uptake of 201TI into primary cell cultures from human thyroid tissue is multiplied by TSH. J Nucl Med. 2002;43:145–52.
Chin BB, Patel P, Chhade C, et al. Recombinant human thyrotropin stimulation of fluoro-d-glucose positron emission tomography uptake in well-differentiated thyroid carcinoma. J Clin Endocrinol Metab. 2004;89:91–5.
Petrich T, Borner AR, Otto D, et al. Influence of rhTSH on [18-F] fluorodeoxyglucose uptake by differentiated thyroid carcinoma. Eur J Nucl Med Mol Imag. 2002;29:641–7.
Moog F, Linke R, Manthey N, et al. Influence of thyroid-stimulating hormone levels on uptake of FDG in recurrent and metastatic differentiated thyroid carcinoma. J Nucl Med. 2000;41:1989–95.
van Tol KM, Jager PL, Piers DA, et al. Better yield of 18-fluorodeoxyglucose-positron emission tomography in patients with metastatic differentiated thyroid carcinoma during thyrotropin stimulation. Thyroid. 2002;12:381–7.
Ma C, Xie J, Lou Y, Gao Y, Zuo S, Wang X. The role of TSH for 18F-FDG-PET in the diagnosis of recurrence and metastases of differentiated thyroid carcinoma with elevated thyroglobulin and negative scan: a meta-analysis. Eur J Endocrinol. 2010;163(2):177–83.
Kasner DL, Spieth ME, Starkman ME, Zdor-North D. Iodine-131negative whole body scan reverses to positive after a combination thyrogen stimulation and withdrawal. Clin Nucl Med. 2002;27:772–80.
Morris JC, Kim CK, Padilla MLK, et al. Conversion of non-iodine concentrating differentiated thyroid carcinoma metastases into iodine-concentrating foci after anticancer chemotherapy. Thyroid. 1997;7:63–6.
Lodish M, Dagalakis U, Chen CC, Sinaii N, Whitcomb P, Aikin A, et al. 111-In-octreotide scintigraphy for identification of metastatic medullary thyroid carcinoma in children and adolescents. J Clin Endocrinol Metab. 2012;97:E207–12.
Baudin E, Schlumberger M, Lumbroso J, et al. Octreotide scintigraphy in patients with differentiated thyroid carcinoma: contribution for patients with negative radioiodine scan. J Clin Endocrinol Metab. 1996;81:2541–4.
Haslinghuis LM, Krenning EP, De Herder WW, et al. Somatostatin receptor scintigraphy in the follow-up of patients with differentiated thyroid cancer. J Endocrinol Invest. 2001;24:415–22.
Margulies DJ, Blum M. Somatostatin receptor scintigraphy as a potential diagnostic and treatment modality for thyroid follicular-cell derived cancers. Thyroid. 2010;20:671–2.
Teunissen JJ, Kwekkeboom DJ, Kooij PP, Bakker W, Krenning EP. Peptide receptor radionuclide therapy for non-radioiodine-avid differentiated thyroid cancer. J Nucl Med. 2005;46 Suppl 1:107S–14.
Stokkel MP, Reigman HI, Verkooijen RB, Smit JW. Indium-11Octreotide scintigraphy in differentiated thyroid carcinoma metastases that do not respond to treatment with high-dose I-131. J Cancer Res Clin Oncol. 2003;129:287–94.
Sarlis NJ, Gourgiotis L, Guthrie LC, et al. In-111 DTPA-octreotide scintigraphy for disease detection in metastatic thyroid cancer: comparison with F-18 FDG PET and extensive conventional radiographic imaging. Clin Nucl Med. 2003;28:208–17.
Sager S, Kabasakal L, Halac M, Maecke H, Uslu L, Onsel C, Kanmaz B. Comparison of 99mTc-HYNIC-TATE octreotide scintigraphy with FSG PET and 99mTc-MIBI in local recurrent or distant metastatic thyroid cancers. Clin Nucl Med. 2013;38:321–5.
Middendorp M, Selkinski I, Happel C, Kranert WT, Grunwald F. Comparison of positron emission tomography with [(18)F]FDG and [968] Ga] DOTATOC in recurrent differentiated thyroid cancer: preliminary data. Q J Nucl Med Mol Imag. 2010;54:76–83.
Durante C, Filetti S. Management of papillary thyroid cancer patients in absence of postoperative radioiodine remnant ablation: tailoring follow-up by neck sonography. J Clin Endocrinol Metab. 2011;96:3059–61.
Ito Y, Amino N, Miyauchi A. Thyroid ultrasonography. World J Surg. 2010;34:1171–80.
Pacini F, Molinaro E, Castagna MG, et al. Recombinant human thyrotropin-stimulated serum thyroglobulin combined with neck ultrasonography has the highest sensitivity in monitoring differentiated thyroid carcinoma. J Clin Endocrinol Metab. 2003;88:3668–73.
Alzahrani AS, Raef H, Sultan A, et al. Impact of cervical lymph node dissection on serum TG and the course of disease in TG-positive, radioactive iodine whole body scan-negative recurrent/persistent papillary thyroid cancer. J Endocrinol Invest. 2002;25:526–31.
Karwowski JK, Jeffrey B, McDougall IR, Weigel RJ. Intraoperative ultrasonography improves identification of recurrent thyroid cancer. Surgery. 2002;132:924–9.
Agrawal A, Hall NC, Ringel MD, Povoski SP, Martin Jr EW. Combined use of perioperative TSH-stimulated (18)F-FDG PET/CT imaging and gamma probe radio-guided surgery to localize and verify resection of iodine scan-negative recurrent thyroid carcinoma. Laryngoscope. 2008;118(12):2190–4.
Cherk MH, Francis P, Topliss DJ, Bailey M, Kalff V. Incidence and implications of negative serum thyroglobulin but positive I-131 whole-body scans in patients with well-differentiated thyroid cancer prepared with rhTSH or thyroid hormone withdrawal. Clin Endocrinol. 2012;76(5):734–40.
Giovanella L, Suriano S, Ceriani L, Verburg FA. Undetectable thyroglobulin in patients with differentiated thyroid carcinoma and residual radioiodine uptake on a postablation whole-body scan. Clin Nucl Med. 2011;36(2):109–12.
Park EK, Chung JK, Lim IH, do Park J, Lee DS, Lee MC, Cho BY. Recurrent/metastatic thyroid carcinomas false negative for serum thyroglobulin but positive by posttherapy I-131 whole body scans. Europ J Nucl Med Molec Imag. 2009;36(2):172–9.
Lim D-J, O JH, Kim M-H, Kim J-H, Kwon H-S, Kim S-H, et al. Clinical significance of observation without repeated radioiodine therapy in differentiated thyroid carcinoma patients with positive surveillance whole-body scans and negative thyroglobulin. Korean J Intern Med. 2010;25:408–14.
Nygaard B, Bentzen J, Laurberg P, Pedersen SM, Bastholt L, Handberg A, et al. Large discrepancy in the results of sensitive measurements of thyroglobulin antibodies in the follow-up on thyroid cancer: a diagnostic dilemma. Eur Thyroid J. 2012;1:193–7.
Giovanella L. False-negative thyroglobulin measurement in recurrent/metastatic thyroid carcinomas. Eur J Nucl Med Mol Imag. 2009;36(2):326–7. author reply 328 [Letter].
Schlumberger MJ. Papillary and follicular thyroid carcinoma. N Engl J Med. 1998;338:297–306.
Schlumberger M, Hay ID. Use of radioactive iodine in patients with papillary and follicular thyroid cancer. J Clin Endocrinol Metab. 1998;83:4195–203.
van Tol KM, Jager PL, deVries EG, et al. Outcome in patients with differentiated thyroid cancer with negative diagnostic whole-body scanning and detectable stimulated thyroglobulin. Eur J Endocrinol. 2003;148:589–96.
Kabasakal L, Selcuk NA, Shafipour H, et al. Treatment of iodine-negative thyroglobulin-positive thyroid cancer; differences in outcome in patients with macrometastases and patients with micrometastases. Eur J Nucl Med Mol Imag. 2004;31:1500–4.
Kamel N, Corapcioglu D, Sahin M, et al. I-131 therapy for thyroglobulin positive patients without anatomical evidence of persistent disease. J Endocrinol Invest. 2004;27:949–53.
Author information
Authors and Affiliations
Corresponding author
Editor information
Editors and Affiliations
Rights and permissions
Copyright information
© 2016 Springer Science+Business Media New York
About this chapter
Cite this chapter
Wartofsky, L. (2016). Management of the Patients with Negative Radioiodine Scan and Elevated Serum Thyroglobulin. In: Wartofsky, L., Van Nostrand, D. (eds) Thyroid Cancer. Springer, New York, NY. https://doi.org/10.1007/978-1-4939-3314-3_47
Download citation
DOI: https://doi.org/10.1007/978-1-4939-3314-3_47
Published:
Publisher Name: Springer, New York, NY
Print ISBN: 978-1-4939-3312-9
Online ISBN: 978-1-4939-3314-3
eBook Packages: MedicineMedicine (R0)