Abstract
The guinea pig sensitized by ovalbumin is the most widely used model to study cough experimentally, as the neurophysiology of the vagus nerve in the guinea pig is closest to humans. Nonetheless, the choice of the antigen remains questionable, which influences the translation of results into clinical medicine. The present study seeks to develop an alternative model of cough study using house dust mite sensitization (HDM). Thirty guinea pigs were divided into the HDM group, ovalbumin (OVA) group, and control group based on their cough response to 0.4 M citric acid. In the HDM group animals were sensitized by 0.25 %HDM aerosol, which they inhaled for 5 min over 5 days, followed by inhalation of 0.5 %HDM in the same protocol. Sensitization was confirmed by a skin test. Symptoms of allergic rhinitis were induced by intranasal application of 15 μl 0.5 %HDM and cough challenges with citric acid were performed. Airway resistance was measured in vivo by Pennock’s method. We found that both HDM and OVA-sensitized groups showed a significantly enhanced nasal reactivity and cough response compared with controls. The airway resistance data did not show significant differences. We conclude that the HDM cough model replicates functional aspects of the OVA model, which may make it an alternative to the latter. However, the superiority of the HDM model for experimental cough studies remains to be further explored.
Access provided by Autonomous University of Puebla. Download chapter PDF
Similar content being viewed by others
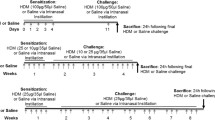
Keywords
1 Introduction
Animal models are extensively used in the biomedical research with the purpose to improve the understanding of disease mechanisms, to find early and effective diagnostic tools, and to develop novel treatment options. The rationale for using animal models is that they are likely to remain necessary until alternative models and systems, which would be equally sound and robust, are developed (Chow et al. 2008). The data obtained from animal models should be compared and translated to the conditions of human diseases.
Cough is the most important airway defensive mechanism and a symptom of the majority of respiratory diseases including asthma and COPD. Despite extensive animal research, there is still a lack of sufficient treatment for cough, regardless of its cause. The inability to translate and replicate the results from preclinical animal models to humans can stem not from the medication or the animal used, but from the model itself, which should mimic the natural pathogenesis of disease processes. Most preclinical studies of neural pathways involved in cough reflex and its pharmacological regulation have been conducted in guinea pigs, rats, rabbits, cats, or dogs (Belvisi and Bolser 2002). The literature shows that the most useful animal model to study cough is the conscious guinea pig.
Extensive work in this field clearly shows that the neurophysiology and neuropharmacology of the guinea pig vagus nerves are very similar to those of man. A great deal of research on the modulation of cough as a defensive mechanism and on the neural background involved in the cough regulatory circuits have been obtained from animal models using ovalbumin (OVA)-induced airway inflammation (Mokry et al. 2013; Sutovska et al. 2013; Hori et al. 2011; Brozmanova et al. 2008). Although the animals sensitized to OVA exhibit positive skin reactivity to the allergen and they develop early and late phase allergic responses after OVA exposure, accompanied by eosinophilia, the way of sensitization (intraperitoneal) and the antigen itself (chicken egg protein) are not as natural as human inhalation allergies.
The elucidation of mechanisms which are responsible for airway hypersensitivity can significantly increase the effectiveness of cough treatment and contribute to better quality of life of the affected patients. Effective therapy can simultaneously decrease the costs associated with diagnostics and more or less effective therapy. In the UK alone, there are around two million prescriptions issued by general practitioners for medications used for cough treatment every year, which translates into £1,314,000 (British Thoracic Society 2006). These costs are underestimated, as many cough treatment medications can be obtained over the counter.
The main objective of this study was to develop a model of house dust mite (HDM) antigens inhalation allergy in the guinea pig and to compare selected functional parameters with those that are usually measured in cough reflex studies employing OVA in the conscious guinea pig. The HDMs are complex antigens which sensitize the subjects via immunogenic epitopes, fecal pellets, lipopolysaccharides, beta-glucans, and chitin. They are used to study allergy and asthma (Yasue et al. 1998), but have never been used in the cough research field. We hypothesized that HDM-induced airway inflammation could also be an alternative model to study the cough response in the guinea pig.
2 Methods
2.1 General Notes
Animal care was provided and the experiments were conducted in agreement with the Animal Welfare Guidelines of the Comenius University and the statutes and rules of the Slovak Republic legislation. The study was approved by the Ethics Committee of Jessenius Faculty of Medicine (permit no. 2999/07-221). Male Dunking Hartley guinea pigs were used, obtained from an accredited breeding facility (L. Sobota, Městec Králové, Czech Republic). The animals were housed at a controlled room temperature of 21–22 °C, humidity of 60–70 %, 12-h light–dark cycle, and had free access to water and standard chow.
The animals were adapted twice to the laboratory conditions. To keep the stress to the minimum, they stayed in a plethysmography chamber to get familiarized with the environment and the laboratory personnel responsible for the experimental manipulations. In the chamber, the animals were exposed to an aerosol of buffered saline for 2 min, which corresponded to the future experimental procedure. Later on, the animals were challenged with 0.4 M citric acid and based on the response, they were divided into the control, OVA, and HDM groups, 10 animals each. All the groups involved an equal count of normo-, hypo-, and hyperreactors. The reason for this pattern of group assignment was that the cough response to citric acid in conscious animals shares extremely high variability, present even among healthy animals.
2.2 Sensitization
There were the following sensitization procedures.
-
1.
Sham-sensitized animals in the control group, injected with physiological saline – 1 ml;
-
2.
OVA-sensitized animals, injected with 10 μg of OVA, suspended in the aluminium hydroxide adjuvant, in 1 ml of saline; sensitization was confirmed by a skin prick test 21 days later on. All injections were intraperitoneal.
-
3.
HDM-sensitized animals. First line sensitization was achieved by inhalation of 0.25 % HDM aerosol (Greer Labs; Lenoir, NC) for 5 consecutive days for 5 min each in the plethysmography chamber. Second line (boosting) sensitization was achieved by inhalation of 0.5 % HDM aerosol for 5 consecutive days for 5 min each. Successful sensitization was confirmed 14 days later by a skin prick test (15 μl of 0.5 % HDM; intradermal application) and the animals were observed throughout the early and late phases of allergic reaction.
2.3 Assessment of Nasal Symptoms After Antigen Challenge
The intensity of symptoms was evaluated using a nasal symptom score system that matches symptoms’ intensity to numeric values (maximum 6 and minimum 0), according to the method of Brozmanova et al. (2006). The number of sneezes was tallied and the following symptoms were scored: (i) nasal discharge – lack 0, mild/moderate 1, dropping from the nostrils 2, (ii) eye/conjunctival reaction – lack 0, hazy eyes 1, visible lacrimation 2, and (iii) nasal crackles: lack 0, audible 1, audible from a distance 2. The main objective of this procedure was to confirm the ability of the allergen to induce upper airway response after a topical antigen challenge. To this end, the OVA group received 0.15 ml of 0.5 % OVA and the HDM group received 0.15 ml of 0.25 % HDM into both nostrils.
2.4 Citric Acid-Induced Cough
Conscious guinea pigs were individually placed in the body box (type 855, Hugo Sachs Electronik, March, Germany) consisting of head and body chambers. The opening between the two chambers was equipped with a plastic collar sealing the animal’s neck to prevent communication between the chambers. The appropriate collar size was chosen for each animal to prevent neck compression. The head chamber was connected to a nebulizer (Provokation Test I-Menzel; PARI GmbH, Starnberg, Germany), having the following specification: output 5 l/min and particle mass median aerodynamic diameter 1.2 μm. A suction device adjusted to the input of 5 l/min was connected to the head chamber to maintain constant airflow through the chamber during the aerosol administration. The airflow was measured with a Fleisch head connected to the head chamber. Data were recorded with the acquisition system ACQ Knowledge (Biopack, Santa Barbara, CA). Respiratory sounds, including sounds during coughing and sneezing, were recorded with a microphone placed in the roof of the head chamber and connected to a preamplifier and MP3 recorder. The pneumotachograph and microphone output were simultaneously recorded for off-line analysis.
The cough challenge was performed using an inhalation of 0.4 M citric acid for 10 min. Cough was defined as an expiratory airflow interrupting the basic respiratory pattern accompanied by a coughing sound. Coughs were analyzed by two trained persons; both being blind to the procedures carried out. Their results were compared and averaged if no statistically significant differences occurred.
To assess the cough threshold, the animals were challenged by gradually increasing concentrations of citric acid (0.05–1.60 M; 30 s inhalation separated by 1 min intervals) conducted at least 3 days apart. Parameters of C2 and C5 were obtained, corresponding to the concentrations of citric acid inducing 2 and more coughs or 5 or more coughs, respectively. The dose response curves also were constructed. Cough responses were measured after nasal allergen and saline challenges as based on the upper airway cough syndrome model validated by Brozmanova et al. (2006).
2.5 Measurement of Specific Airway Resistance
Specific airway resistance (Raw) was measured by a non-invasive plethysmographic method of Pennock et al. (1979). Conscious animals were placed in the body box as outlined above. Nasal airflow was measured in the head chamber and thoracic airflow was calculated from the pressure change in the lower chamber. Both measurements, made with differential pressure transducers (Simsoft; Martin, Slovakia), were fed into PC equipped with Pulmodyn Pennock W software for further analysis. The Raw is proportional to the phase difference between nasal and thoracic airflows and is based on the Lissajous loop representation of the two airflows on the X and Y axes. The Raw was measured after inhalation of histamine and methacholine aerosols.
3 Results
3.1 Skin Prick Tests
Skin tests were performed in all groups and early and late phase responses were observed on the skin after OVA and HDM intradermal injections, with saline and histamine pricks used as negative and positive controls, respectively (Fig. 1a). All OVA-sensitized animals exhibited the acute phase response with the presence of flare and edema, which developed within 15 min after antigen administration. The acute phase was observed up to 1 h. After 4–8 h, late phase was observed with the presence of flare (3–5 mm on average), edema, and induration, which was detected in 8/10 OVA-sensitized animals. In the HDM group, acute phase of the skin response was significantly weaker; redness with edema developed only in 4/10 animals. The late phase response, however, was considerably stronger when compared with the OVA group as it was present in 10/10 animals. Intensive scratching was observed in all animals a day after sensitization and scratch injuries and scabs were observable (Fig. 1b).
3.2 Nasal Symptoms
Animals in all groups were challenged by intranasal saline, OVA, or HDM. Control (saline) challenge induced no considerable symptoms, animals sneezed once or twice immediately after or during the insertion of a catheter. The animals of OVA and HDM groups were sneezing significantly more during the whole observation time of 1 h: 2 ± 1 vs. 12 ± 4 vs. 10 ± 2, p < 0.05, in the control, OVA, and HDM groups, respectively. In the last two groups, there also were present nasal discharge and nasal acoustic phenomenon typical for breathing through obstructed nasal passages. The OVA, but no HDM group, developed a conjunctival reaction. A total symptom score was comparable in these two groups: 5 ± 1 vs. 4 ± 1 points, which was significantly higher from the lack of symptoms in the control group (p < 0.05) (Fig. 2).
3.3 Cough Response to Inhalation of Citric Acid
Cough response to citric acid, the most frequently used tussive agent, was analyzed during the acute phase of the allergic response induced by nasal challenges of saline, HDM, and OVA. It is generally known that an inflammatory process in the nose/sinuses considerably upregulates the cough response. A total cough count obtained during 10-min lasting inhalation of citric acid was 9 ± 2 in controls vs. 16 ± 3 in HDM and 15 ± 2 coughs in OVA groups. The cough response in both sensitized groups, OVA and HDM, was significantly higher than that in controls (p < 0.05). However, the OVA and HDM groups achieved very similar results for the total cough count. This pattern was present also for the cough latency, which is the time from the start of exposure to a tussigen to the onset of the first cough bout. In controls, the first cough appeared after 180 ± 8 s, while in the OVA and HDM groups it appeared after 86 ± 8 and 80 ± 10 s, respectively, after the start of citric acid exposure (Fig. 3).
3.4 Specific Airway Resistance In Vivo
No significant changes were observed in specific airway resistance measured in vivo in response to histamine or methacholine, as compared with saline, in the HDM group (Table 1).
4 Discussion
The present study demonstrates that the house dust mite antigens can be used to model airway hypersensitivity/hyperreactivity in the guinea pig, with the possible application of this model in studies on cough reflex. Sensitization to HDM was confirmed indirectly by positive skin tests after intradermal administration of HDM, and also by the onset of nasal symptoms after topical intranasal HDM challenges in sensitized animals. We were able to reproduce functional changes, especially the upregulation of cough reflex in HDM-sensitized guinea pigs after topical administration of the allergen. Some of the data were comparable to the functional changes observed in the OVA-sensitized animals. However, we failed to show a significantly modified cough reflex sensitivity, which is well known for the OVA model (Brozmanova et al. 2006).
An animal model suitable to study cough in conscious animals, along with the effectiveness of a medication, should be characterized by an enhanced cough response to tussive stimuli when compared with control subjects, which reflects the upregulation of cough. This is achieved by the mechanisms of either peripheral or central neuroplasticity. The effectiveness of an antitussive medication should be studied on the background of upregulated cough response, which enables to notice a reduction of coughing. Further, such a model should be characterized by increased permeability of airway mucosa to tussive stimuli, which allows using lower concentrations of tussive aerosols. This strategy mimics the situation in humans, especially when the cough response is triggered by subthreshold stimuli. Increased permeability of airway mucosa is typical for airway inflammation. The animals used to model cough responses should be in the state of consciousness to mimic the cough reflex in humans that cannot be studied in anesthetized subjects, when the essential contribution of the suprapontine neural mechanisms is missing.
Another important issue is to limit bronchoconstrictive response, which is a usual accompaniment of different models of airway hyperreactivity. Bronchoconstriction influences breathing pattern of an animal and a total amount of inhaled tussigen and its deposition in airways. Therefore, none or mild bronchoconstriction would benefit a cough study, whereas models in which animals respond to airway challenge with wheezing and breathing difficulties are not suitable for this purpose. In the present study, we used the 0.25 % and later 0.50 % HDM antigen concentrations to sensitize the guinea pig. These concentrations are lower than those used in the mouse asthma models where they are in a range of 10 mg HDM extract per ml (Yasue et al. 1998).
In modeling, it is important to consider what the expected outcomes would be. Intraperitoneal sensitization, followed by intranasal and aerosol challenges, is relatively easy to conduct, induces strong Th2 responses, and it is more suitable from the immunological standpoint. On the other side, repeated aerosol challenges as a way of sensitization are more suitable to develop airway hyperreactivity and airway remodeling. Therefore, we set out to use the aerosol HDM challenges to develop our model. The downside of the HDM use is that it represents increased risk for the development of occupational allergy or asthma in laboratory personnel (Ruoppi et al. 2005), whereas this risk is virtually null when using intraperitoneal sensitization.
The question arises of whether the HDM as a whole extract or Der p 1 protein alone should be employed for sensitization? The Der p 1 allergen is the most immunogenic house mite protein of the extract, but not the only one. Accordingly, we found the sensitization to be far less effective with Der p 1 alone. Also, no one is exposed to Der p 1 alone. Therefore, unless one desires to study the allergenic capacity of just this allergen or to work on a specific desensitization, the whole HDM extract is more advisable (Hammad et al. 2010).
Cough is the most important defensive reflex of airways and it remains the most common symptom of airway diseases. Nonetheless, extensive research in the cough field has by far failed to bring satisfactory results which could possibly be translated into clinical applications in chronic cough. Neural circuits responsible for the mediation and modulation of cough reflex are studied in both anesthetized and conscious animals using either naïve animals or animals with airway inflammation. These models use different approaches to induce inflammation and thus modulate neurophysiological properties of cough mediating fibres in airways. This process, known as peripheral cough plasticity, is believed to be responsible for upregulation of cough reflex and troublesome persistent coughing (Poliacek et al. 2009a,b).
The overview of the literature suggests that the most frequently used model is based on ovalbumin sensitization with different patterns of secondary airway challenges (intranasal, inhalational) after the primary sensitization phase, which is usually intraperitoneal. Ovalbumin models of acute and chronic airway inflammation have been extensively reviewed by Kumar et al. (2008), with the listing of advantages and disadvantages regarding the clinical translation of findings as well as the description of limitations of OVA use. The OVA, however, is seldom implicated in humans, and other researchers have used alternative allergens that may have a greater clinical relevance, e.g., house dust mite or cockroach extracts (Nials and Uddin 2008).
The antigenic potential of HDM is complex, and this aggregate of allergens can induce an inflammatory response via multiple mechanisms compared with OVA. Proteases, fecal pellets, chitin, lipopolysaccharide, and others belong to the spectrum of pro-inflammatory signals released by the HDM. In general, currently used HDM models rely on topical administration of HDM into the airways (usually intranasally) over a course of weeks, which results in airway inflammation accompanied by eosinophilia, but there is not enough evidence to show the allergic nature of inflammation underscored by HDM-specific IgE antibodies, B-, or T-cells. Therefore, it is unclear whether the inflammation is truly allergic or just a consequence of repeated nasal insults.
Cough studies in vivo are usually based on the observation and analysis of the cough response to tussive challenges. Citric acid or capsaicin aerosols are used to induce reliable and reproducible cough responses to study either the mechanisms of cough or effectiveness of anti-cough medications. The HDM could play a role in such studies. It contains a number of proteolytic enzymes: cysteine proteases (group 1: Der p 1 and Der f 1) and serine proteases (groups 3, 6, and 9: Der p 3, Der p 6, Der p 9, Der f 3, Der f 6, and Der f 9); the enzymes are also present in HDM fecal pellets (Chapman et al. 2007). Collectively, they are responsible for 79 % of proteolytic activity of HDM (Stewart et al. 1994). Der p 1 and Der f 1 disrupt the intercellular tight junction proteins occludin and claudin-1 (Wan et al. 2001; Wan et al. 1999), which enables the delivery of an antigen to submucosal antigen-presenting cells. This impairment of the barrier function of the epithelium, present in poorly controlled asthmatic patients (Bhure et al. 2009), has not been replicated in a murine in vivo model after a single dose of HDM (Turi et al. 2011), which suggests that it results from chronic exposure to HDM rather than from an acute insult. To induce coughing, tussive aerosols must penetrate the airway mucosa to reach afferent nerve endings mediating cough. It is supposed that the HDM, with its protease activity, can destroy tight junction proteins connecting the airway epithelial cells, which may, in turn, increase mucosal permeability for different irritants, including tussive stimuli. Increased permeability of airway mucosa may facilitate cough induction with the use of lower concentrations of tussive substances, which possibly better emulates the natural conditions in which coughing appears.
In conclusion, HDM antigens appear to be a promising way to study the cough reflex. Further research is required to validate and optimize the HDM model of hypersensitive, cough producing airways.
References
Belvisi MG, Bolser DC (2002) Summary: animal models for cough. Pulm Pharmacol Ther 15(3):249–250
Bhure UN, Bhure SU, Bhatt BM, Mistry S, Pednekar SJ, Chari VV, Desai SA, Joshi JM, Paidhungat AJ (2009) Lung epithelial permeability and inhaled furosemide: added dimensions in asthmatics. Ann Nucl Med 23(6):549–557
British Thoracic Society (2006) Burden of lung disease – a statistics report from British Thoracic Society 2006, 2nd edn. Available from https://www.brit-thoracic.org.uk/document-library/delivery-of-respiratory-care/burden-of-lung-disease/burden-of-lung-disease-2006. Accessed 12 Aug 2015
Brozmanova M, Calkovsky V, Plevkova J, Bartos V, Plank L, Tatar M (2006) Early and late allergic phase related cough response in sensitized Guinea Pigs with experimental allergic rhinitis. Physiol Res 55(5):577–584
Brozmanova M, Plevkova J, Tatar M, Kollarik M (2008) Cough reflex sensitivity is increased in the guinea pig model of allergic rhinitis. J Physiol Pharmacol 59(Suppl 6):153–161
Chapman MD, Wünschmann S, Pomés A (2007) Proteases as Th2 adjuvants. Curr Allergy Asthma Rep 7(5):363–367
Chow PKH, Ng RTH, Ogden BE (2008) Using animal models in biomedical research: a primer for the investigator. World Scientific, Hackensack
Hammad H, Plantinga M, Deswarte K, Pouliot P, Willart MA, Kool M, Muskens F, Lambrecht BN (2010) Inflammatory dendritic cells – not basophils – are necessary and sufficient for induction of Th2 immunity to inhaled house dust mite allergen. J Exp Med 207(10):2097–2111
Hori A, Fujimura M, Ohkura N, Tokuda A (2011) Involvement of nitric oxide (NO) in cough reflex sensitivity between non-sensitized and OVA-sensitized guinea pigs. Cough 7(1):5
Kumar RK, Herbert C, Foster PS (2008) The “classical” ovalbumin challenge model of asthma in mice. Curr Drug Targets 9(6):485–494
Mokry J, Joskova M, Mokra D, Christensen I, Nosalova G (2013) Effects of selective inhibition of PDE4 and PDE7 on airway reactivity and cough in healthy and ovalbumin-sensitized guinea pigs. Adv Exp Med Biol 756:57–64
Nials AT, Uddin S (2008) Mouse models of allergic asthma: acute and chronic allergen challenge. Dis Model Mech 1(4–5):213–220
Pennock BE, Cox CP, Rogers RM, Cain WA, Wells JH (1979) A noninvasive technique for measurement of changes in specific airway resistance. J Appl Physiol Respir Environ Exerc Physiol 46(2):399–406
Poliacek I, Jakus J, Simera M, Barani H, Visnovcova N, Halasova E, Tomori Z (2009a) Excitability and rhythmicity of tracheobronchial cough is altered by aspiration reflex in cats. J Physiol Pharmacol 60(Suppl 5):105–110
Poliacek I, Tomori Z, Simera M, Barani H, Visnovcova N, Halasova E, Donic V, Jakus J (2009b) Provocation of aspiration reflexes and their effects on the pattern of cough and reflex apnea in cats. J Physiol Pharmacol 60(Suppl 5):99–104
Ruoppi P, Koistinen T, Pennanen S (2005) Sensitisation to mites in laboratory animal workers with rhinitis. Occup Environ Med 62(9):612–615
Stewart GA, Boyd SM, Bird CH, Krska KD, Kollinger MR, Thompson PJ (1994) Immunobiology of the serine protease allergens from house dust mites. Am J Ind Med 25(1):105–107
Sutovska M, Adamkov M, Kocmalova M, Mesarosova L, Oravec M, Franova S (2013) CRAC ion channels and airway defense reflexes in experimental allergic inflammation. Adv Exp Med Biol 756:39–48
Turi GJ, Ellis R, Wattie JN, Labiris NR, Inman MD (2011) The effects of inhaled house dust mite on airway barrier function and sensitivity to inhaled methacholine in mice. Am J Physiol Lung Cell Mol Physiol 300(2):L185–L190
Wan H, Winton HL, Soeller C, Tovey ER, Gruenert DC, Thompson PJ, Stewart GA, Taylor GW, Garrod DR, Cannell MB, Robinson C (1999) Der p 1 facilitates transepithelial allergen delivery by disruption of tight junctions. J Clin Invest 104(1):123–133
Wan H, Winton HL, Soeller C, Taylor GW, Gruenert DC, Thompson PJ, Cannell MB, Stewart GA, Garrod DR, Robinson C (2001) The transmembrane protein occludin of epithelial tight junctions is a functional target for serine peptidases from faecal pellets of Dermatophagoides pteronyssinus. Clin Exp Allergy 31(2):279–294
Yasue M, Yokota T, Suko M, Okudaira H, Okumura Y (1998) Comparison of sensitization to crude and purified house dust mite allergens in inbred mice. Lab Anim Sci 48(4):346–352
Acknowledgements
This study was supported by VEGA No. 1/0107/2014 and Biomed ITMS: 26220220187
Conflicts of Interest
The authors declare no conflicts of interest in relation to this article.
Author information
Authors and Affiliations
Corresponding author
Editor information
Editors and Affiliations
Rights and permissions
Copyright information
© 2016 Springer International Publishing Switzerland
About this chapter
Cite this chapter
Buday, T., Gavliakova, S., Mokry, J., Medvedova, I., Kavalcikova-Bogdanova, N., Plevkova, J. (2016). The Guinea Pig Sensitized by House Dust Mite: A Model of Experimental Cough Studies. In: Pokorski, M. (eds) Respiratory Contagion. Advances in Experimental Medicine and Biology(), vol 905. Springer, Cham. https://doi.org/10.1007/5584_2016_217
Download citation
DOI: https://doi.org/10.1007/5584_2016_217
Published:
Publisher Name: Springer, Cham
Print ISBN: 978-3-319-30603-2
Online ISBN: 978-3-319-30604-9
eBook Packages: Biomedical and Life SciencesBiomedical and Life Sciences (R0)